Abstract
Aeroadaptive microaerophilic Azotobacter chroococcum 184 produced a cell-associated black pigment when grown at high aeration rates under nitrogen-fixing conditions. This pigment was shown to be a catechol melanin. Polyphenol oxidase activity was detected in cell extracts of cells grown for 72 h. Melanin formation was optimal in the later stages of growth, and there was no correlation between nitrogenase activity and melanization. Nitrogenase activity in strain 184 was optimal at 10% O2, and melanin formation was suppressed by O2 limitation. In the presence of charcoal, an adsorbent of toxic oxygen intermediates, and benzoic acid, a scavenger of hydroxyl radicals, melanization was inhibited. However, in the presence of copper, the intensity of pigment color increased and melanization was accelerated. Copper also eliminated catalase and peroxidase activities of the organism but still permitted aerobic growth. In the presence of low levels of iron, melanization was accelerated under high aeration rates, and under low rates of aeration, melanization was observed only at higher levels of iron. Hydroxamate-siderophore production was detectable in the presence of soluble iron under high rates of aeration but was repressed by the same levels of iron under low aeration rates. Unlike melanization and hydroxamate formation, catechol formation was observed under both low and high rates of aeration under nitrogen-fixing conditions. Catechol formation and melanization were repressed by 14 mM NH4+, at which level nitrogenase activity was also repressed. Copper reversed the repressive effect of NH4+. A role for catechol formation and melanization in aeroadaptation is proposed.
Full text
PDF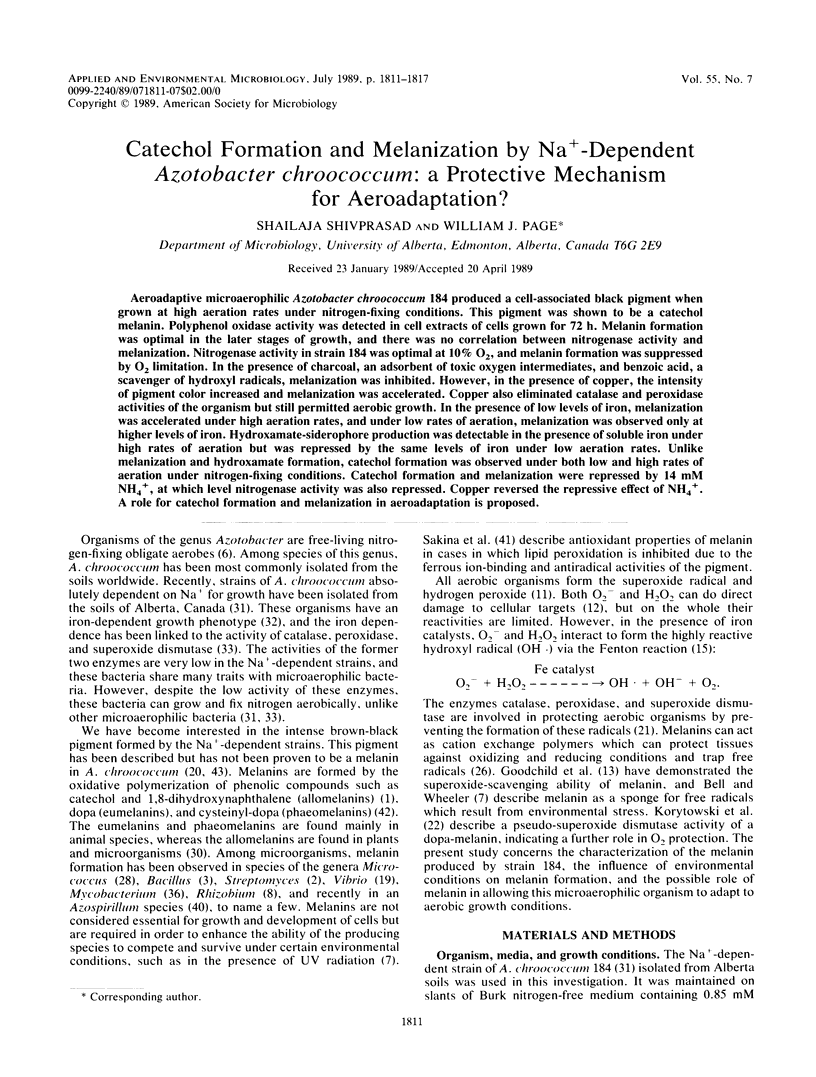
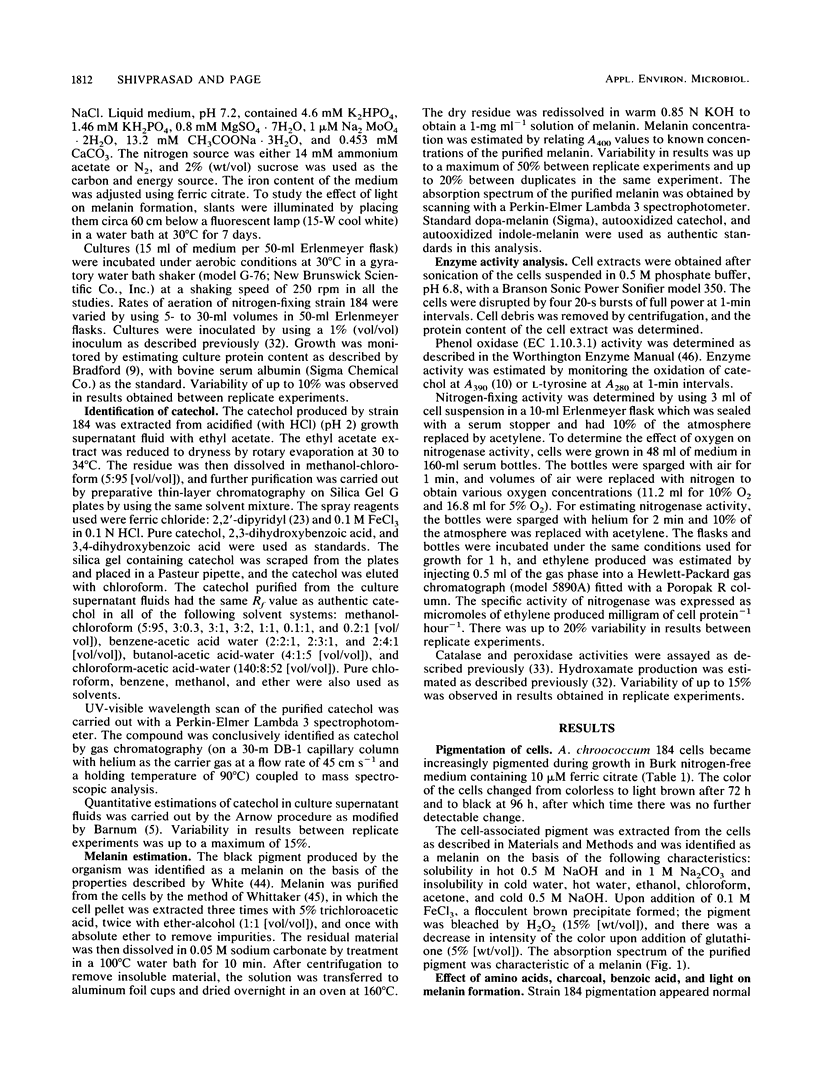
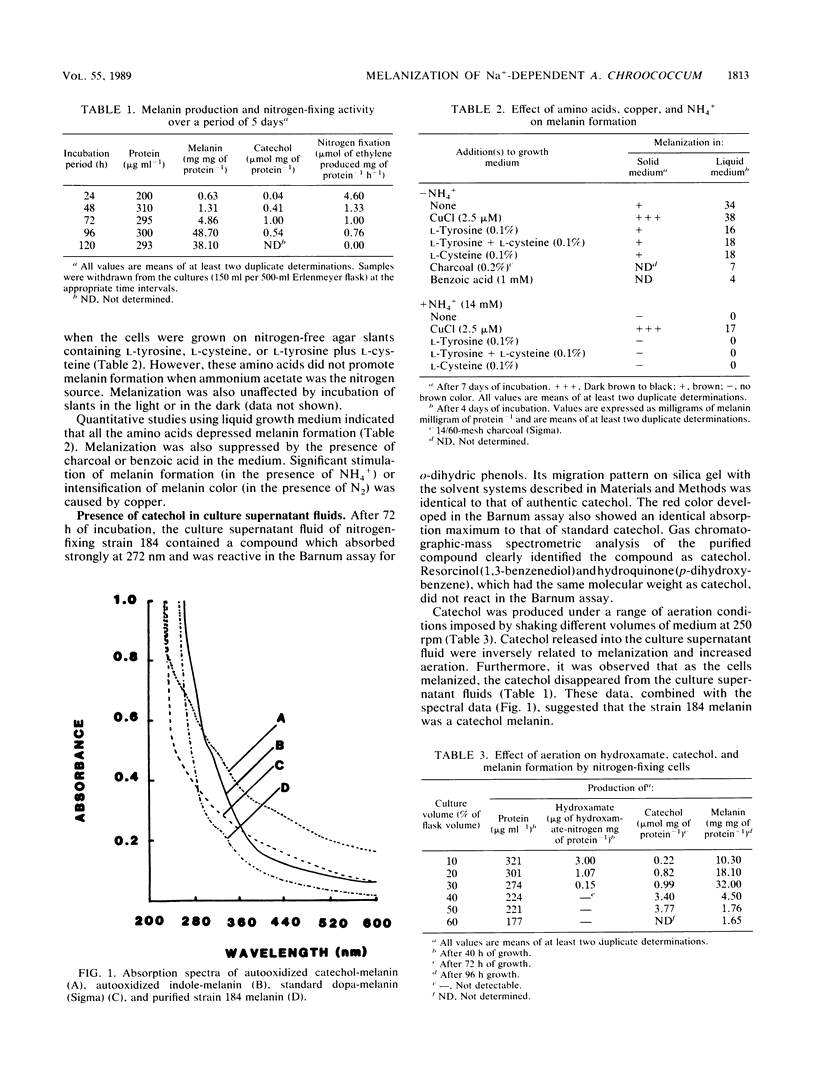
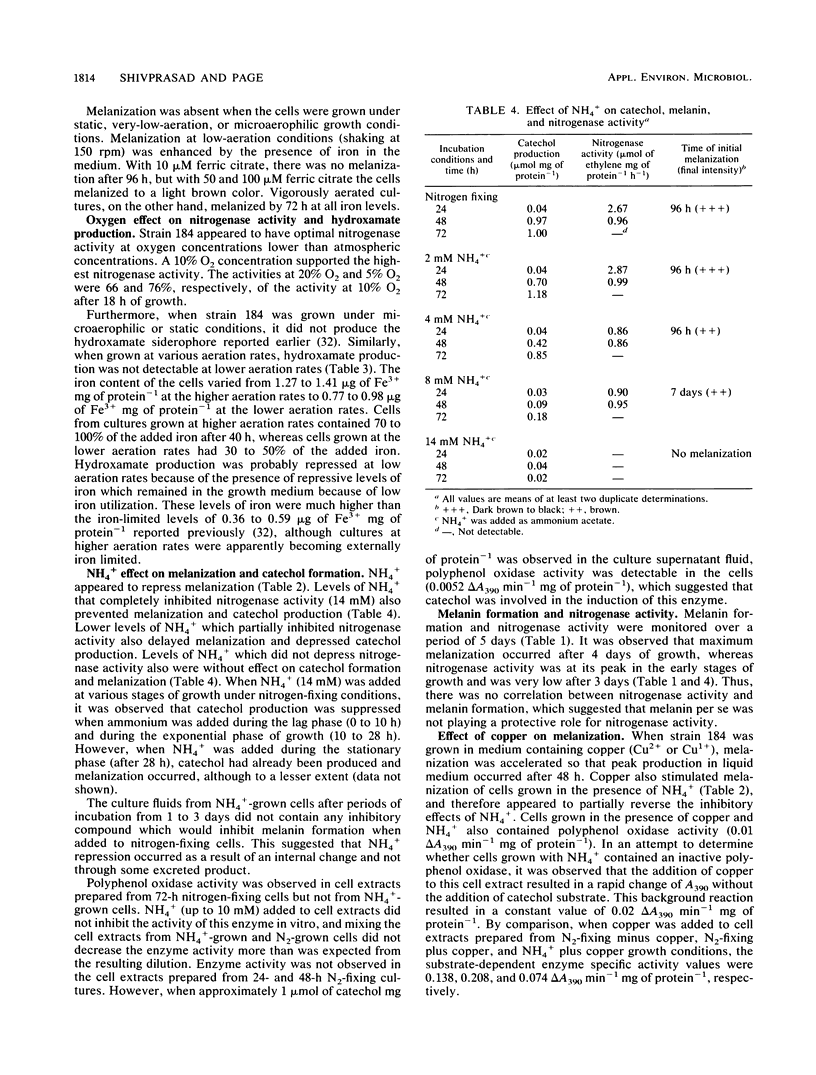
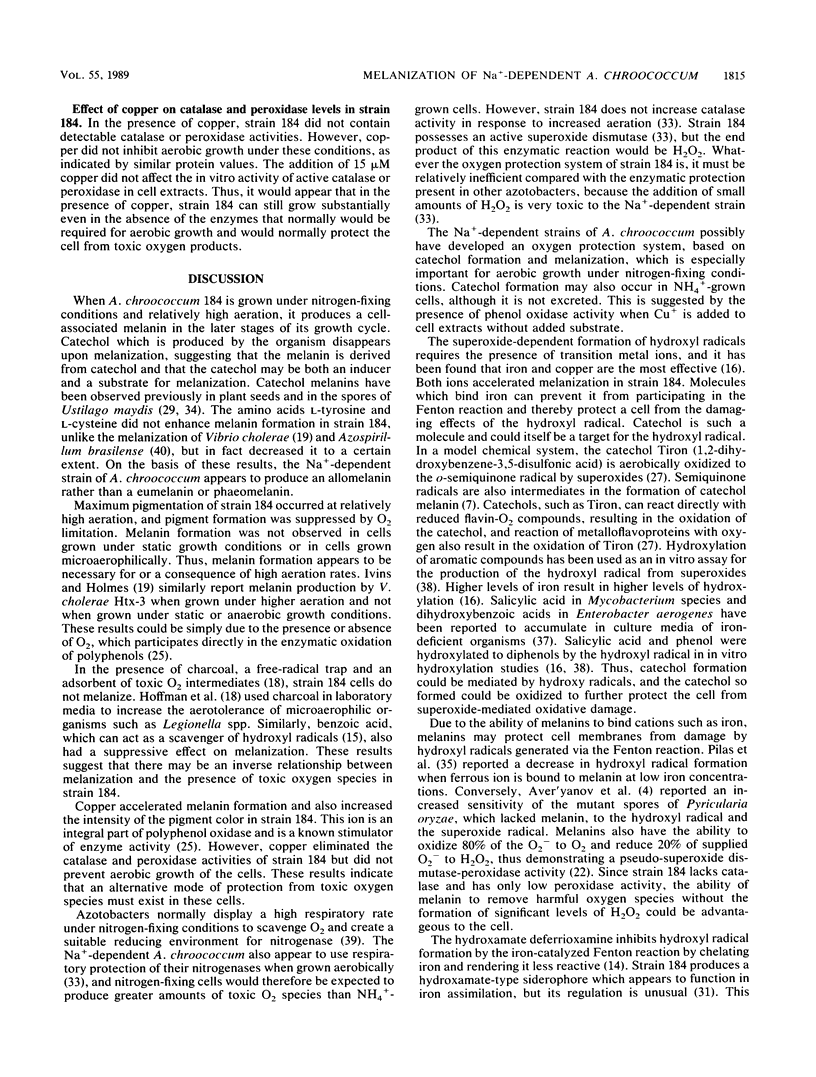
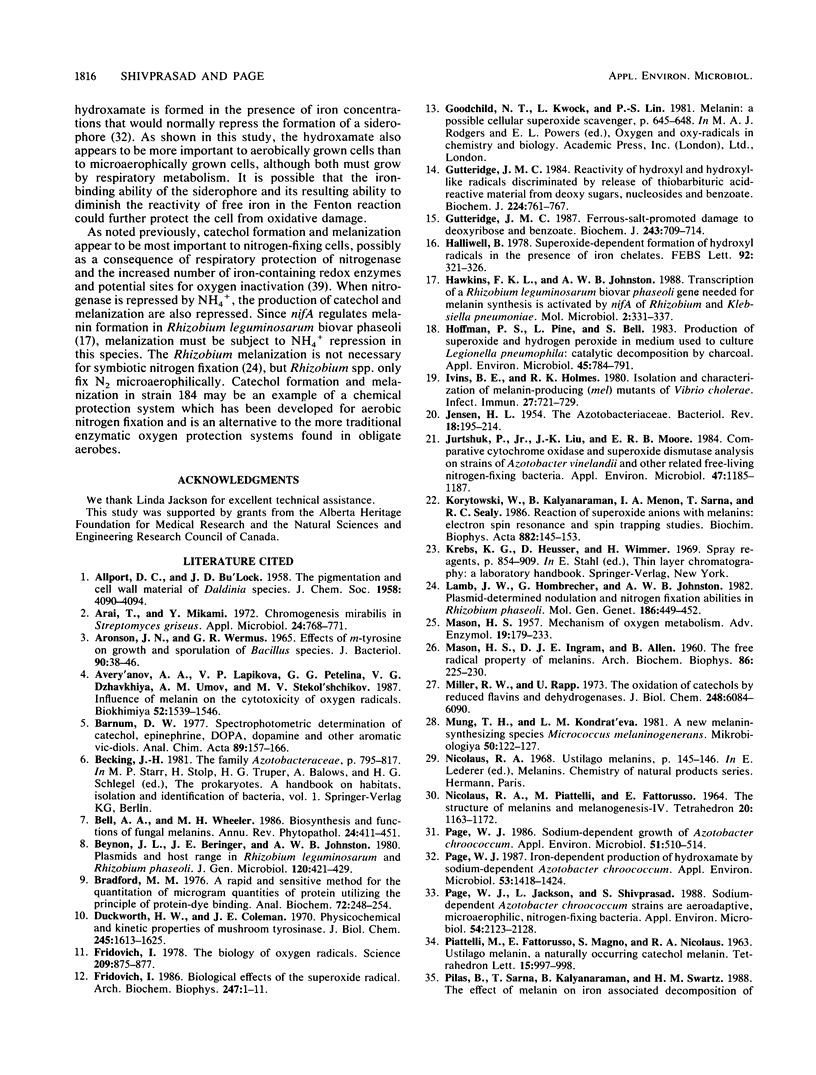
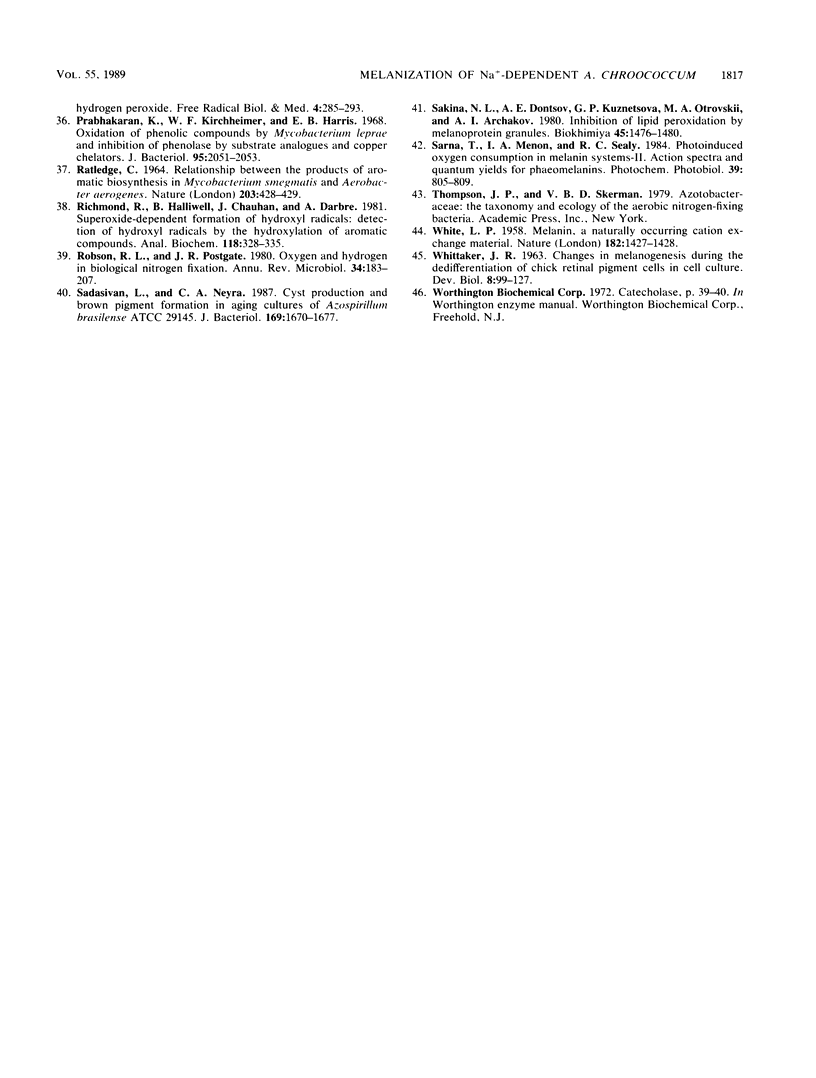
Selected References
These references are in PubMed. This may not be the complete list of references from this article.
- Arai T., Mikami Y. Chromogenesis mirabilis in Streptomyces griseus. Appl Microbiol. 1972 Nov;24(5):768–771. doi: 10.1128/am.24.5.768-771.1972. [DOI] [PMC free article] [PubMed] [Google Scholar]
- Aronson J. N., Wermus G. R. Effects of m-Tyrosine on Growth and Sporulation of Bacillus Species. J Bacteriol. 1965 Jul;90(1):38–46. doi: 10.1128/jb.90.1.38-46.1965. [DOI] [PMC free article] [PubMed] [Google Scholar]
- Barnum D. W. Spectrophotometric determination of catechol, epinephrine, dopa, dopamine and other aromatic vic-diols. Anal Chim Acta. 1977 Mar;89(1):157–166. doi: 10.1016/S0003-2670(01)83081-6. [DOI] [PubMed] [Google Scholar]
- Bradford M. M. A rapid and sensitive method for the quantitation of microgram quantities of protein utilizing the principle of protein-dye binding. Anal Biochem. 1976 May 7;72:248–254. doi: 10.1016/0003-2697(76)90527-3. [DOI] [PubMed] [Google Scholar]
- Duckworth H. W., Coleman J. E. Physicochemical and kinetic properties of mushroom tyrosinase. J Biol Chem. 1970 Apr 10;245(7):1613–1625. [PubMed] [Google Scholar]
- Fridovich I. Biological effects of the superoxide radical. Arch Biochem Biophys. 1986 May 15;247(1):1–11. doi: 10.1016/0003-9861(86)90526-6. [DOI] [PubMed] [Google Scholar]
- Fridovich I. The biology of oxygen radicals. Science. 1978 Sep 8;201(4359):875–880. doi: 10.1126/science.210504. [DOI] [PubMed] [Google Scholar]
- Gutteridge J. M. Ferrous-salt-promoted damage to deoxyribose and benzoate. The increased effectiveness of hydroxyl-radical scavengers in the presence of EDTA. Biochem J. 1987 May 1;243(3):709–714. doi: 10.1042/bj2430709. [DOI] [PMC free article] [PubMed] [Google Scholar]
- Gutteridge J. M. Reactivity of hydroxyl and hydroxyl-like radicals discriminated by release of thiobarbituric acid-reactive material from deoxy sugars, nucleosides and benzoate. Biochem J. 1984 Dec 15;224(3):761–767. doi: 10.1042/bj2240761. [DOI] [PMC free article] [PubMed] [Google Scholar]
- Halliwell B. Superoxide-dependent formation of hydroxyl radicals in the presence of iron chelates: is it a mechanism for hydroxyl radical production in biochemical systems? FEBS Lett. 1978 Aug 15;92(2):321–326. doi: 10.1016/0014-5793(78)80779-0. [DOI] [PubMed] [Google Scholar]
- Hawkins F. K., Johnston A. W. Transcription of a Rhizobium leguminosarum biovar phaseoli gene needed for melanin synthesis is activated by nifA of Rhizobium and Klebsiella pneumoniae. Mol Microbiol. 1988 May;2(3):331–337. doi: 10.1111/j.1365-2958.1988.tb00036.x. [DOI] [PubMed] [Google Scholar]
- Hoffman P. S., Pine L., Bell S. Production of superoxide and hydrogen peroxide in medium used to culture Legionella pneumophila: catalytic decomposition by charcoal. Appl Environ Microbiol. 1983 Mar;45(3):784–791. doi: 10.1128/aem.45.3.784-791.1983. [DOI] [PMC free article] [PubMed] [Google Scholar]
- Ivins B. E., Holmes R. K. Isolation and characterization of melanin-producing (mel) mutants of Vibrio cholerae. Infect Immun. 1980 Mar;27(3):721–729. doi: 10.1128/iai.27.3.721-729.1980. [DOI] [PMC free article] [PubMed] [Google Scholar]
- JENSEN H. L. The Azotobacteriaceae. Bacteriol Rev. 1954 Dec;18(4):195–214. doi: 10.1128/br.18.4.195-214.1954. [DOI] [PMC free article] [PubMed] [Google Scholar]
- Jurtshuk P., Liu J. K., Moore E. R. Comparative Cytochrome Oxidase and Superoxide Dismutase Analyses on Strains of Azotobacter vinelandii and Other Related Free-Living Nitrogen-Fixing Bacteria. Appl Environ Microbiol. 1984 May;47(5):1185–1187. doi: 10.1128/aem.47.5.1185-1187.1984. [DOI] [PMC free article] [PubMed] [Google Scholar]
- Korytowski W., Kalyanaraman B., Menon I. A., Sarna T., Sealy R. C. Reaction of superoxide anions with melanins: electron spin resonance and spin trapping studies. Biochim Biophys Acta. 1986 Jun 19;882(2):145–153. doi: 10.1016/0304-4165(86)90149-2. [DOI] [PubMed] [Google Scholar]
- MASON H. S., INGRAM D. J., ALLEN B. The free radical property of melanins. Arch Biochem Biophys. 1960 Feb;86:225–230. doi: 10.1016/0003-9861(60)90409-4. [DOI] [PubMed] [Google Scholar]
- MASON H. S. Mechanisms of oxygen metabolism. Adv Enzymol Relat Subj Biochem. 1957;19:79–233. doi: 10.1002/9780470122648.ch2. [DOI] [PubMed] [Google Scholar]
- Miller R. W., Rapp U. The oxidation of catechols by reduced flavins and dehydrogenases. An electron spin resonance study of the kinetics and initial products of oxidation. J Biol Chem. 1973 Sep 10;248(17):6084–6090. [PubMed] [Google Scholar]
- Nicolaus R. A., Piattelli M., Fattorusso E. The structure of melanins and melanogenesis. IV. On some natural melanins. Tetrahedron. 1964 May;20(5):1163–1172. doi: 10.1016/s0040-4020(01)98983-5. [DOI] [PubMed] [Google Scholar]
- Page W. J. Iron-Dependent Production of Hydroxamate by Sodium-Dependent Azotobacter chroococcum. Appl Environ Microbiol. 1987 Jul;53(7):1418–1424. doi: 10.1128/aem.53.7.1418-1424.1987. [DOI] [PMC free article] [PubMed] [Google Scholar]
- Page W. J., Jackson L., Shivprasad S. Sodium-Dependent Azotobacter chroococcum Strains Are Aeroadaptive, Microaerophilic, Nitrogen-Fixing Bacteria. Appl Environ Microbiol. 1988 Aug;54(8):2123–2128. doi: 10.1128/aem.54.8.2123-2128.1988. [DOI] [PMC free article] [PubMed] [Google Scholar]
- Page W. J. Sodium-Dependent Growth of Azotobacter chroococcum. Appl Environ Microbiol. 1986 Mar;51(3):510–514. doi: 10.1128/aem.51.3.510-514.1986. [DOI] [PMC free article] [PubMed] [Google Scholar]
- Prabhakaran K., Kirchheimer W. F., Harris E. B. Oxidation of phenolic compounds by Mycobacterium leprae and inhibition of phenolase by substrate analogues and copper chelators. J Bacteriol. 1968 Jun;95(6):2051–2053. doi: 10.1128/jb.95.6.2051-2053.1968. [DOI] [PMC free article] [PubMed] [Google Scholar]
- RATLEDGE C. RELATIONSHIP BETWEEN THE PRODUCTS OF AROMATIC BIOSYNTHESIS IN MYCOBACTERIUM SMEGMATIS AND AEROBACTER AEROGENES. Nature. 1964 Jul 25;203:428–429. doi: 10.1038/203428a0. [DOI] [PubMed] [Google Scholar]
- Richmond R., Halliwell B., Chauhan J., Darbre A. Superoxide-dependent formation of hydroxyl radicals: detection of hydroxyl radicals by the hydroxylation of aromatic compounds. Anal Biochem. 1981 Dec;118(2):328–335. doi: 10.1016/0003-2697(81)90590-x. [DOI] [PubMed] [Google Scholar]
- Robson R. L., Postgate J. R. Oxygen and hydrogen in biological nitrogen fixation. Annu Rev Microbiol. 1980;34:183–207. doi: 10.1146/annurev.mi.34.100180.001151. [DOI] [PubMed] [Google Scholar]
- Sadasivan L., Neyra C. A. Cyst production and brown pigment formation in aging cultures of Azospirillum brasilense ATCC 29145. J Bacteriol. 1987 Apr;169(4):1670–1677. doi: 10.1128/jb.169.4.1670-1677.1987. [DOI] [PMC free article] [PubMed] [Google Scholar]
- Sakina N. L., Dontsov A. E., Kuznetsova G. P., Ostrovskii M. A., Archakov A. I. Ingibirovania perekisnogo okisleniia lipidov melanoproteinovymi graulami. Biokhimiia. 1980 Aug;45(8):1476–1480. [PubMed] [Google Scholar]
- Sarna T., Menon I. A., Sealy R. C. Photoinduced oxygen consumption in melanin systems--II. Action spectra and quantum yields for pheomelanins. Photochem Photobiol. 1984 Jun;39(6):805–809. doi: 10.1111/j.1751-1097.1984.tb08863.x. [DOI] [PubMed] [Google Scholar]
- WHITE L. P. Melanin: a naturally occurring cation exchange material. Nature. 1958 Nov 22;182(4647):1427–1428. doi: 10.1038/1821427a0. [DOI] [PubMed] [Google Scholar]
- WHITTAKER J. R. CHANGES IN MELANOGENESIS DURING THE DEDIFFERENTIATION OF CHICK RETINAL PIGMENT CELLS IN CELL CULTURE. Dev Biol. 1963 Aug;8:99–127. doi: 10.1016/0012-1606(63)90028-9. [DOI] [PubMed] [Google Scholar]