Abstract
Flux balance models of metabolism use stoichiometry of metabolic pathways, metabolic demands of growth, and optimality principles to predict metabolic flux distribution and cellular growth under specified environmental conditions. These models have provided a mechanistic interpretation of systemic metabolic physiology, and they are also useful as a quantitative tool for metabolic pathway design. Quantitative predictions of cell growth and metabolic by-product secretion that are experimentally testable can be obtained from these models. In the present report, we used independent measurements to determine the model parameters for the wild-type Escherichia coli strain W3110. We experimentally determined the maximum oxygen utilization rate (15 mmol of O2 per g [dry weight] per h), the maximum aerobic glucose utilization rate (10.5 mmol of Glc per g [dry weight] per h), the maximum anaerobic glucose utilization rate (18.5 mmol of Glc per g [dry weight] per h), the non-growth-associated maintenance requirements (7.6 mmol of ATP per g [dry weight] per h), and the growth-associated maintenance requirements (13 mmol of ATP per g of biomass). The flux balance model specified by these parameters was found to quantitatively predict glucose and oxygen uptake rates as well as acetate secretion rates observed in chemostat experiments. We have formulated a predictive algorithm in order to apply the flux balance model to describe unsteady-state growth and by-product secretion in aerobic batch, fed-batch, and anaerobic batch cultures. In aerobic experiments we observed acetate secretion, accumulation in the culture medium, and reutilization from the culture medium. In fed-batch cultures acetate is cometabolized with glucose during the later part of the culture period.(ABSTRACT TRUNCATED AT 250 WORDS)
Full text
PDF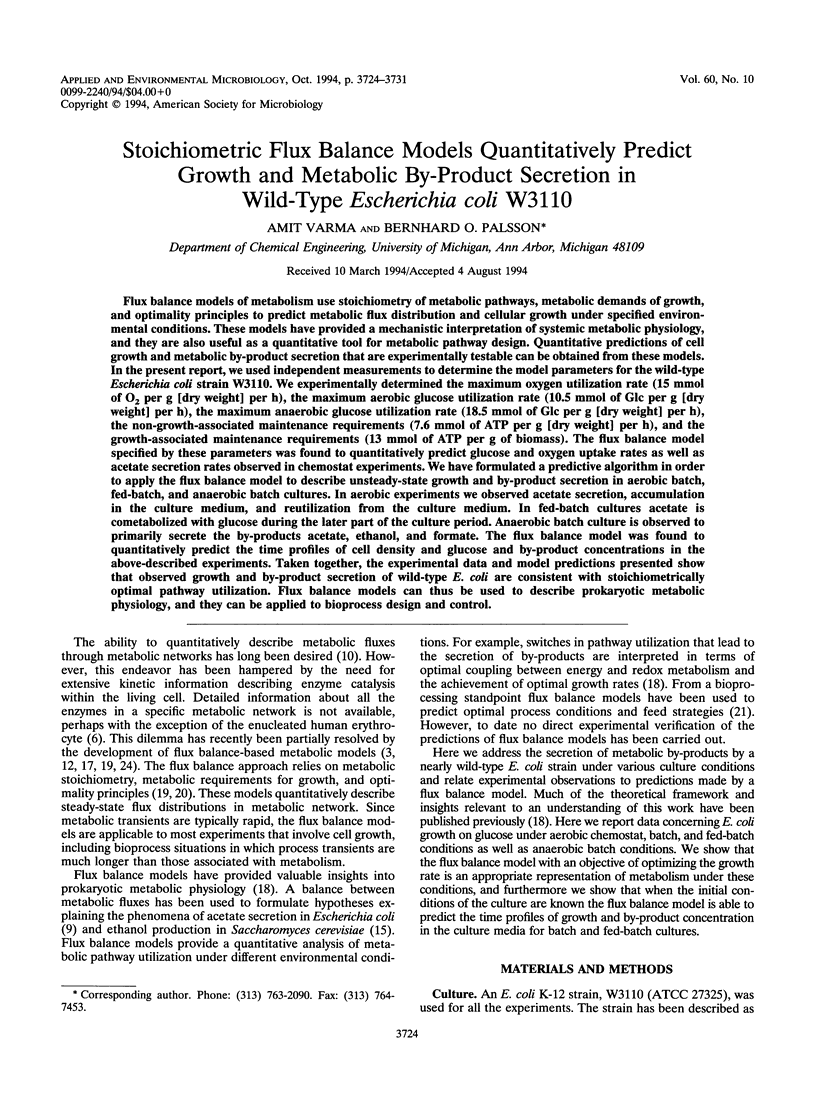
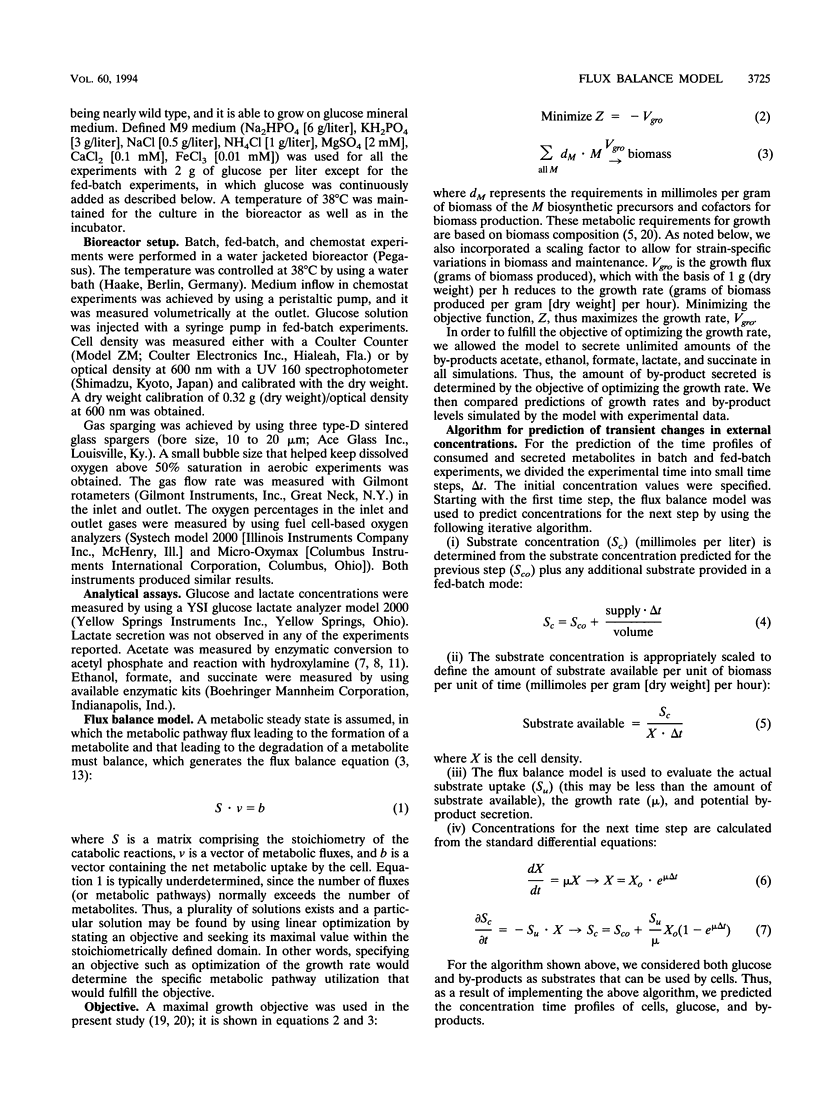
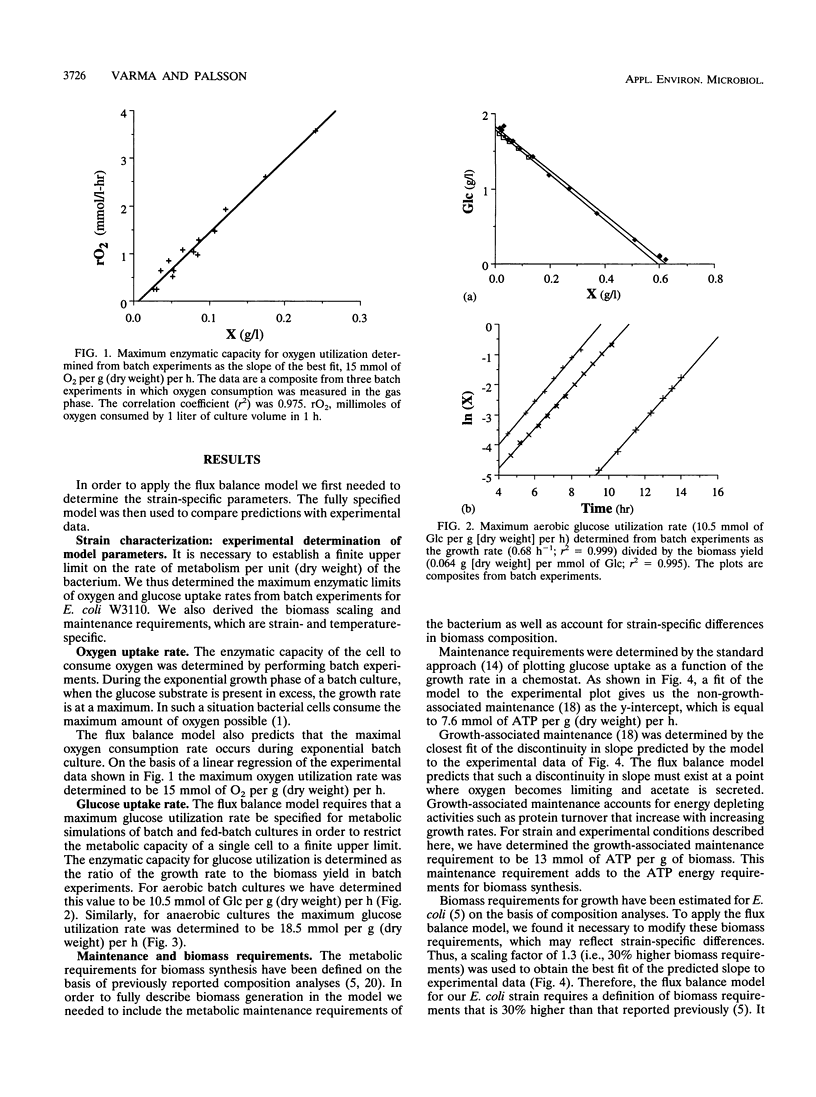
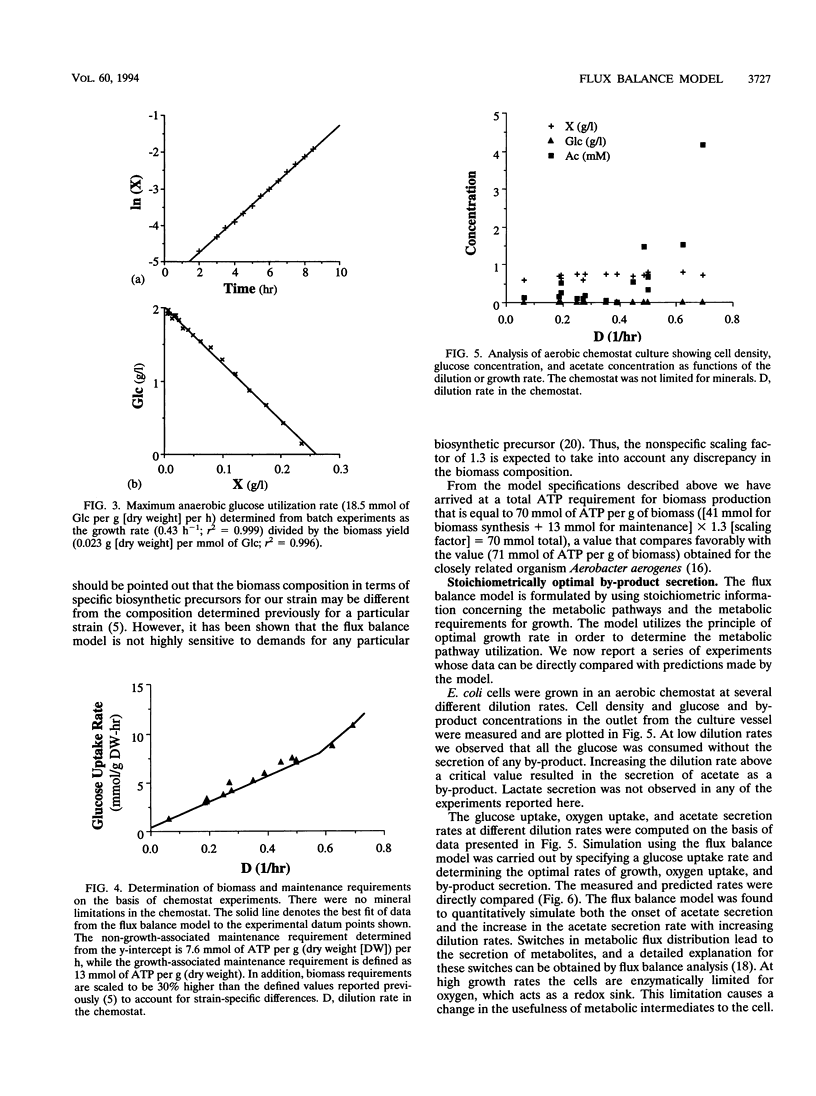
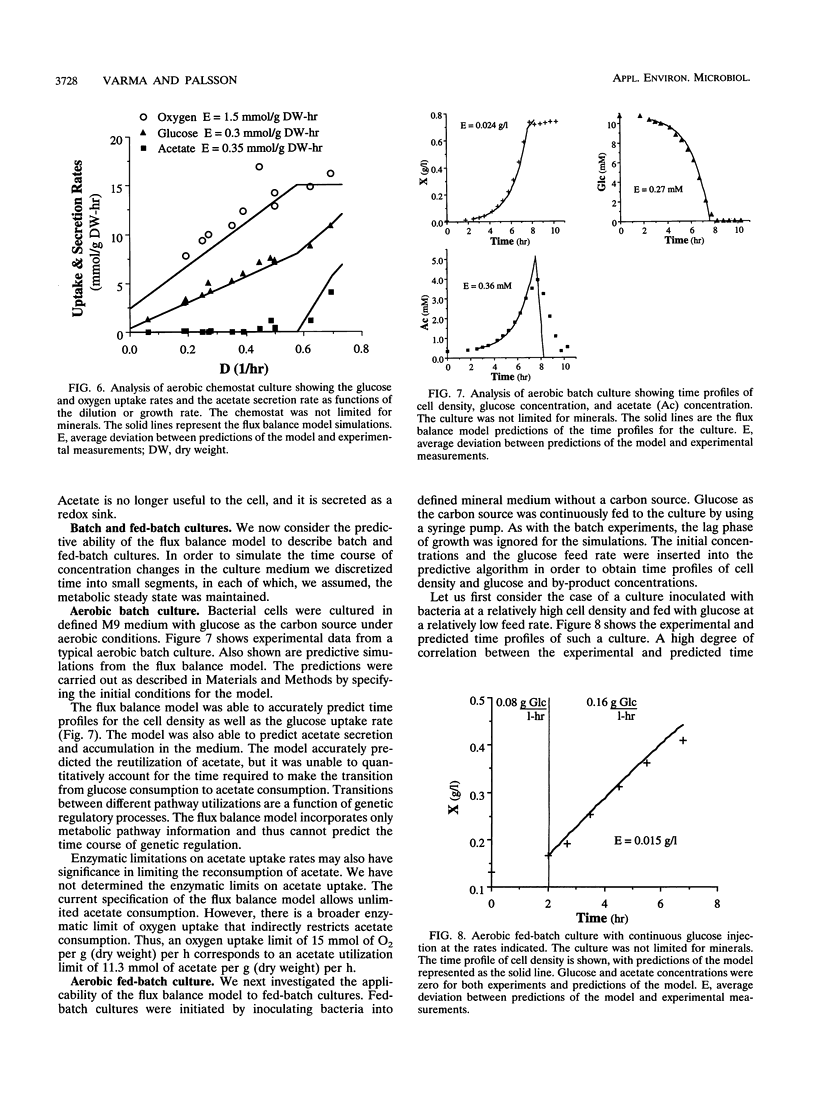
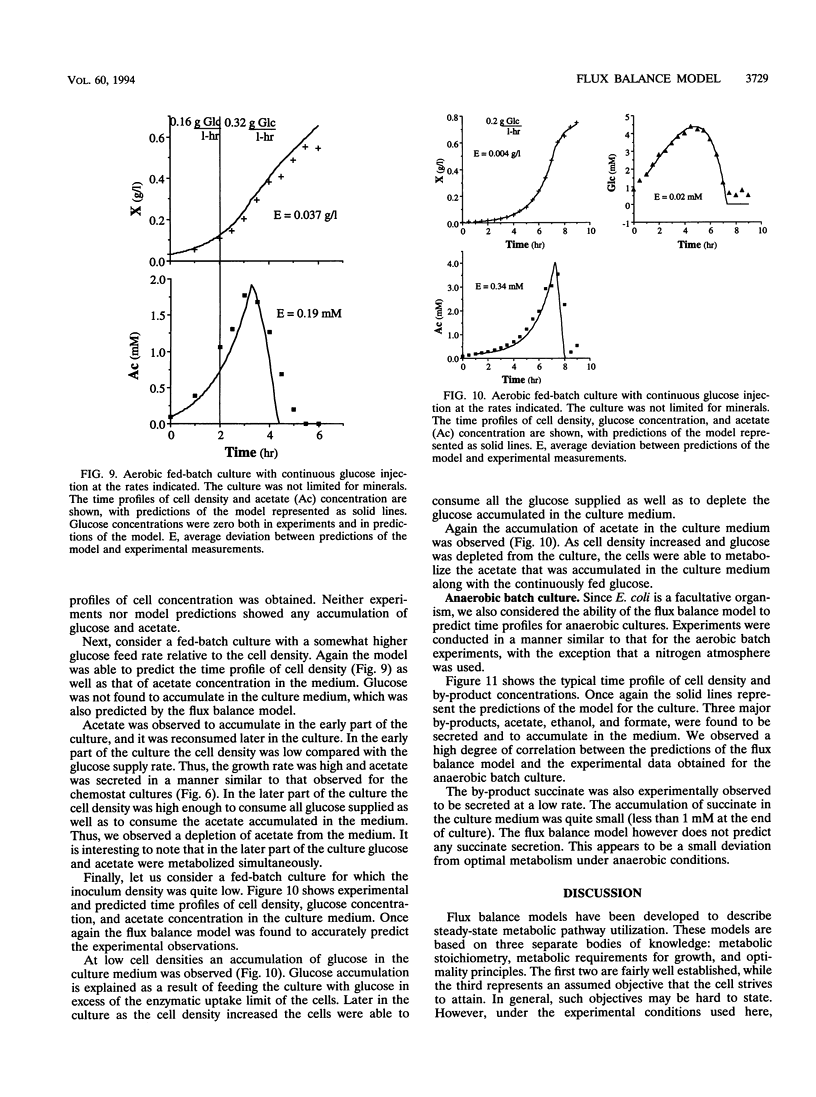
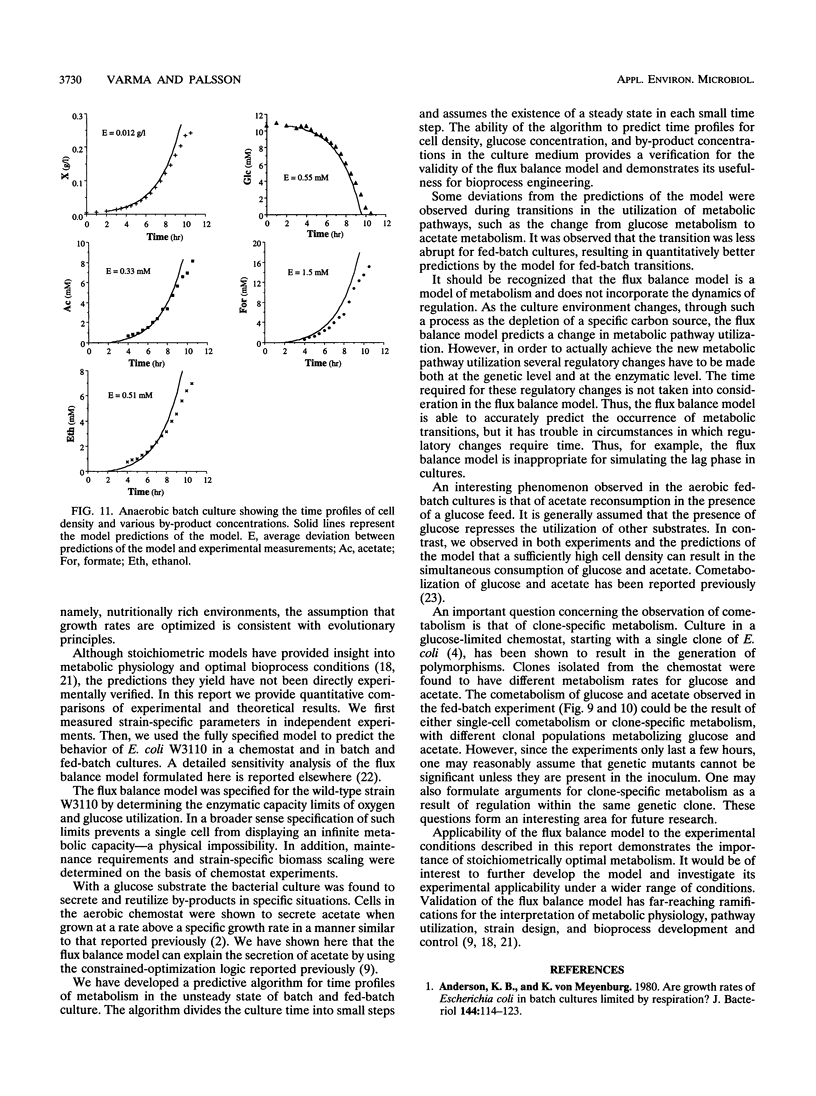
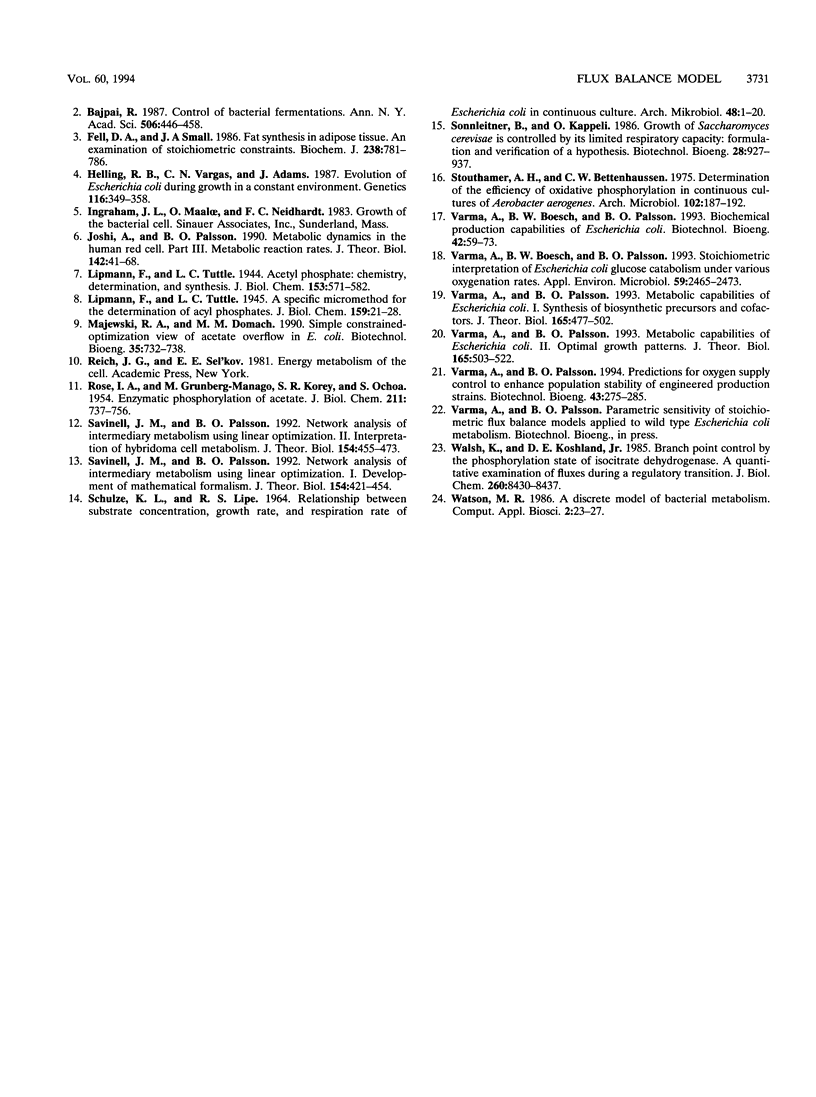
Selected References
These references are in PubMed. This may not be the complete list of references from this article.
- Andersen K. B., von Meyenburg K. Are growth rates of Escherichia coli in batch cultures limited by respiration? J Bacteriol. 1980 Oct;144(1):114–123. doi: 10.1128/jb.144.1.114-123.1980. [DOI] [PMC free article] [PubMed] [Google Scholar]
- Bajpai R. Control of bacterial fermentations. Ann N Y Acad Sci. 1987;506:446–458. doi: 10.1111/j.1749-6632.1987.tb23840.x. [DOI] [PubMed] [Google Scholar]
- Fell D. A., Small J. R. Fat synthesis in adipose tissue. An examination of stoichiometric constraints. Biochem J. 1986 Sep 15;238(3):781–786. doi: 10.1042/bj2380781. [DOI] [PMC free article] [PubMed] [Google Scholar]
- Helling R. B., Vargas C. N., Adams J. Evolution of Escherichia coli during growth in a constant environment. Genetics. 1987 Jul;116(3):349–358. doi: 10.1093/genetics/116.3.349. [DOI] [PMC free article] [PubMed] [Google Scholar]
- Joshi A., Palsson B. O. Metabolic dynamics in the human red cell. Part III--Metabolic reaction rates. J Theor Biol. 1990 Jan 9;142(1):41–68. doi: 10.1016/s0022-5193(05)80012-8. [DOI] [PubMed] [Google Scholar]
- ROSE I. A., GRUNBERG-MANAGO M., KOREY S. R., OCHOA S. Enzymatic phosphorylation of acetate. J Biol Chem. 1954 Dec;211(2):737–756. [PubMed] [Google Scholar]
- SCHULZE K. L., LIPE R. S. RELATIONSHIP BETWEEN SUBSTRATE CONCENTRATION, GROWTH RATE, AND RESPIRATION RATE OF ESCHERICHIA COLI IN CONTINUOUS CULTURE. Arch Mikrobiol. 1964 Apr 2;48:1–20. doi: 10.1007/BF00406595. [DOI] [PubMed] [Google Scholar]
- Savinell J. M., Palsson B. O. Network analysis of intermediary metabolism using linear optimization. I. Development of mathematical formalism. J Theor Biol. 1992 Feb 21;154(4):421–454. doi: 10.1016/s0022-5193(05)80161-4. [DOI] [PubMed] [Google Scholar]
- Savinell J. M., Palsson B. O. Network analysis of intermediary metabolism using linear optimization. II. Interpretation of hybridoma cell metabolism. J Theor Biol. 1992 Feb 21;154(4):455–473. doi: 10.1016/s0022-5193(05)80162-6. [DOI] [PubMed] [Google Scholar]
- Stouthamer A. H., Bettenhaussen C. W. Determination of the efficiency of oxidative phosphorylation in continuous cultures of Aerobacter aerogenes. Arch Microbiol. 1975 Mar 10;102(3):187–192. doi: 10.1007/BF00428367. [DOI] [PubMed] [Google Scholar]
- Varma A., Boesch B. W., Palsson B. O. Stoichiometric interpretation of Escherichia coli glucose catabolism under various oxygenation rates. Appl Environ Microbiol. 1993 Aug;59(8):2465–2473. doi: 10.1128/aem.59.8.2465-2473.1993. [DOI] [PMC free article] [PubMed] [Google Scholar]
- Walsh K., Koshland D. E., Jr Branch point control by the phosphorylation state of isocitrate dehydrogenase. A quantitative examination of fluxes during a regulatory transition. J Biol Chem. 1985 Jul 15;260(14):8430–8437. [PubMed] [Google Scholar]
- Watson M. R. A discrete model of bacterial metabolism. Comput Appl Biosci. 1986 Apr;2(1):23–27. doi: 10.1093/bioinformatics/2.1.23. [DOI] [PubMed] [Google Scholar]