Abstract
The conversion factor for the calculation of bacterial production from rates of [3H]thymidine incorporation was examined with diluted batch cultures of freshwater bacteria. Natural bacterial assemblages were grown in aged, normal, and enriched media at 10 to 20°C. The generation time during 101 growth cycles covered a range from 4 to >200 h. The average conversion factor was 2.15 × 1018 cells mol-1 of thymidine incorporated into the trichloroacetic acid (TCA) precipitate (standard error = 0.29 × 1018; n = 54), when the generation time exceeded 20 h. At generation times of <20 h, the average conversion factor was 11.8 × 1018 cells mol-1 of thymidine incorporated into TCA precipitate (standard error = 1.72 × 1018; n = 47). The amount of radioactivity in purified DNA increased with decreasing generation time and increasing conversion factor (calculated from the TCA precipitate), corresponding to a decrease in the percentage in protein. The conversion factors calculated from purified DNA or from the TCA precipitate gave the same variability. Conversion factors did not change significantly with the medium, but were significantly higher at 20°C than at 15 and 10°C. A detailed examination of the [3H]thymidine concentrations that were needed to achieve maximum labeling in DNA was carried out 6 times during a complete growth cycle. During periods with low generation times and high conversion factors, 15 nM [3H]thymidine was enough for the maximum labeling of the TCA precipitate. This suggests that incorporation of [3H]thymidine into DNA is probably limited by uptake during periods with generation times of <20 h and that freshwater bacterioplankton cell production sometimes is underestimated when a conversion factor of 2.15 × 1018 cells mol-1 of thymidine incorporated is used.
Full text
PDF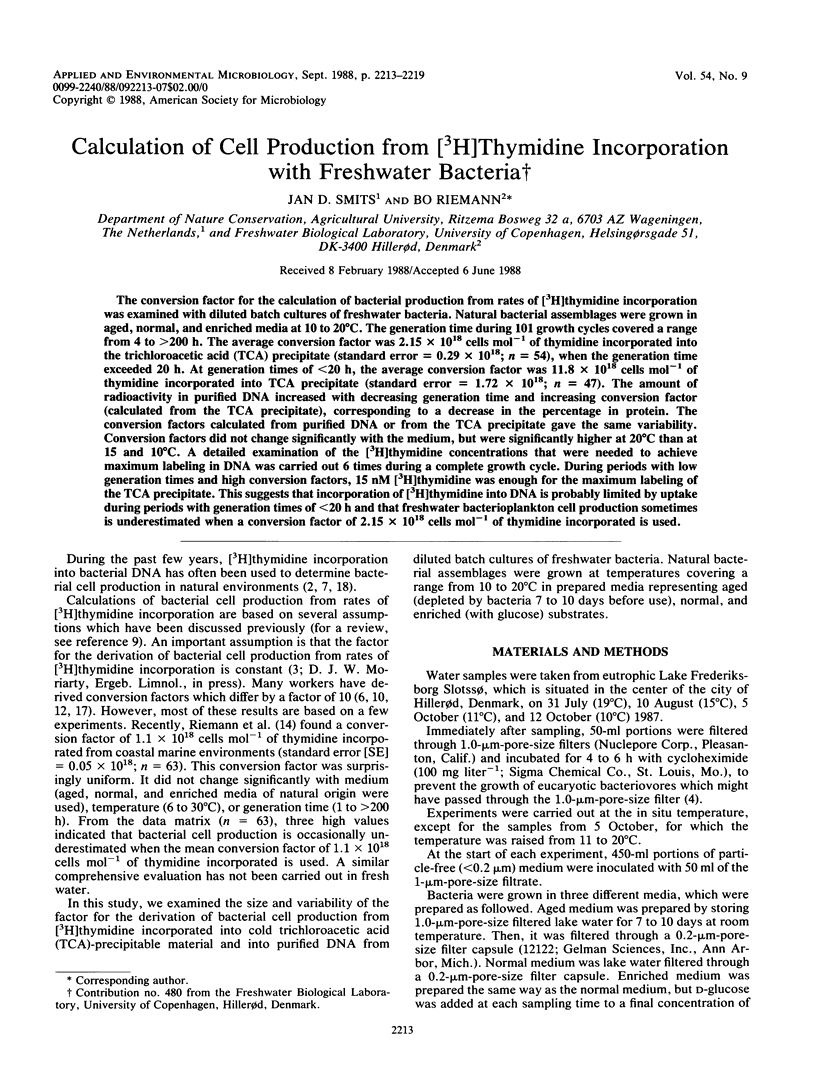
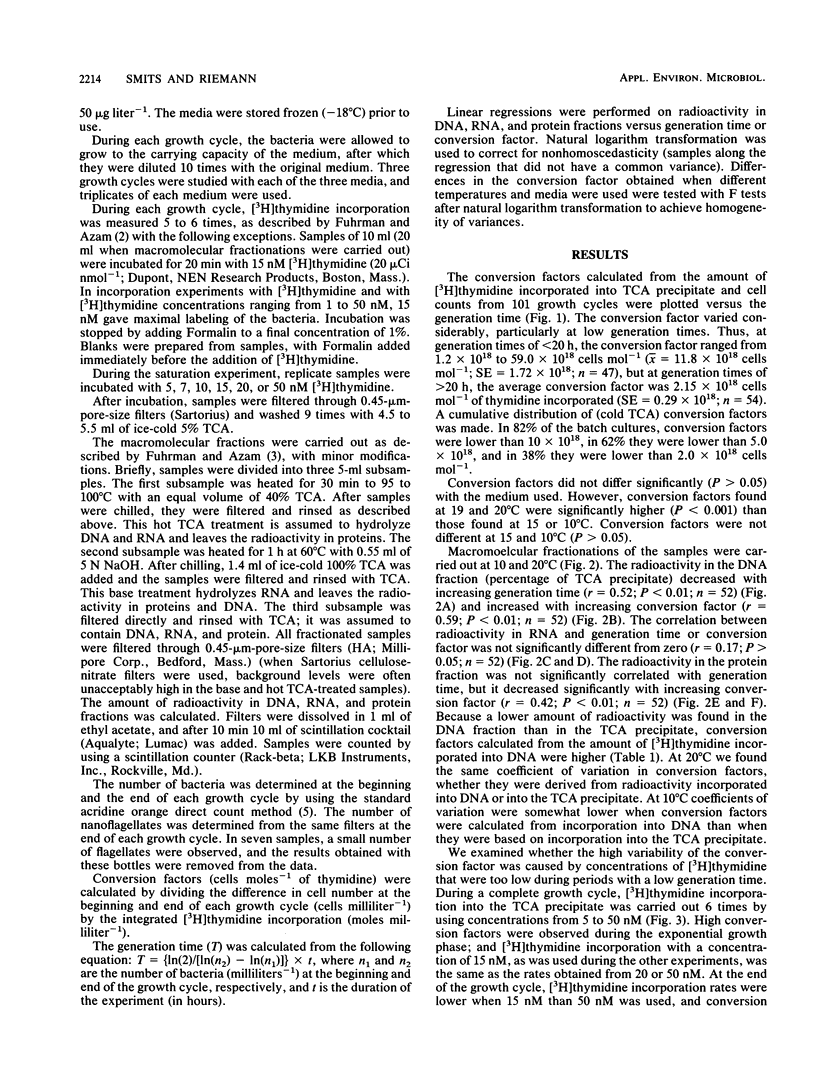
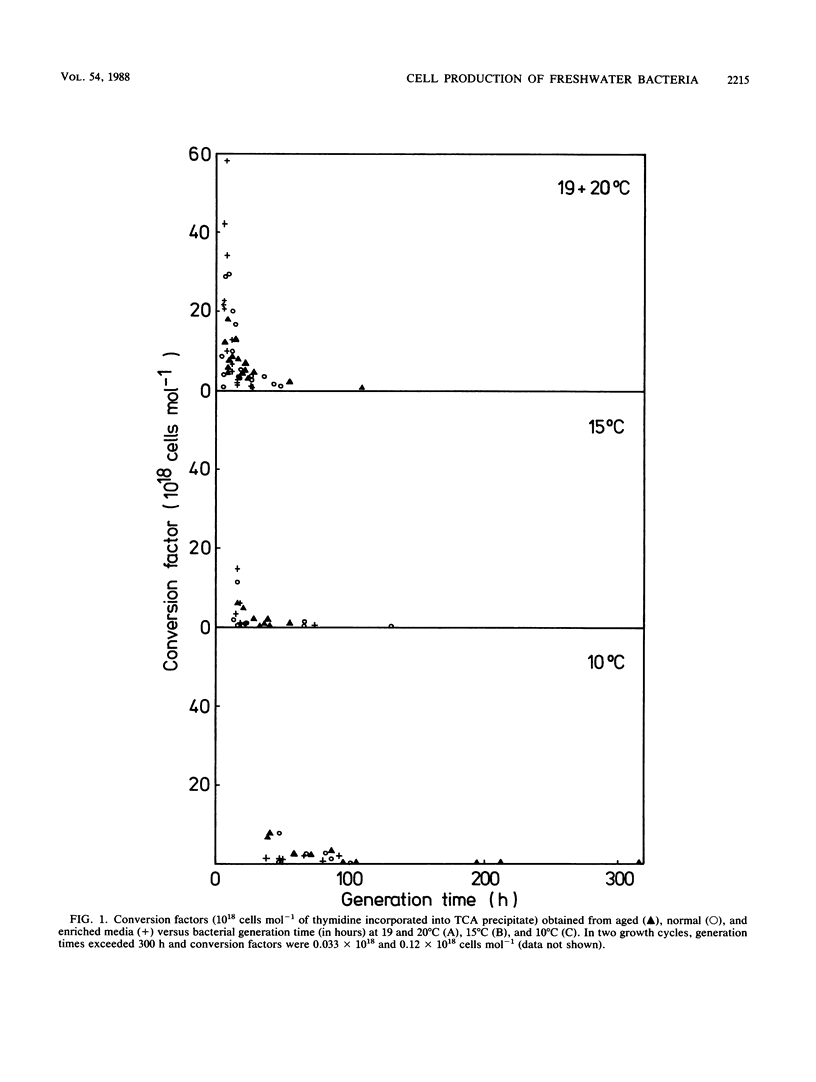
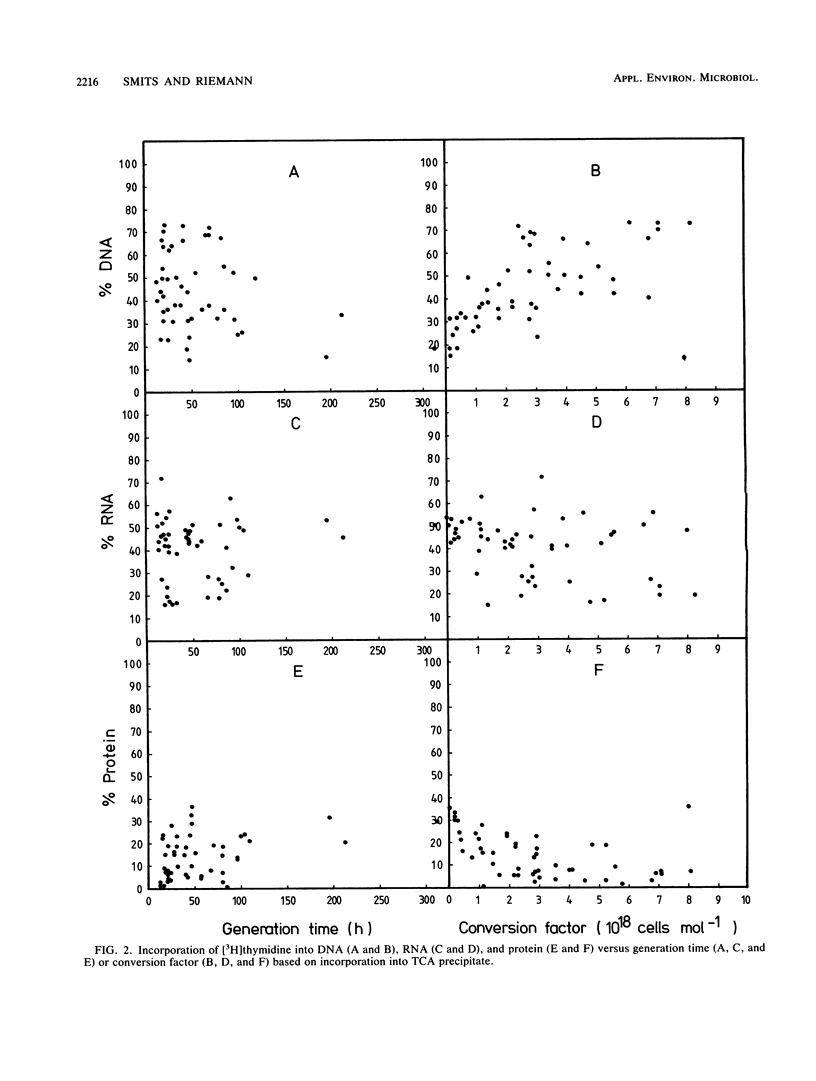
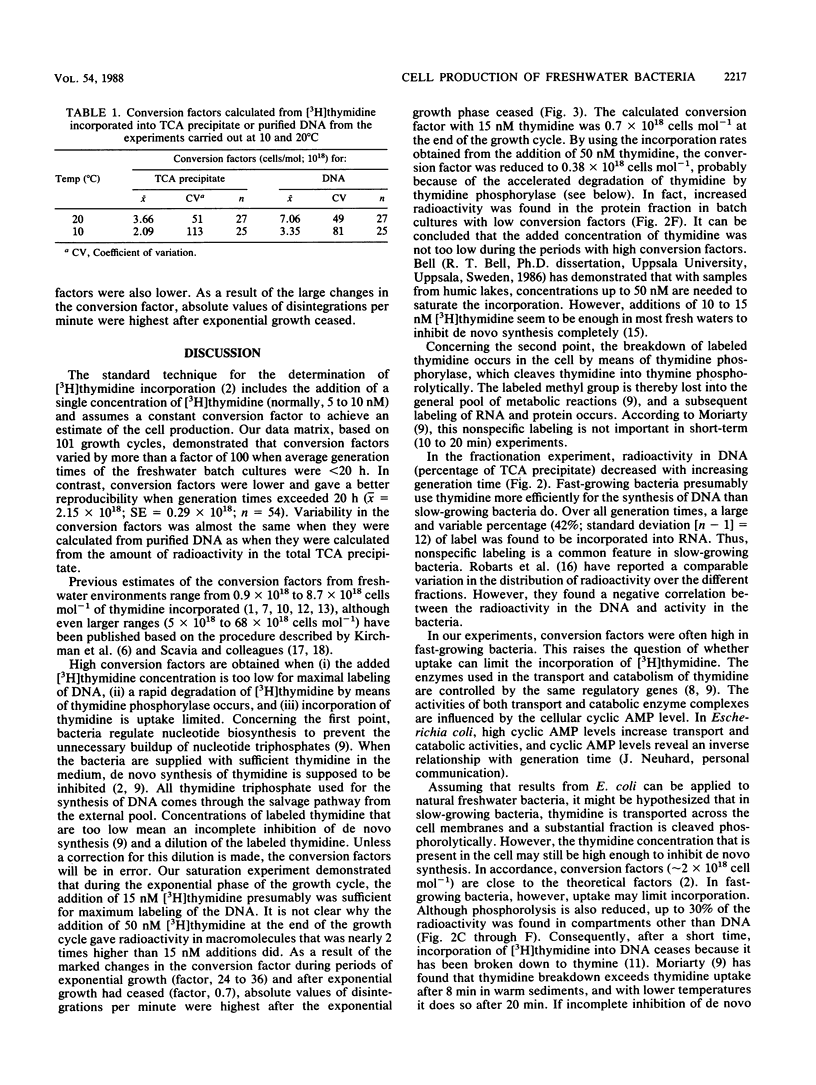
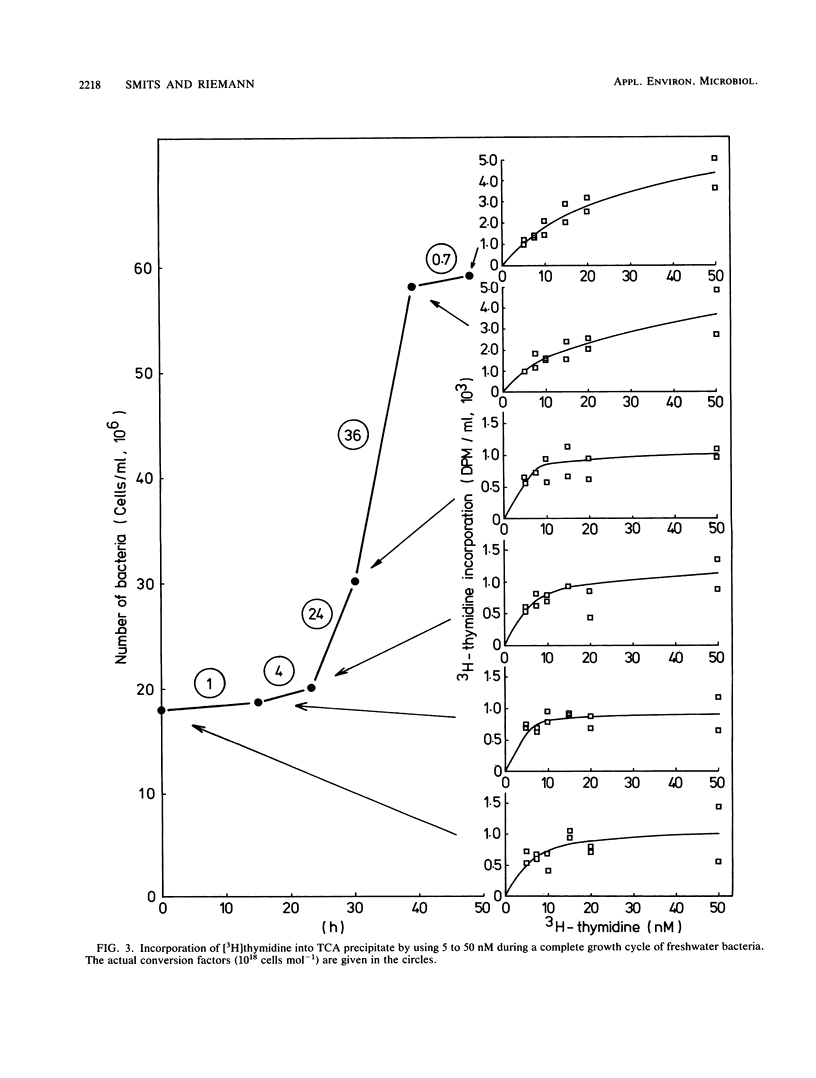
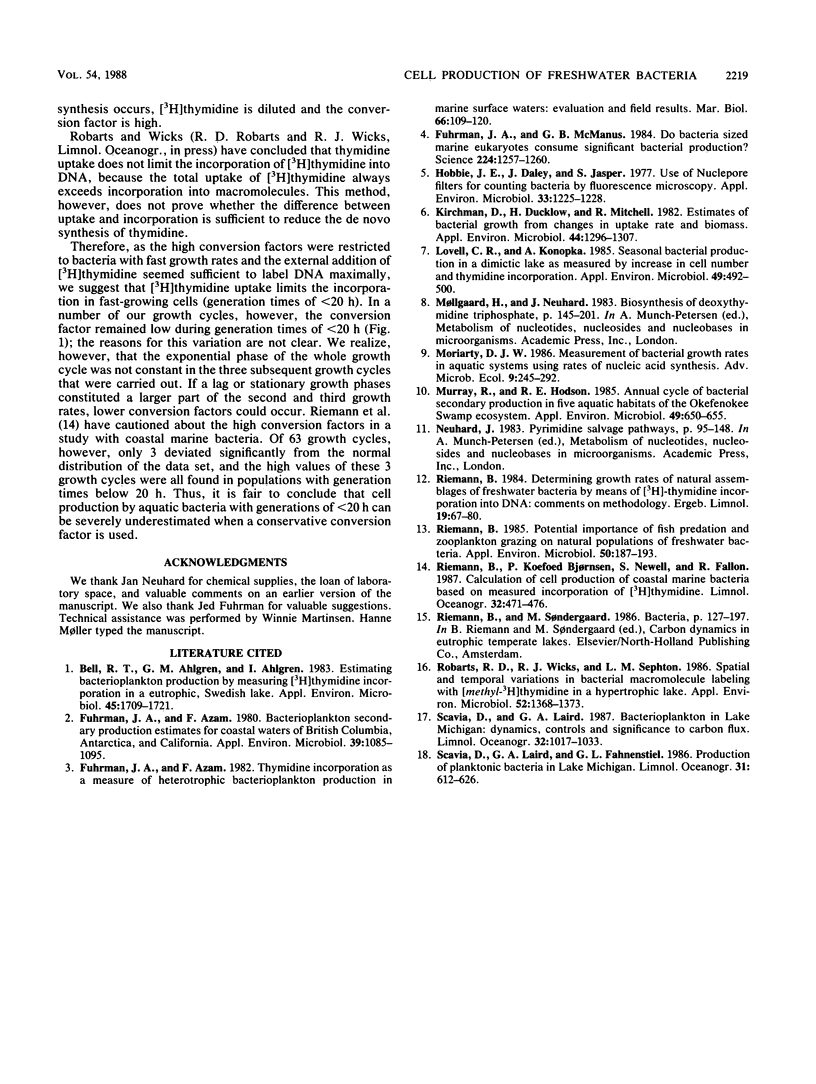
Selected References
These references are in PubMed. This may not be the complete list of references from this article.
- Bell R. T., Ahlgren G. M., Ahlgren I. Estimating Bacterioplankton Production by Measuring [H]thymidine Incorporation in a Eutrophic Swedish Lake. Appl Environ Microbiol. 1983 Jun;45(6):1709–1721. doi: 10.1128/aem.45.6.1709-1721.1983. [DOI] [PMC free article] [PubMed] [Google Scholar]
- Fuhrman J. A., Azam F. Bacterioplankton secondary production estimates for coastal waters of british columbia, antarctica, and california. Appl Environ Microbiol. 1980 Jun;39(6):1085–1095. doi: 10.1128/aem.39.6.1085-1095.1980. [DOI] [PMC free article] [PubMed] [Google Scholar]
- Fuhrman J. A., McManus G. B. Do bacteria-sized marine eukaryotes consume significant bacterial production? Science. 1984 Jun 15;224(4654):1257–1260. doi: 10.1126/science.224.4654.1257. [DOI] [PubMed] [Google Scholar]
- Hobbie J. E., Daley R. J., Jasper S. Use of nuclepore filters for counting bacteria by fluorescence microscopy. Appl Environ Microbiol. 1977 May;33(5):1225–1228. doi: 10.1128/aem.33.5.1225-1228.1977. [DOI] [PMC free article] [PubMed] [Google Scholar]
- Kirchman D., Ducklow H., Mitchell R. Estimates of bacterial growth from changes in uptake rates and biomass. Appl Environ Microbiol. 1982 Dec;44(6):1296–1307. doi: 10.1128/aem.44.6.1296-1307.1982. [DOI] [PMC free article] [PubMed] [Google Scholar]
- Lovell C. R., Konopka A. Seasonal bacterial production in a dimictic lake as measured by increases in cell numbers and thymidine incorporation. Appl Environ Microbiol. 1985 Mar;49(3):492–500. doi: 10.1128/aem.49.3.492-500.1985. [DOI] [PMC free article] [PubMed] [Google Scholar]
- Murray R. E., Hodson R. E. Annual cycle of bacterial secondary production in five aquatic habitats of the okefenokee swamp ecosystem. Appl Environ Microbiol. 1985 Mar;49(3):650–655. doi: 10.1128/aem.49.3.650-655.1985. [DOI] [PMC free article] [PubMed] [Google Scholar]
- Riemann B. Potential importance of fish predation and zooplankton grazing on natural populations of freshwater bacteria. Appl Environ Microbiol. 1985 Aug;50(2):187–193. doi: 10.1128/aem.50.2.187-193.1985. [DOI] [PMC free article] [PubMed] [Google Scholar]
- Robarts R. D., Wicks R. J., Sephton L. M. Spatial and Temporal Variations in Bacterial Macromolecule Labeling with [methyl-H]Thymidine in a Hypertrophic Lake. Appl Environ Microbiol. 1986 Dec;52(6):1368–1373. doi: 10.1128/aem.52.6.1368-1373.1986. [DOI] [PMC free article] [PubMed] [Google Scholar]