Abstract
Glucose-excess cultures of Streptococcus bovis consumed glucose faster than the amount that could be explained by growth or maintenance, and nongrowing chloramphenicol-treated cells had a rate of glucose consumption that was 10-fold greater than the maintenance rate. Because N,N-dicyclohexylcarbodiimide, an inhibitor of the membrane-bound F1F0 ATPase, eliminated the nongrowth energy dissipation (energy spilling) without a decrease in ATP and the rate of energy spilling could be increased by the protonophore 3,3',4',5-tetrachlorosalicylanilide, it appeared that a futile cycle of protons through the cell membrane was responsible for most of the energy spilling. When the rate of energy spilling was decreased gradually with iodoacetate, there was only a small decrease in the phosphorylation potential (delta G'p) and the theoretical estimate of H+ per ATP decreased from 4.2 to 3.6. On the bases of this ratio of H+ to ATP and the rate of ATP production, the flux of protons (amperage) across the cell membrane was directly proportional to the rate of energy spilling. Amperage values estimated from delta G'p were, however, nearly twice as great as values which were estimated from the heat production (delta H) of the cells [amperage = (0.38 x wattage)/delta p]. The last comparison indicated that only a fraction of the delta G of ATP hydrolysis was harvested by the F1F0 ATPase to pump protons. Both estimates of amperage indicated that the resistance of the cell membrane to proton conductance was inversely proportional to the log of the energy-spilling rate.
Full text
PDF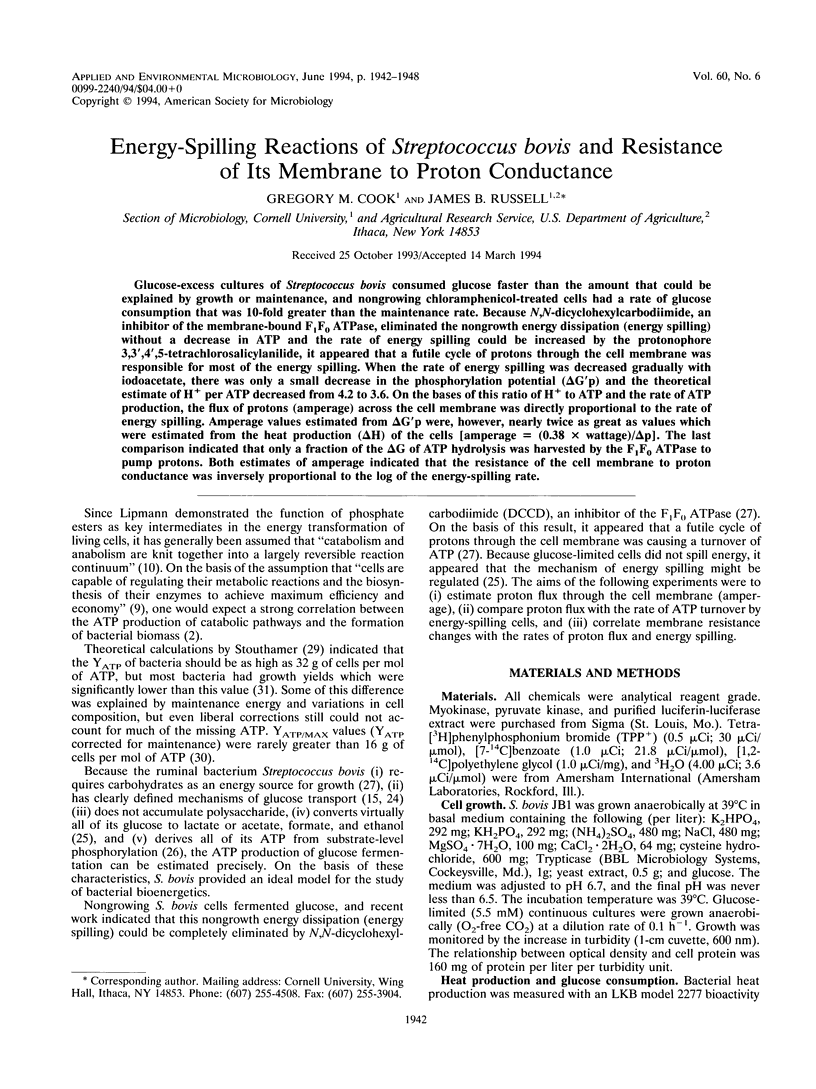
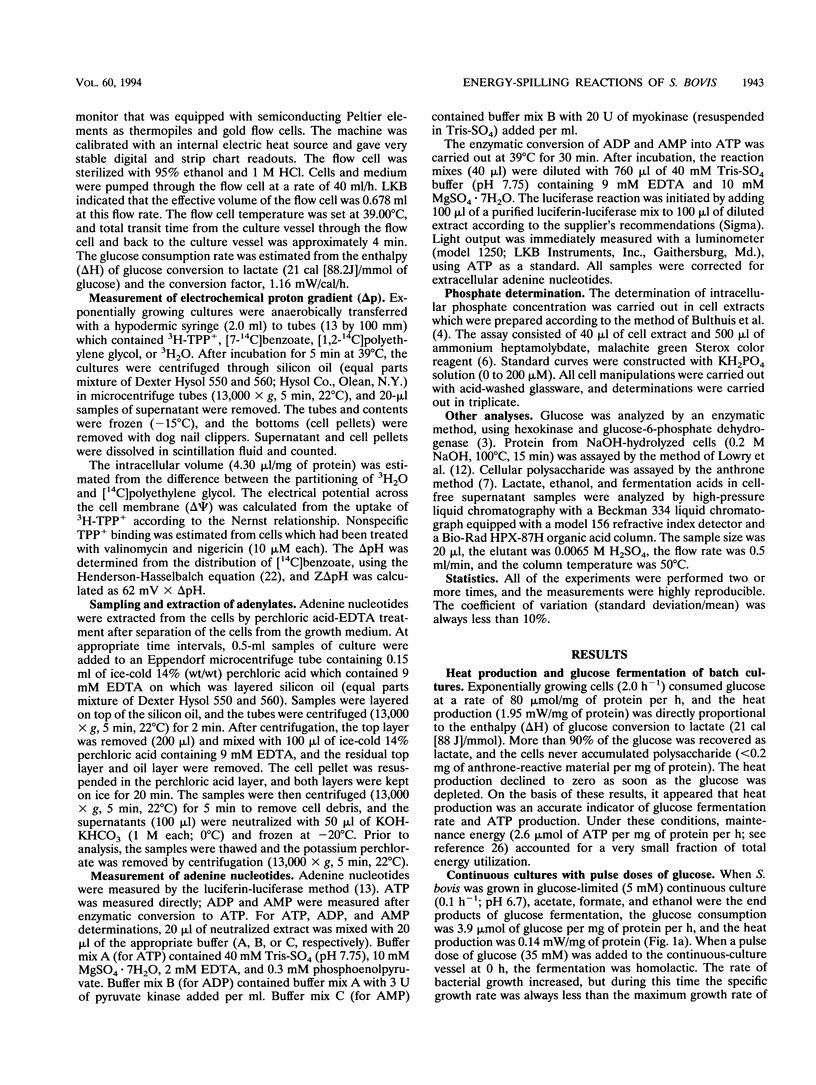
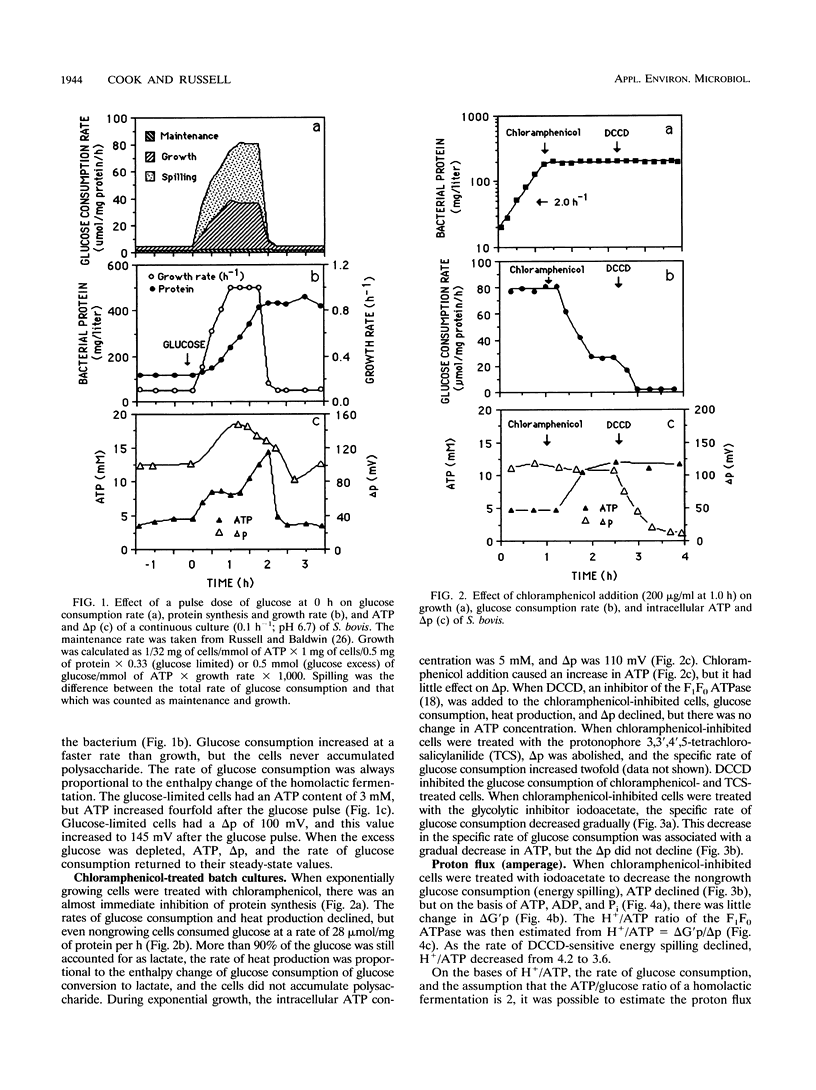
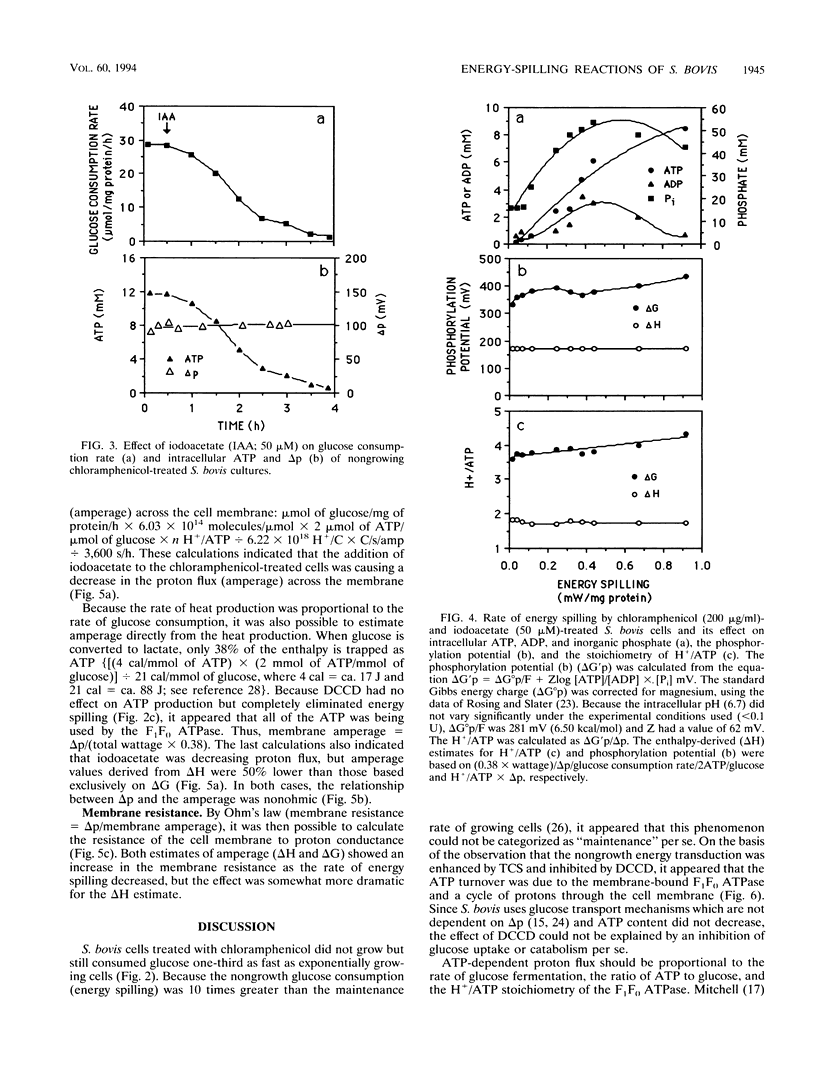
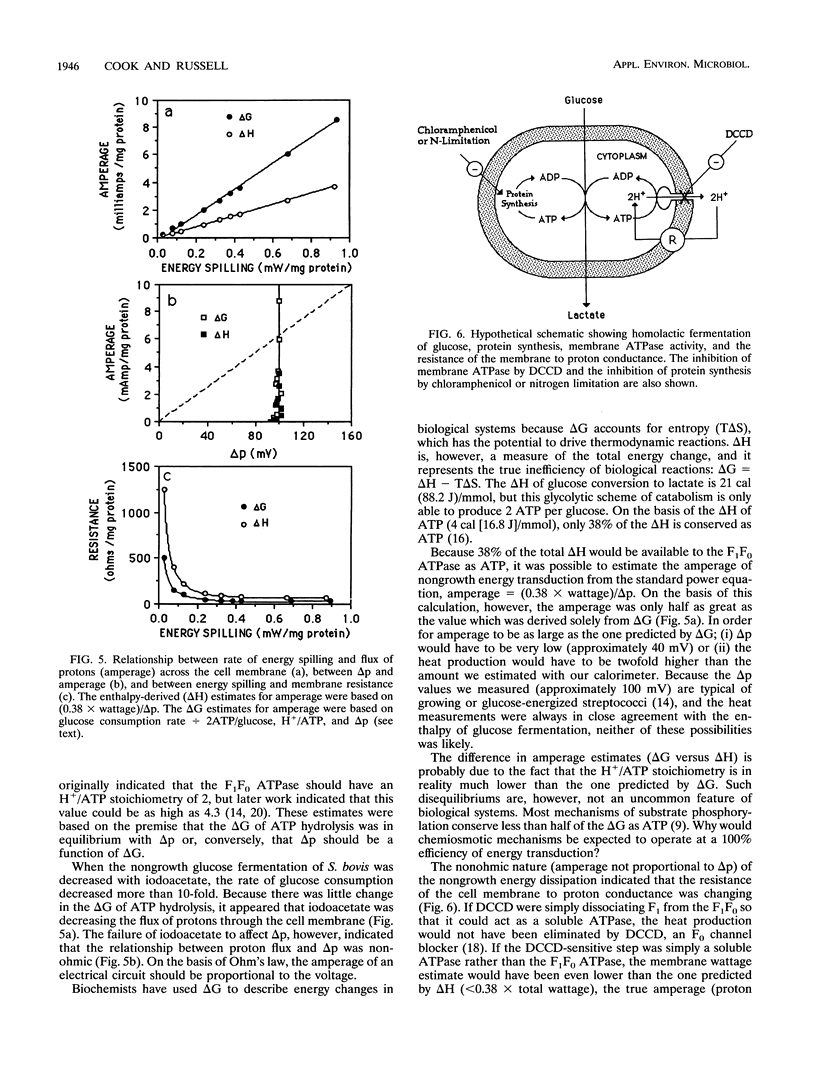
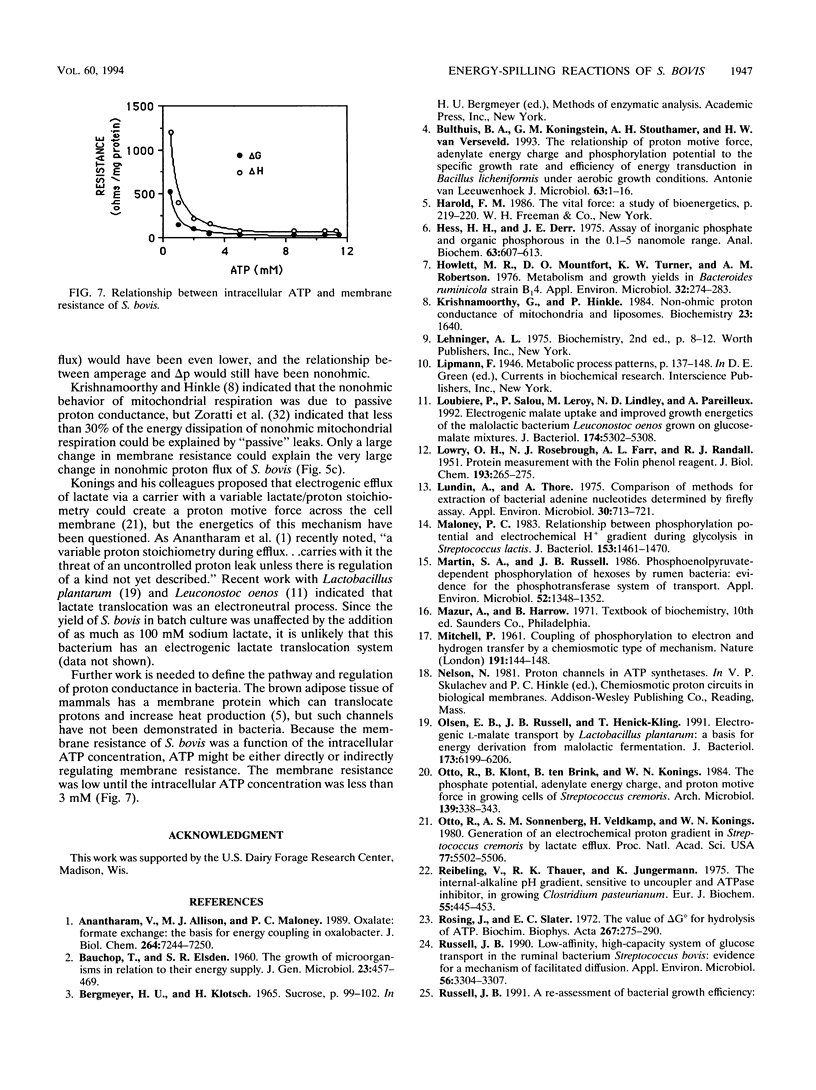
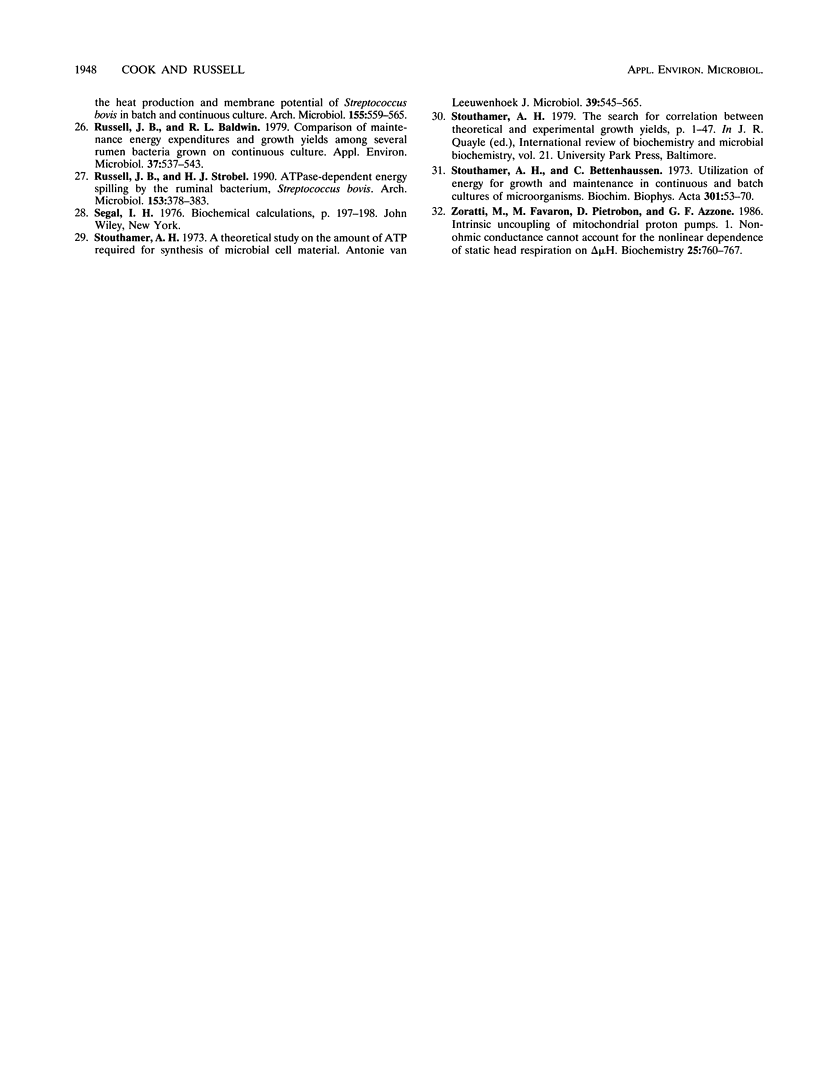
Selected References
These references are in PubMed. This may not be the complete list of references from this article.
- Anantharam V., Allison M. J., Maloney P. C. Oxalate:formate exchange. The basis for energy coupling in Oxalobacter. J Biol Chem. 1989 May 5;264(13):7244–7250. [PubMed] [Google Scholar]
- BAUCHOP T., ELSDEN S. R. The growth of micro-organisms in relation to their energy supply. J Gen Microbiol. 1960 Dec;23:457–469. doi: 10.1099/00221287-23-3-457. [DOI] [PubMed] [Google Scholar]
- Bulthuis B. A., Koningstein G. M., Stouthamer A. H., van Verseveld H. W. The relation of proton motive force, adenylate energy charge and phosphorylation potential to the specific growth rate and efficiency of energy transduction in Bacillus licheniformis under aerobic growth conditions. Antonie Van Leeuwenhoek. 1993 Jan;63(1):1–16. doi: 10.1007/BF00871725. [DOI] [PubMed] [Google Scholar]
- Hess H. H., Derr J. E. Assay of inorganic and organic phosphorus in the 0.1-5 nanomole range. Anal Biochem. 1975 Feb;63(2):607–613. doi: 10.1016/0003-2697(75)90388-7. [DOI] [PubMed] [Google Scholar]
- Howlett M. R., Mountfort D. O., Turner K. W., Roberton A. M. Metabolism and growth yields in Bacteroides ruminicola strain b14. Appl Environ Microbiol. 1976 Aug;32(2):274–283. doi: 10.1128/aem.32.2.274-283.1976. [DOI] [PMC free article] [PubMed] [Google Scholar]
- Krishnamoorthy G., Hinkle P. C. Non-ohmic proton conductance of mitochondria and liposomes. Biochemistry. 1984 Apr 10;23(8):1640–1645. doi: 10.1021/bi00303a009. [DOI] [PubMed] [Google Scholar]
- LOWRY O. H., ROSEBROUGH N. J., FARR A. L., RANDALL R. J. Protein measurement with the Folin phenol reagent. J Biol Chem. 1951 Nov;193(1):265–275. [PubMed] [Google Scholar]
- Loubiere P., Salou P., Leroy M. J., Lindley N. D., Pareilleux A. Electrogenic malate uptake and improved growth energetics of the malolactic bacterium Leuconostoc oenos grown on glucose-malate mixtures. J Bacteriol. 1992 Aug;174(16):5302–5308. doi: 10.1128/jb.174.16.5302-5308.1992. [DOI] [PMC free article] [PubMed] [Google Scholar]
- Lundin A., Thore A. Comparison of methods for extraction of bacterial adenine nucleotides determined by firefly assay. Appl Microbiol. 1975 Nov;30(5):713–721. doi: 10.1128/am.30.5.713-721.1975. [DOI] [PMC free article] [PubMed] [Google Scholar]
- MITCHELL P. Coupling of phosphorylation to electron and hydrogen transfer by a chemi-osmotic type of mechanism. Nature. 1961 Jul 8;191:144–148. doi: 10.1038/191144a0. [DOI] [PubMed] [Google Scholar]
- Maloney P. C. Relationship between phosphorylation potential and electrochemical H+ gradient during glycolysis in Streptococcus lactis. J Bacteriol. 1983 Mar;153(3):1461–1470. doi: 10.1128/jb.153.3.1461-1470.1983. [DOI] [PMC free article] [PubMed] [Google Scholar]
- Martin S. A., Russell J. B. Phosphoenolpyruvate-dependent phosphorylation of hexoses by ruminal bacteria: evidence for the phosphotransferase transport system. Appl Environ Microbiol. 1986 Dec;52(6):1348–1352. doi: 10.1128/aem.52.6.1348-1352.1986. [DOI] [PMC free article] [PubMed] [Google Scholar]
- Olsen E. B., Russell J. B., Henick-Kling T. Electrogenic L-malate transport by Lactobacillus plantarum: a basis for energy derivation from malolactic fermentation. J Bacteriol. 1991 Oct;173(19):6199–6206. doi: 10.1128/jb.173.19.6199-6206.1991. [DOI] [PMC free article] [PubMed] [Google Scholar]
- Otto R., Sonnenberg A. S., Veldkamp H., Konings W. N. Generation of an electrochemical proton gradient in Streptococcus cremoris by lactate efflux. Proc Natl Acad Sci U S A. 1980 Sep;77(9):5502–5506. doi: 10.1073/pnas.77.9.5502. [DOI] [PMC free article] [PubMed] [Google Scholar]
- Riebeling V., Thauer R. K., Jungermann K. The internal-alkaline pH gradient, sensitive to uncoupler and ATPase inhibitor, in growing Clostridium pasteurianum. Eur J Biochem. 1975 Jul 1;55(2):445–453. doi: 10.1111/j.1432-1033.1975.tb02181.x. [DOI] [PubMed] [Google Scholar]
- Rosing J., Slater E. C. The value of G degrees for the hydrolysis of ATP. Biochim Biophys Acta. 1972 May 25;267(2):275–290. doi: 10.1016/0005-2728(72)90116-8. [DOI] [PubMed] [Google Scholar]
- Russell J. B., Baldwin R. L. Comparison of maintenance energy expenditures and growth yields among several rumen bacteria grown on continuous culture. Appl Environ Microbiol. 1979 Mar;37(3):537–543. doi: 10.1128/aem.37.3.537-543.1979. [DOI] [PMC free article] [PubMed] [Google Scholar]
- Russell J. B. Low-affinity, high-capacity system of glucose transport in the ruminal bacterium Streptococcus bovis: evidence for a mechanism of facilitated diffusion. Appl Environ Microbiol. 1990 Nov;56(11):3304–3307. doi: 10.1128/aem.56.11.3304-3307.1990. [DOI] [PMC free article] [PubMed] [Google Scholar]
- Russell J. B., Strobel H. J. ATPase-dependent energy spilling by the ruminal bacterium, Streptococcus bovis. Arch Microbiol. 1990;153(4):378–383. doi: 10.1007/BF00249009. [DOI] [PubMed] [Google Scholar]
- Stouthamer A. H. A theoretical study on the amount of ATP required for synthesis of microbial cell material. Antonie Van Leeuwenhoek. 1973;39(3):545–565. doi: 10.1007/BF02578899. [DOI] [PubMed] [Google Scholar]
- Stouthamer A. H., Bettenhaussen C. Utilization of energy for growth and maintenance in continuous and batch cultures of microorganisms. A reevaluation of the method for the determination of ATP production by measuring molar growth yields. Biochim Biophys Acta. 1973 Feb 12;301(1):53–70. doi: 10.1016/0304-4173(73)90012-8. [DOI] [PubMed] [Google Scholar]
- Zoratti M., Favaron M., Pietrobon D., Azzone G. F. Intrinsic uncoupling of mitochondrial proton pumps. 1. Non-ohmic conductance cannot account for the nonlinear dependence of static head respiration on delta microH. Biochemistry. 1986 Feb 25;25(4):760–767. doi: 10.1021/bi00352a004. [DOI] [PubMed] [Google Scholar]