Abstract
Both the wild type and an isogenic hydrogenase-negative mutant of Azorhizobium caulinodans growing ex planta on N2 as the N source were studied in succinate-limited steady-state chemostat cultures under 0.2 to 3.0% dissolved O2 tension. Production or consumption of O2, H2, and CO2 was measured with an on-line-connected mass spectrometer. In the range of 0.2 to 3.0%, growth of both the wild type and the mutant was equally dependent on the dissolved O2 tension: the growth yield decreased, and the specific O2 consumption and CO2 production increased. A similar dependency on the dissolved O2 tension was found for the mutant with 2.5% H2 in the influent gas. The H2/N2 ratio (moles of H2 evolved per mole of N2 consumed via nitrogenase) of the mutant, growing with or without 2.5% H2, increased with increasing dissolved O2 tensions. This increase in the H2/N2 ratio was small but significant. The dependencies of the ATP/N2 ratio (moles of ATP consumed per mole of N2 fixed) and the ATP/2e- ratio [moles of ATP consumed per mole of electron pairs transferred from NAD(P)H to nitrogenase] on the dissolved O2 tension were estimated. These dependencies were interpreted in terms of the physiological concepts of respiratory protection and autoprotection.
Full text
PDF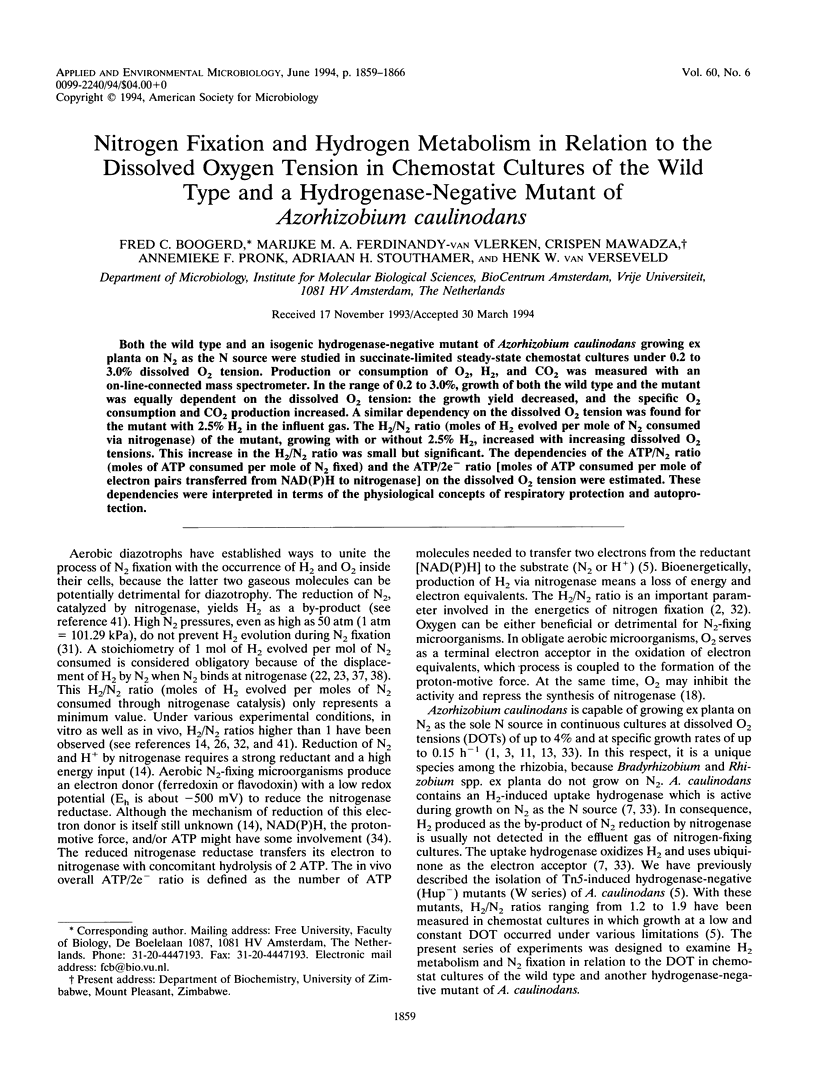
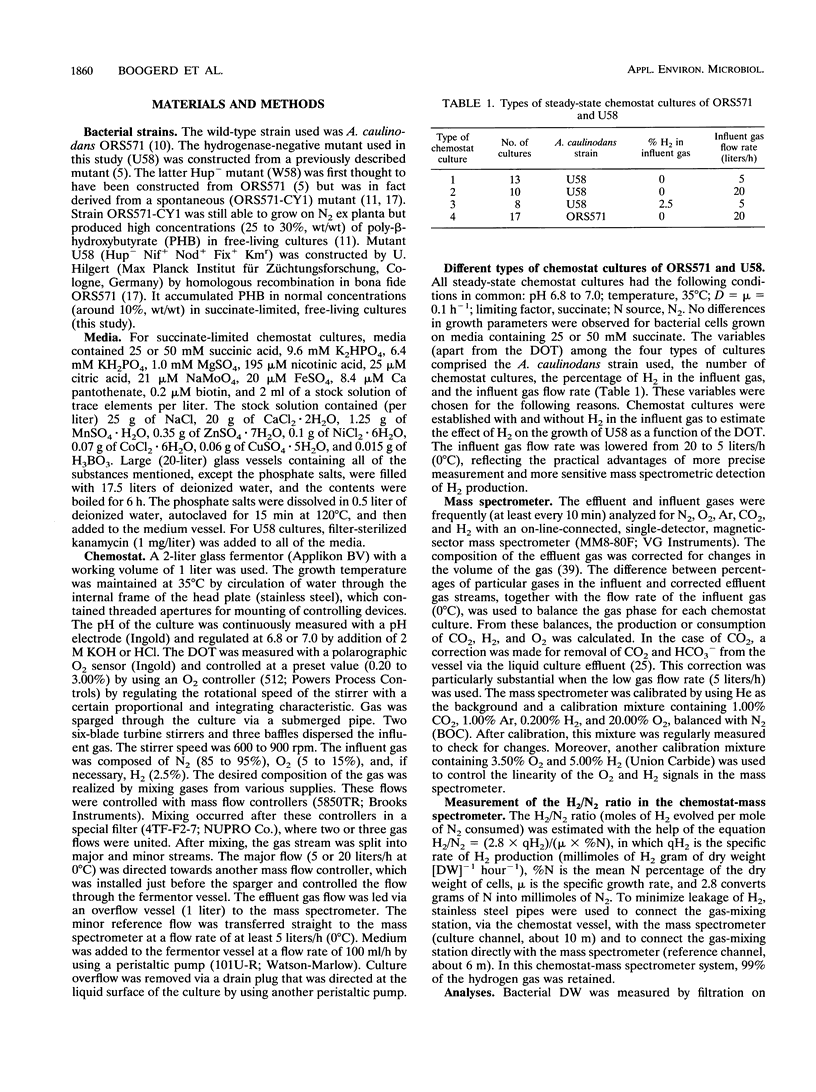
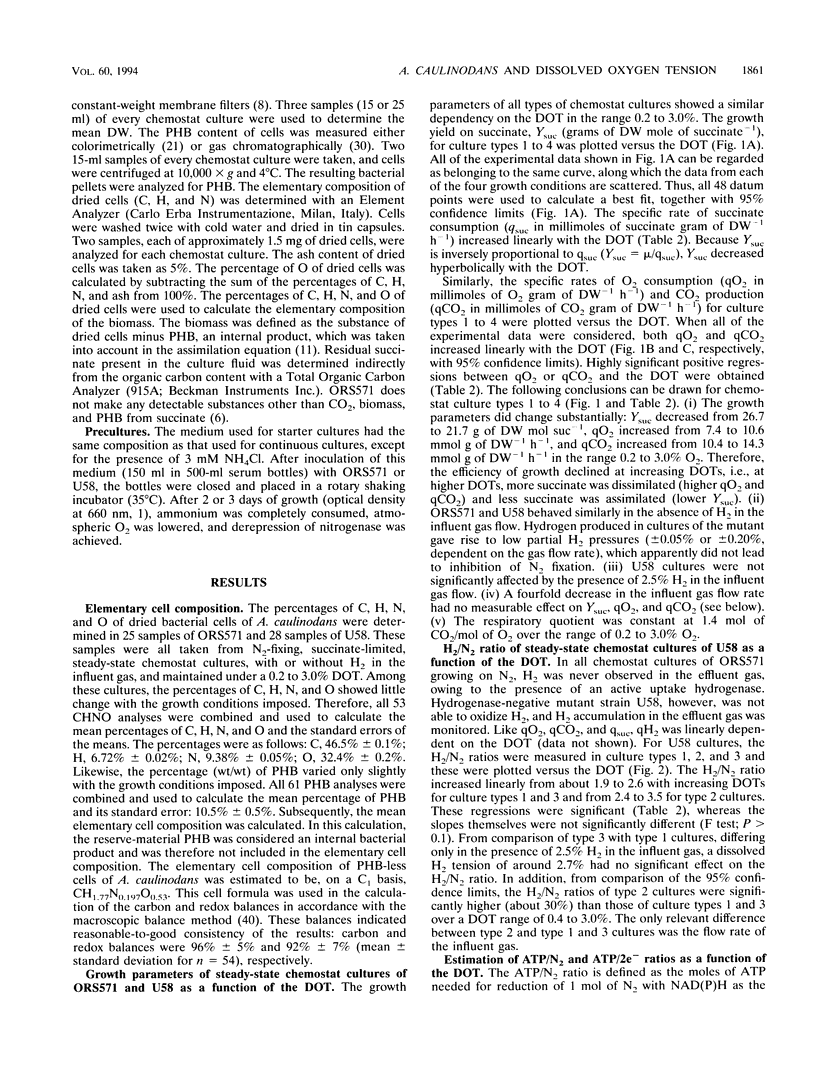
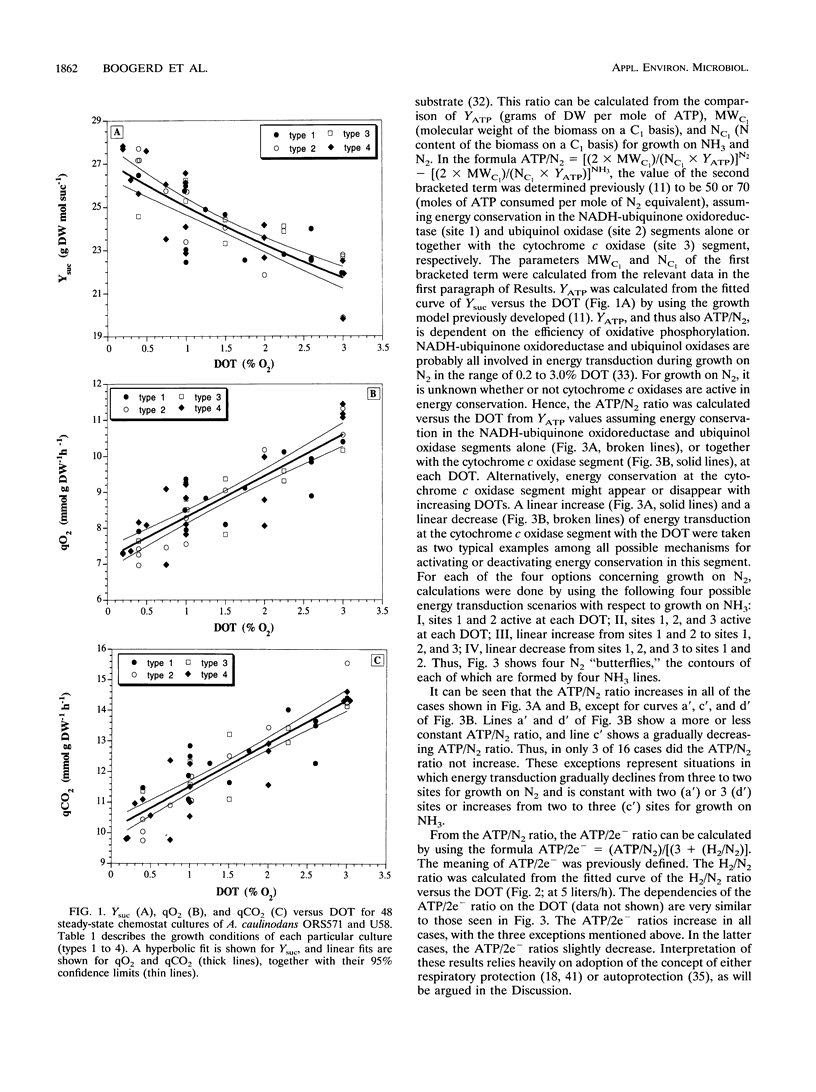
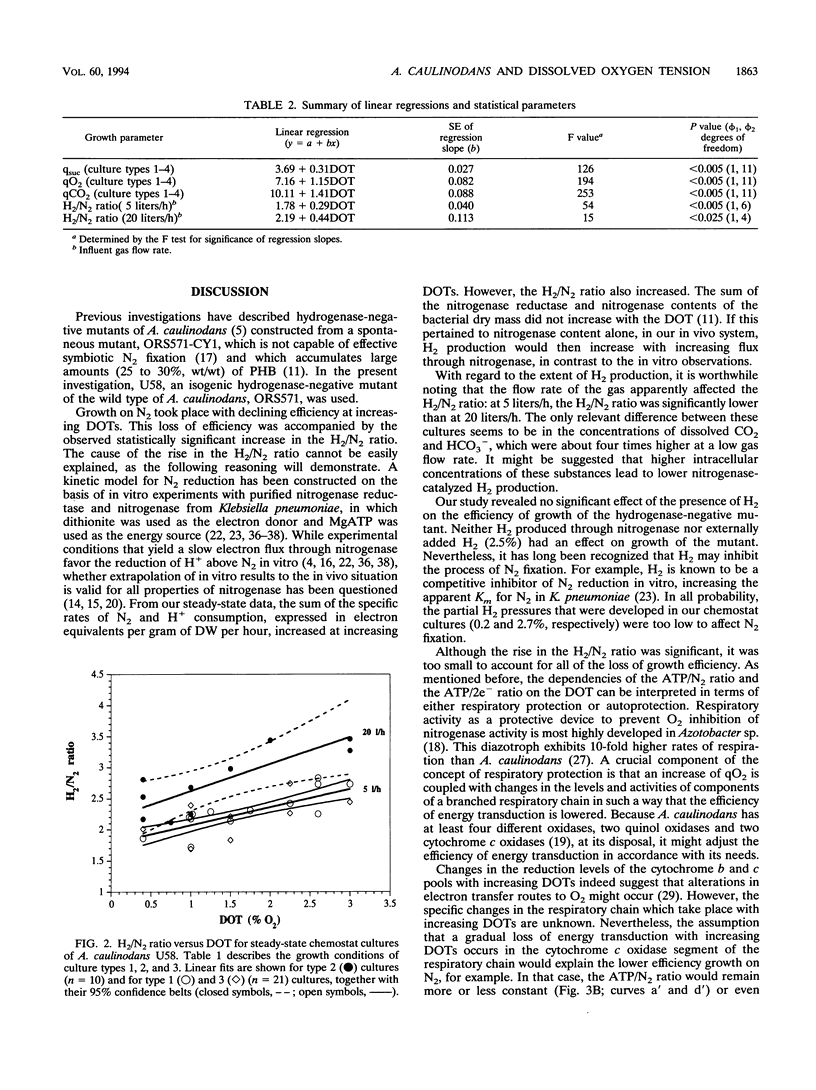
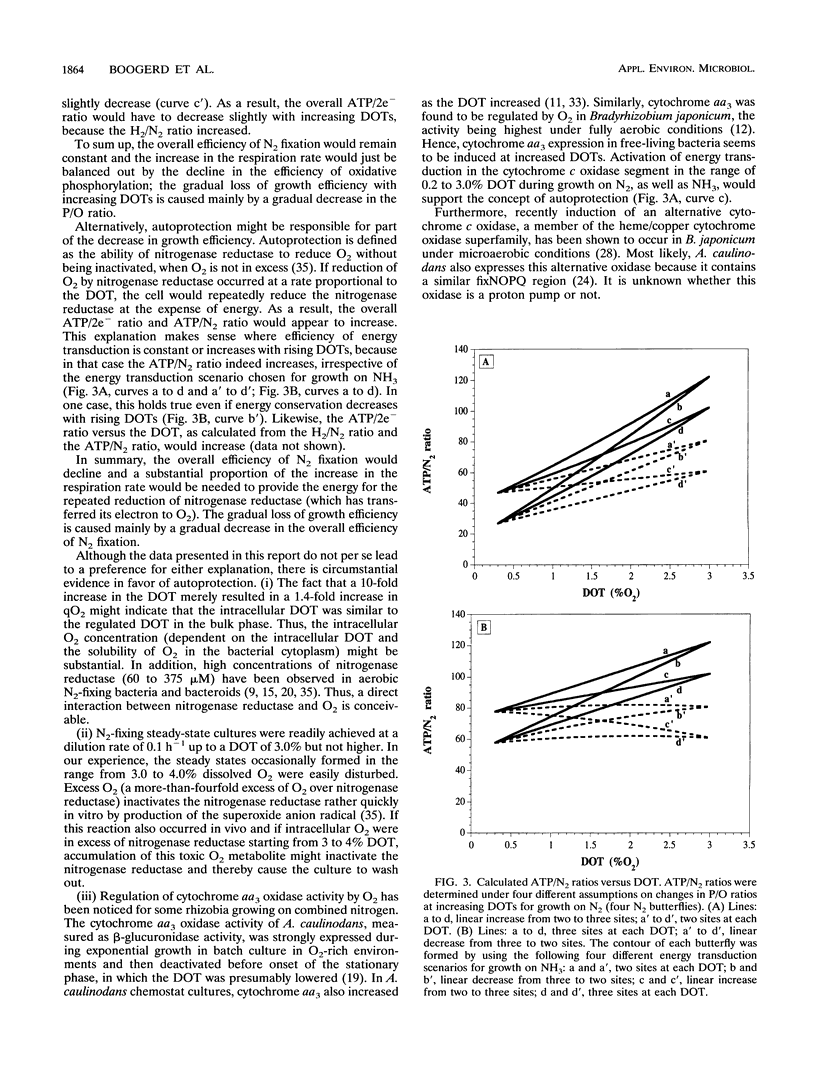
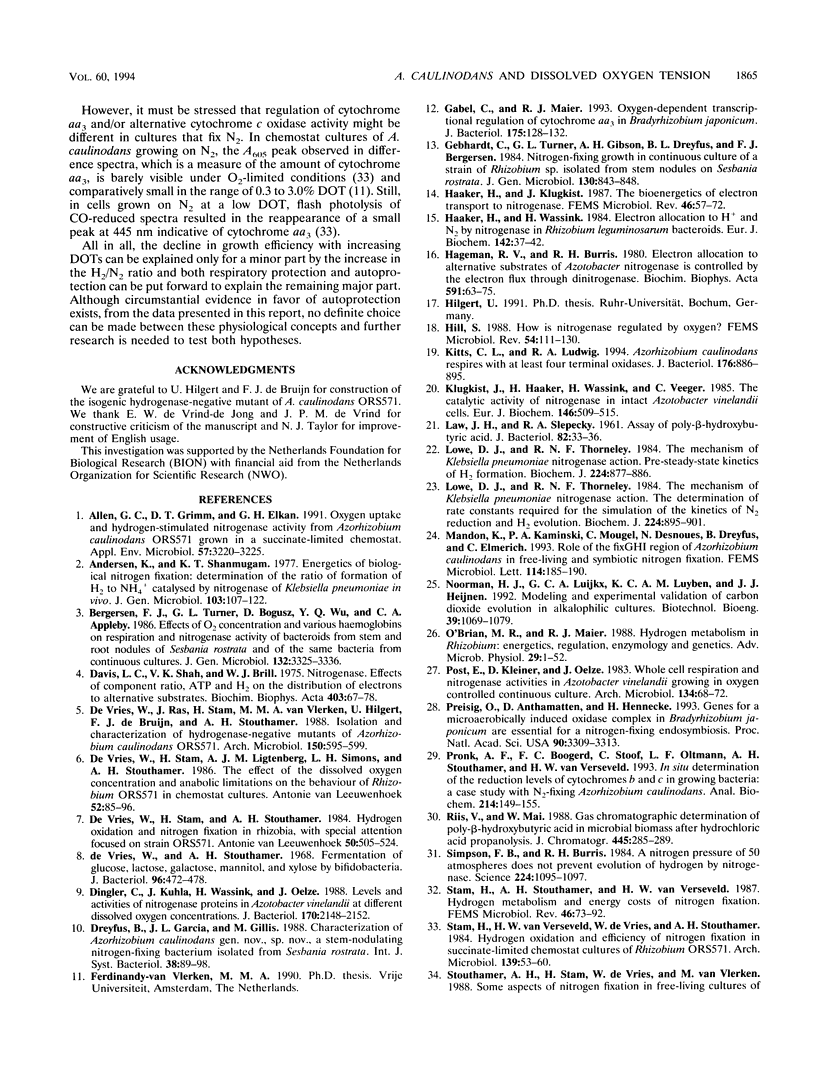
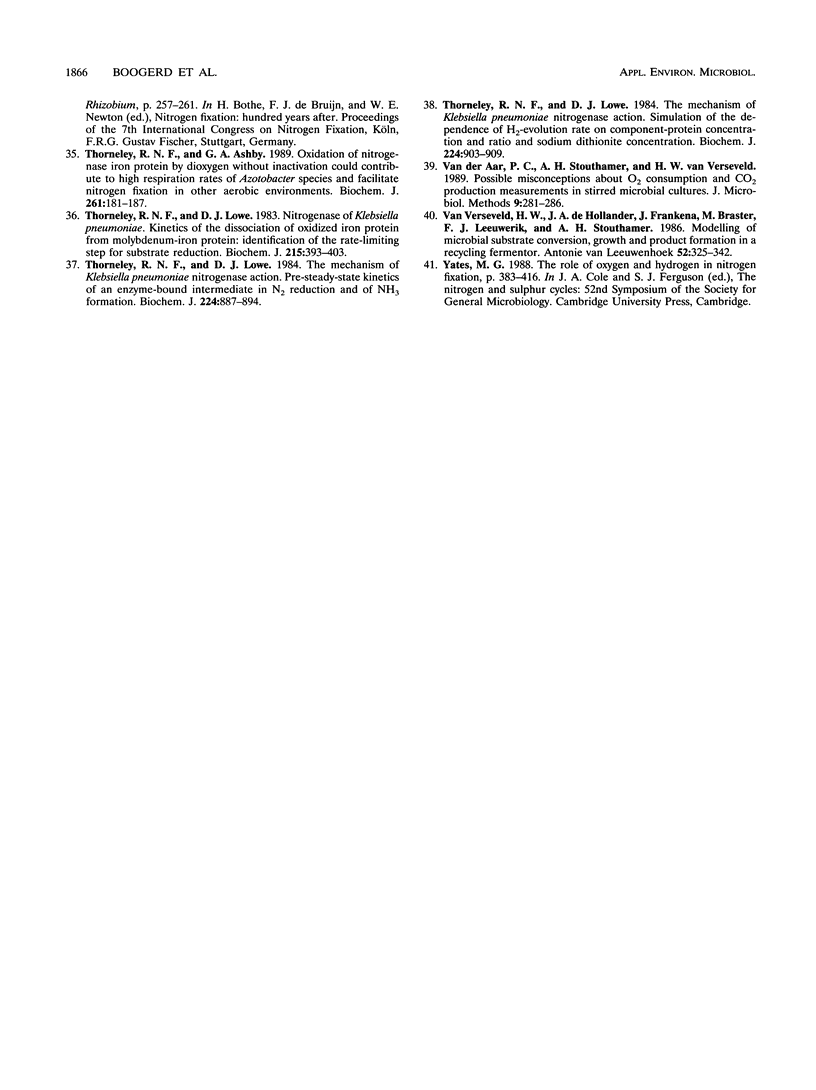
Selected References
These references are in PubMed. This may not be the complete list of references from this article.
- Allen G. C., Grimm D. T., Elkan G. H. Oxygen Uptake and Hydrogen-Stimulated Nitrogenase Activity from Azorhizobium caulinodans ORS571 Grown in a Succinate-Limited Chemostat. Appl Environ Microbiol. 1991 Nov;57(11):3220–3225. doi: 10.1128/aem.57.11.3220-3225.1991. [DOI] [PMC free article] [PubMed] [Google Scholar]
- Andersen K., Shanmugam K. T. Energetics of biological nitrogen fixation: determination of the ratio of formation of H2 to NH4+ catalysed by nitrogenase of Klebsiella pneumoniae in vivo. J Gen Microbiol. 1977 Nov;103(1):107–122. doi: 10.1099/00221287-103-1-107. [DOI] [PubMed] [Google Scholar]
- Davis L. C., Shah V. K., Brill W. J. Nitrogenase. VII. Effect of component ratio, ATP and H2 on the distribution of electrons to alternative substrates. Biochim Biophys Acta. 1975 Sep 22;403(1):67–78. doi: 10.1016/0005-2744(75)90009-1. [DOI] [PubMed] [Google Scholar]
- Dingler C., Kuhla J., Wassink H., Oelze J. Levels and activities of nitrogenase proteins in Azotobacter vinelandii grown at different dissolved oxygen concentrations. J Bacteriol. 1988 May;170(5):2148–2152. doi: 10.1128/jb.170.5.2148-2152.1988. [DOI] [PMC free article] [PubMed] [Google Scholar]
- Gabel C., Maier R. J. Oxygen-dependent transcriptional regulation of cytochrome aa3 in Bradyrhizobium japonicum. J Bacteriol. 1993 Jan;175(1):128–132. doi: 10.1128/jb.175.1.128-132.1993. [DOI] [PMC free article] [PubMed] [Google Scholar]
- Haaker H., Wassink H. Electron allocation to H+ and N2 by nitrogenase in Rhizobium leguminosarum bacteroids. Eur J Biochem. 1984 Jul 2;142(1):37–42. doi: 10.1111/j.1432-1033.1984.tb08247.x. [DOI] [PubMed] [Google Scholar]
- Hageman R. V., Burris R. H. Electron allocation to alternative substrates of Azotobacter nitrogenase is controlled by the electron flux through dinitrogenase. Biochim Biophys Acta. 1980 Jun 10;591(1):63–75. doi: 10.1016/0005-2728(80)90220-0. [DOI] [PubMed] [Google Scholar]
- Hill S. How is nitrogenase regulated by oxygen? FEMS Microbiol Rev. 1988 Apr-Jun;4(2):111–129. doi: 10.1111/j.1574-6968.1988.tb02738.x. [DOI] [PubMed] [Google Scholar]
- Kitts C. L., Ludwig R. A. Azorhizobium caulinodans respires with at least four terminal oxidases. J Bacteriol. 1994 Feb;176(3):886–895. doi: 10.1128/jb.176.3.886-895.1994. [DOI] [PMC free article] [PubMed] [Google Scholar]
- Klugkist J., Haaker H., Wassink H., Veeger C. The catalytic activity of nitrogenase in intact Azotobacter vinelandii cells. Eur J Biochem. 1985 Feb 1;146(3):509–515. doi: 10.1111/j.1432-1033.1985.tb08681.x. [DOI] [PubMed] [Google Scholar]
- LAW J. H., SLEPECKY R. A. Assay of poly-beta-hydroxybutyric acid. J Bacteriol. 1961 Jul;82:33–36. doi: 10.1128/jb.82.1.33-36.1961. [DOI] [PMC free article] [PubMed] [Google Scholar]
- Lowe D. J., Thorneley R. N. The mechanism of Klebsiella pneumoniae nitrogenase action. Pre-steady-state kinetics of H2 formation. Biochem J. 1984 Dec 15;224(3):877–886. doi: 10.1042/bj2240877. [DOI] [PMC free article] [PubMed] [Google Scholar]
- Lowe D. J., Thorneley R. N. The mechanism of Klebsiella pneumoniae nitrogenase action. The determination of rate constants required for the simulation of the kinetics of N2 reduction and H2 evolution. Biochem J. 1984 Dec 15;224(3):895–901. doi: 10.1042/bj2240895. [DOI] [PMC free article] [PubMed] [Google Scholar]
- Mandon K., Kaminski P. A., Mougel C., Desnoues N., Dreyfus B., Elmerich C. Role of the fixGHI region of Azorhizobium caulinodans in free-living and symbiotic nitrogen fixation. FEMS Microbiol Lett. 1993 Dec 1;114(2):185–189. doi: 10.1111/j.1574-6968.1993.tb06571.x. [DOI] [PubMed] [Google Scholar]
- O'Brian M. R., Maier R. J. Hydrogen metabolism in Rhizobium: energetics, regulation, enzymology and genetics. Adv Microb Physiol. 1988;29:1–52. doi: 10.1016/s0065-2911(08)60345-8. [DOI] [PubMed] [Google Scholar]
- Post E., Kleiner D., Oelze J. Whole cell respiration and nitrogenase activities in Azotobacter vinelandii growing in oxygen controlled continuous culture. Arch Microbiol. 1983 Jan;134(1):68–72. doi: 10.1007/BF00429410. [DOI] [PubMed] [Google Scholar]
- Preisig O., Anthamatten D., Hennecke H. Genes for a microaerobically induced oxidase complex in Bradyrhizobium japonicum are essential for a nitrogen-fixing endosymbiosis. Proc Natl Acad Sci U S A. 1993 Apr 15;90(8):3309–3313. doi: 10.1073/pnas.90.8.3309. [DOI] [PMC free article] [PubMed] [Google Scholar]
- Pronk A. F., Boogerd F. C., Stoof C., Oltmann L. F., Stouthamer A. H., van Verseveld H. W. In situ determination of the reduction levels of cytochromes b and c in growing bacteria: a case study with N2-fixing Azorhizobium caulinodans. Anal Biochem. 1993 Oct;214(1):149–155. doi: 10.1006/abio.1993.1470. [DOI] [PubMed] [Google Scholar]
- Simpson F. B., Burris R. H. A nitrogen pressure of 50 atmospheres does not prevent evolution of hydrogen by nitrogenase. Science. 1984 Jun 8;224(4653):1095–1097. doi: 10.1126/science.6585956. [DOI] [PubMed] [Google Scholar]
- Thorneley R. N., Ashby G. A. Oxidation of nitrogenase iron protein by dioxygen without inactivation could contribute to high respiration rates of Azotobacter species and facilitate nitrogen fixation in other aerobic environments. Biochem J. 1989 Jul 1;261(1):181–187. doi: 10.1042/bj2610181. [DOI] [PMC free article] [PubMed] [Google Scholar]
- Thorneley R. N., Lowe D. J. Nitrogenase of Klebsiella pneumoniae. Kinetics of the dissociation of oxidized iron protein from molybdenum-iron protein: identification of the rate-limiting step for substrate reduction. Biochem J. 1983 Nov 1;215(2):393–403. doi: 10.1042/bj2150393. [DOI] [PMC free article] [PubMed] [Google Scholar]
- Thorneley R. N., Lowe D. J. The mechanism of Klebsiella pneumoniae nitrogenase action. Pre-steady-state kinetics of an enzyme-bound intermediate in N2 reduction and of NH3 formation. Biochem J. 1984 Dec 15;224(3):887–894. doi: 10.1042/bj2240887. [DOI] [PMC free article] [PubMed] [Google Scholar]
- Thorneley R. N., Lowe D. J. The mechanism of Klebsiella pneumoniae nitrogenase action. Simulation of the dependences of H2-evolution rate on component-protein concentration and ratio and sodium dithionite concentration. Biochem J. 1984 Dec 15;224(3):903–909. doi: 10.1042/bj2240903. [DOI] [PMC free article] [PubMed] [Google Scholar]
- de Vries W., Stam H., Duys J. G., Ligtenberg A. J., Simons L. H., Stouthamer A. H. The effect of the dissolved oxygen concentration and anabolic limitations on the behaviour of Rhizobium ORS571 in chemostat cultures. Antonie Van Leeuwenhoek. 1986;52(1):85–96. doi: 10.1007/BF00402690. [DOI] [PubMed] [Google Scholar]
- de Vries W., Stam H., Stouthamer A. H. Hydrogen oxidation and nitrogen fixation in rhizobia, with special attention focused on strain ORS 571. Antonie Van Leeuwenhoek. 1984;50(5-6):505–524. doi: 10.1007/BF02386223. [DOI] [PubMed] [Google Scholar]
- de Vries W., Stouthamer A. H. Fermentation of glucose, lactose, galactose, mannitol, and xylose by bifidobacteria. J Bacteriol. 1968 Aug;96(2):472–478. doi: 10.1128/jb.96.2.472-478.1968. [DOI] [PMC free article] [PubMed] [Google Scholar]
- van Verseveld H. W., de Hollander J. A., Frankena J., Braster M., Leeuwerik F. J., Stouthamer A. H. Modeling of microbial substrate conversion, growth and product formation in a recycling fermentor. Antonie Van Leeuwenhoek. 1986;52(4):325–342. doi: 10.1007/BF00428644. [DOI] [PubMed] [Google Scholar]