Abstract
An organism identified as Pseudomonas putida was isolated from an enrichment culture with 2-furoic acid as its sole source of carbon and energy. The organism contained a 2-furoyl-coenzyme A (CoA) synthetase to form 2-furoyl-CoA and a 2-furoyl-CoA dehydrogenase to form 5-hydroxy-2-furoyl-CoA as the first two enzymes involved in the degradation. Tungstate, the specific antagonist of molybdate, decreased growth rate and consumption of 2-furoic acid but had no influence on growth with succinate. Correspondingly, the 2-furoyl-CoA dehydrogenase activity decreased when the organism was grown on 2-furoic acid in the presence of increasing amounts of tungstate. The addition of molybdate reversed the negative effect on 2-furoyl-CoA dehydrogenase activity, which points to the involvement of a molybdoenzyme in this reaction. Both enzymes studied were inducible. No plasmid was detected in this organism.
Full text
PDF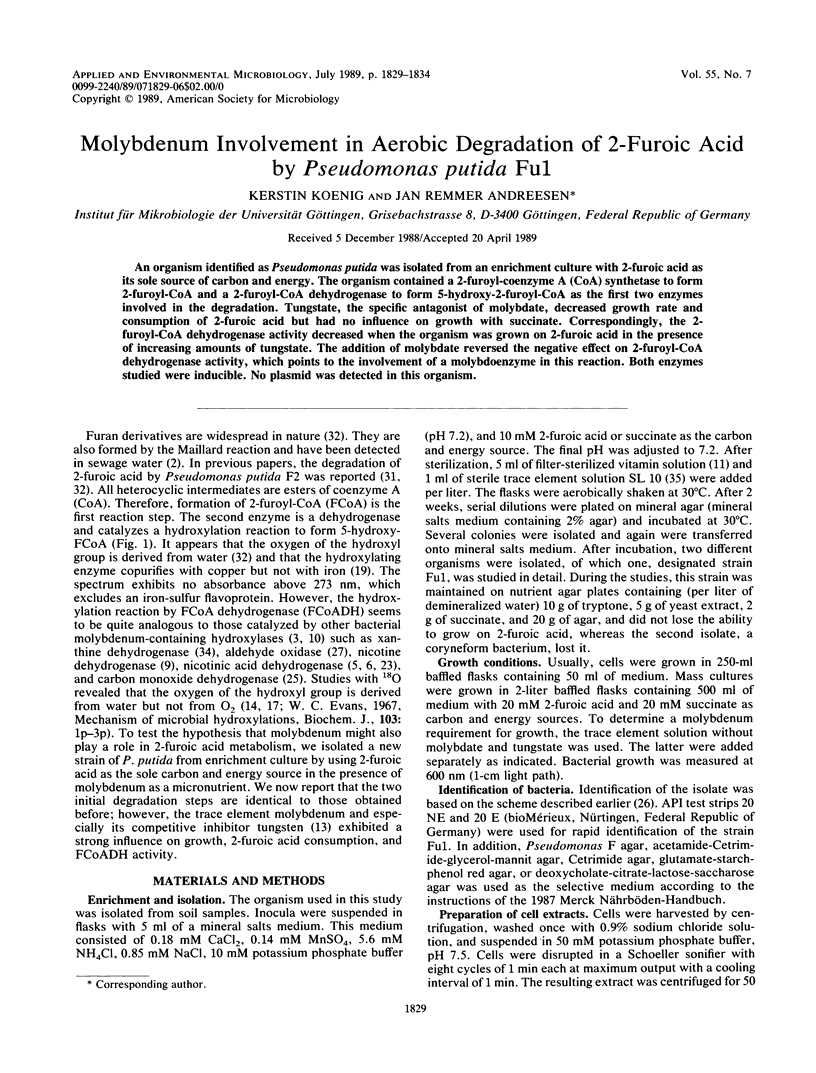
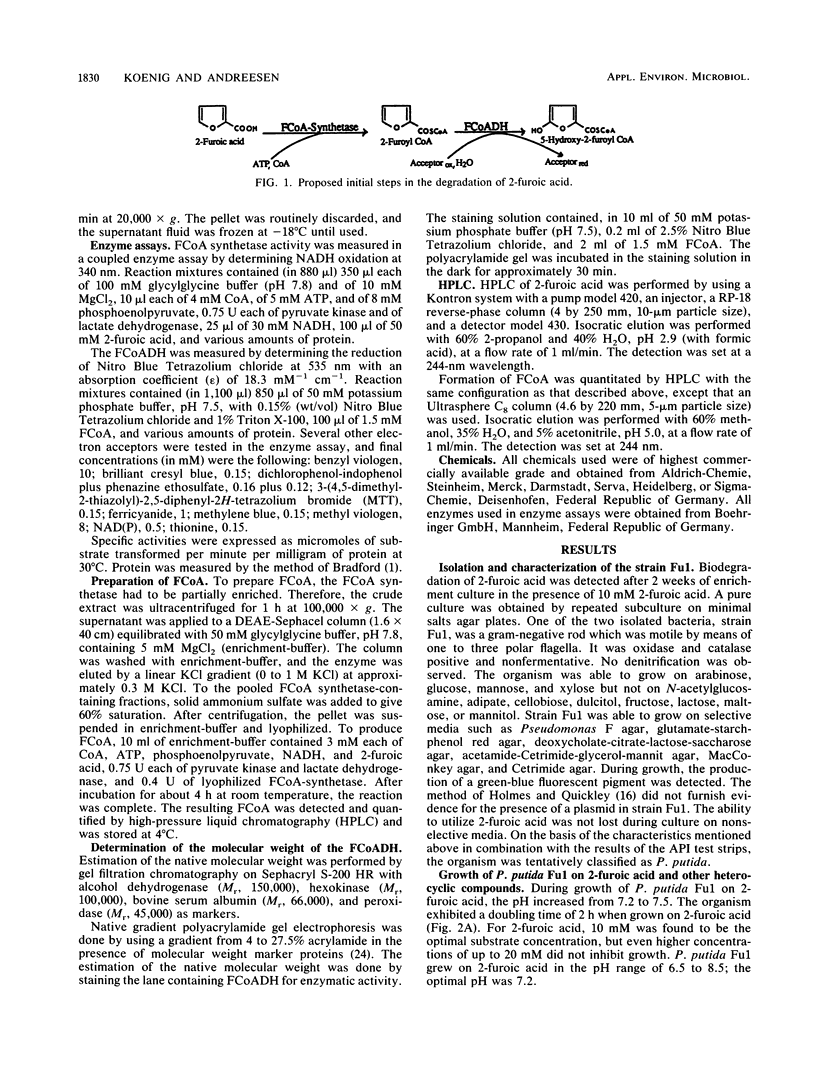
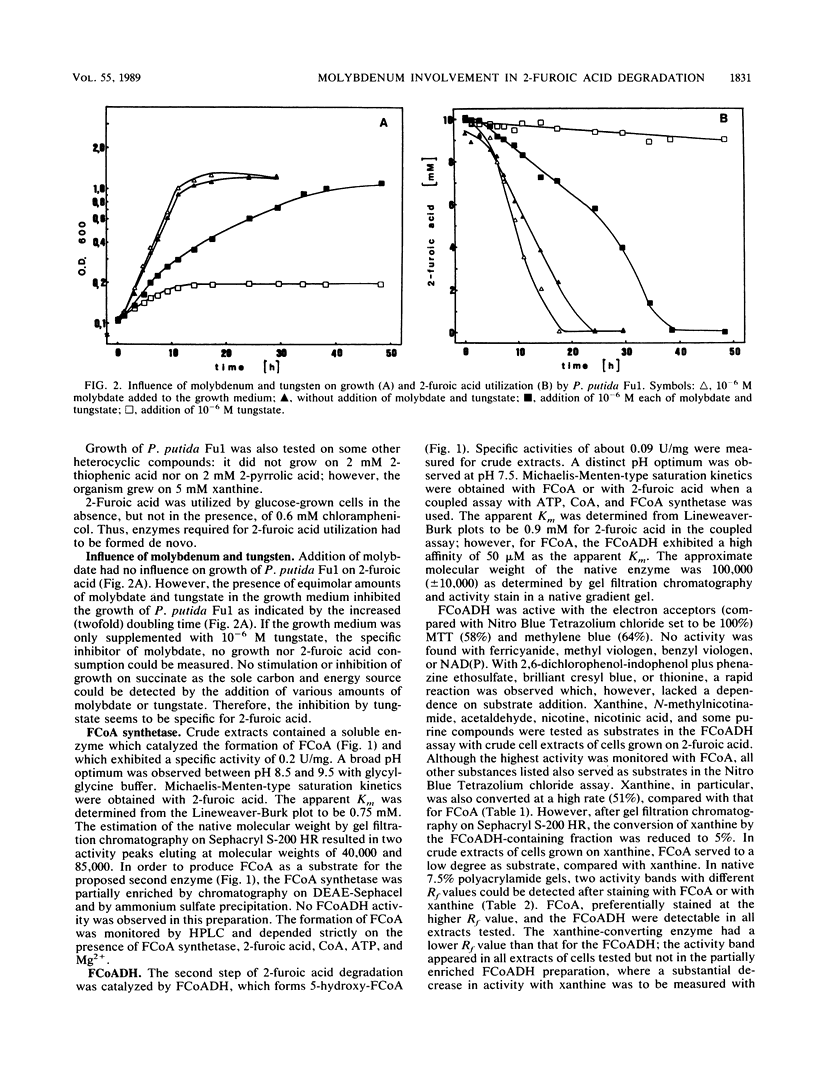
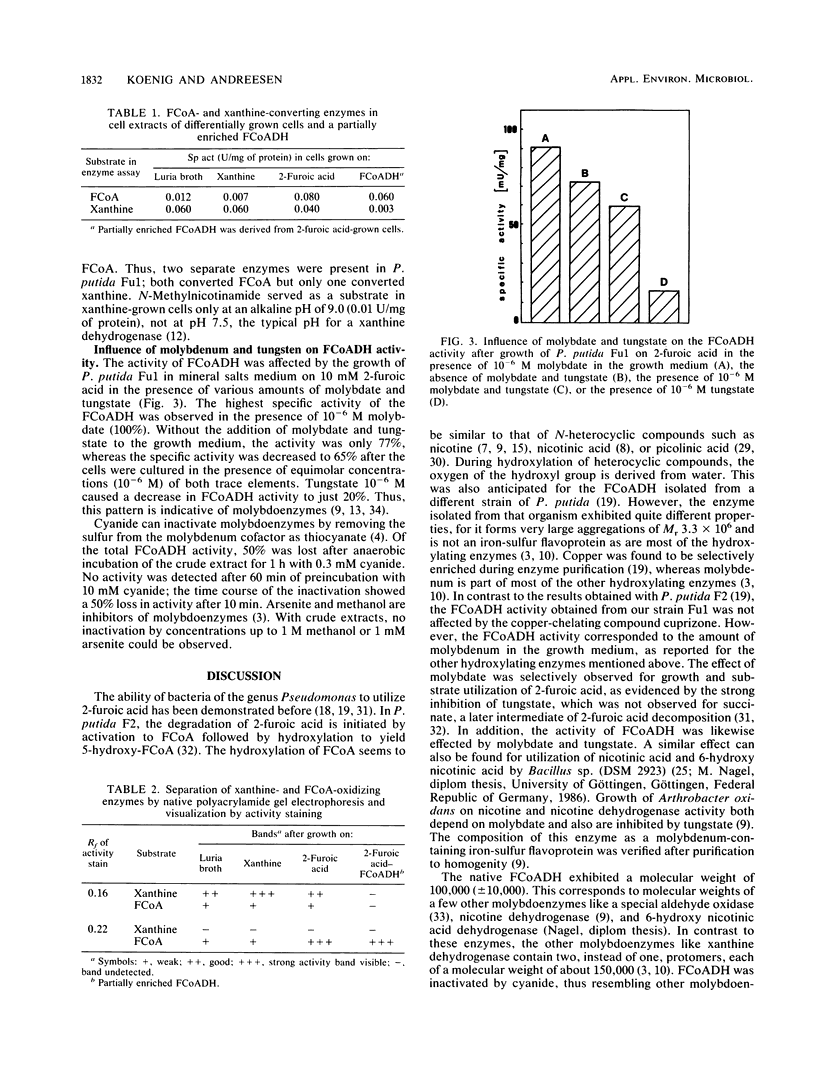
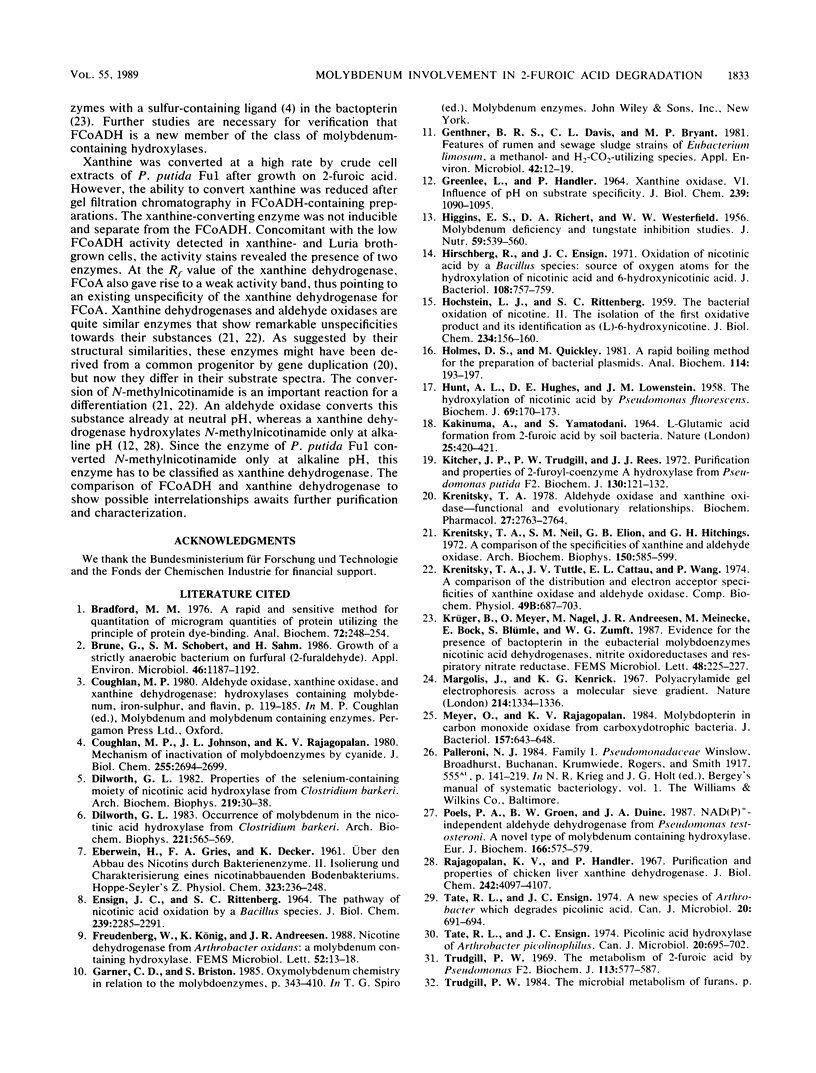
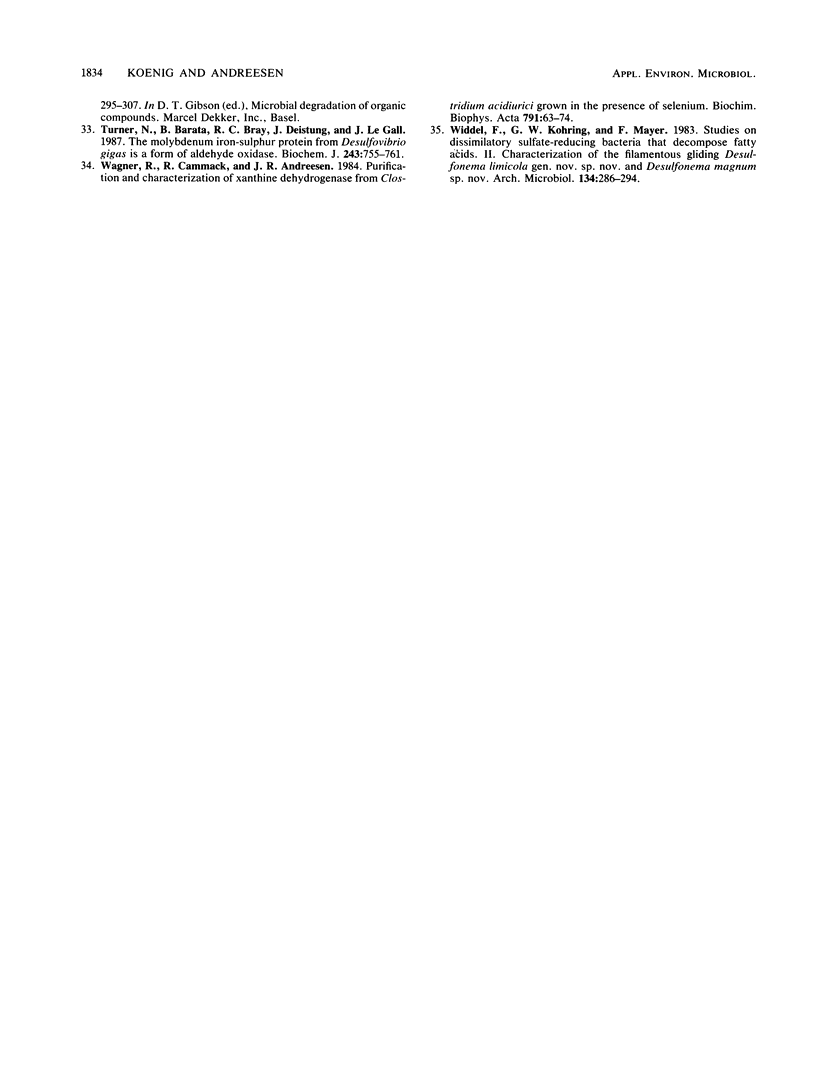
Selected References
These references are in PubMed. This may not be the complete list of references from this article.
- Bradford M. M. A rapid and sensitive method for the quantitation of microgram quantities of protein utilizing the principle of protein-dye binding. Anal Biochem. 1976 May 7;72:248–254. doi: 10.1006/abio.1976.9999. [DOI] [PubMed] [Google Scholar]
- Brune G., Schoberth S. M., Sahm H. Growth of a strictly anaerobic bacterium on furfural (2-furaldehyde). Appl Environ Microbiol. 1983 Nov;46(5):1187–1192. doi: 10.1128/aem.46.5.1187-1192.1983. [DOI] [PMC free article] [PubMed] [Google Scholar]
- Coughlan M. P., Johnson J. L., Rajagopalan K. V. Mechanisms of inactivation of molybdoenzymes by cyanide. J Biol Chem. 1980 Apr 10;255(7):2694–2699. [PubMed] [Google Scholar]
- Dilworth G. L. Occurrence of molybdenum in the nicotinic acid hydroxylase from Clostridium barkeri. Arch Biochem Biophys. 1983 Mar;221(2):565–569. doi: 10.1016/0003-9861(83)90176-5. [DOI] [PubMed] [Google Scholar]
- Dilworth G. L. Properties of the selenium-containing moiety of nicotinic acid hydroxylase from Clostridium barkeri. Arch Biochem Biophys. 1982 Nov;219(1):30–38. doi: 10.1016/0003-9861(82)90130-8. [DOI] [PubMed] [Google Scholar]
- EBERWEIN H., GRIES F. A., DECKER K. [On the decomposition of nicotine by bacterial enzymes. II. Isolation and characterization of a nicotine-splitting soil bacterium]. Hoppe Seylers Z Physiol Chem. 1961 May 3;323:236–248. doi: 10.1515/bchm2.1961.323.1.236. [DOI] [PubMed] [Google Scholar]
- ENSIGN J. C., RITTENBERG S. C. THE PATHWAY OF NICOTINIC ACID OXIDATION BY A BACILLUS SPECIES. J Biol Chem. 1964 Jul;239:2285–2291. [PubMed] [Google Scholar]
- GREENLEE L., HANDLER P. XANTHINE OXIDASE. VI. INFLUENCE OF PH ON SUBSTRATE SPECIFICITY. J Biol Chem. 1964 Apr;239:1090–1095. [PubMed] [Google Scholar]
- Genthner B. R., Davis C. L., Bryant M. P. Features of rumen and sewage sludge strains of Eubacterium limosum, a methanol- and H2-CO2-utilizing species. Appl Environ Microbiol. 1981 Jul;42(1):12–19. doi: 10.1128/aem.42.1.12-19.1981. [DOI] [PMC free article] [PubMed] [Google Scholar]
- HIGGINS E. S., RICHERT D. A., WESTERFELD W. W. Molybdenum deficiency and tungstate inhibition studies. J Nutr. 1956 Aug 10;59(4):539–559. doi: 10.1093/jn/59.4.539. [DOI] [PubMed] [Google Scholar]
- HOCHSTEIN L. I., RITTENBERG S. C. The bacterial oxidation of nicotine. II. The isolation of the first oxidative product and its identification as (1)-6-hydroxynicotine. J Biol Chem. 1959 Jan;234(1):156–160. [PubMed] [Google Scholar]
- HUNT A. L., HUGHES D. E., LOWENSTEIN J. M. The hydroxylation of nicotinic acid by Pseudomonas fluorescens. Biochem J. 1958 Jun;69(2):170–173. doi: 10.1042/bj0690170. [DOI] [PMC free article] [PubMed] [Google Scholar]
- Hirschberg R., Ensign J. C. Oxidation of nicotinic acid by a Bacillus species: source of oxygen atoms for the hydroxylation of nicotinic acid and 6-hydroxynicotinic acid. J Bacteriol. 1971 Nov;108(2):757–759. doi: 10.1128/jb.108.2.757-759.1971. [DOI] [PMC free article] [PubMed] [Google Scholar]
- Holmes D. S., Quigley M. A rapid boiling method for the preparation of bacterial plasmids. Anal Biochem. 1981 Jun;114(1):193–197. doi: 10.1016/0003-2697(81)90473-5. [DOI] [PubMed] [Google Scholar]
- KAKINUMA A., YAMATODANI S. L-GLUTAMIC ACID FORMATION FROM 2-FUROIC ACID BY SOIL BACTERIA. Nature. 1964 Jan 25;201:420–421. doi: 10.1038/201420a0. [DOI] [PubMed] [Google Scholar]
- Kitcher J. P., Trudgill P. W., Rees J. S. Purification and properties of 2-furoyl-coenzyme A hydroxylase from Pseudomonas putida F2. Biochem J. 1972 Nov;130(1):121–132. doi: 10.1042/bj1300121. [DOI] [PMC free article] [PubMed] [Google Scholar]
- Krenitsky T. A. Aldehyde oxidase and xanthine oxidase--functional and evolutionary relationships. Biochem Pharmacol. 1978;27(24):2763–2764. doi: 10.1016/0006-2952(78)90186-7. [DOI] [PubMed] [Google Scholar]
- Krenitsky T. A., Neil S. M., Elion G. B., Hitchings G. H. A comparison of the specificities of xanthine oxidase and aldehyde oxidase. Arch Biochem Biophys. 1972 Jun;150(2):585–599. doi: 10.1016/0003-9861(72)90078-1. [DOI] [PubMed] [Google Scholar]
- Krenitsky T. A., Tuttle J. V., Cattau E. L., Jr, Wang P. A comparison of the distribution and electron acceptor specificities of xanthine oxidase and aldehyde oxidase. Comp Biochem Physiol B. 1974 Dec 15;49(4):687–703. doi: 10.1016/0305-0491(74)90256-9. [DOI] [PubMed] [Google Scholar]
- Margolis J., Kenrick K. C. Polyacrylamide gel-electrophoresis across a molecular sieve gradient. Nature. 1967 Jun 24;214(5095):1334–1336. doi: 10.1038/2141334a0. [DOI] [PubMed] [Google Scholar]
- Meyer O., Rajagopalan K. V. Molybdopterin in carbon monoxide oxidase from carboxydotrophic bacteria. J Bacteriol. 1984 Feb;157(2):643–648. doi: 10.1128/jb.157.2.643-648.1984. [DOI] [PMC free article] [PubMed] [Google Scholar]
- Poels P. A., Groen B. W., Duine J. A. NAD(P)+-independent aldehyde dehydrogenase from Pseudomonas testosteroni. A novel type of molybdenum-containing hydroxylase. Eur J Biochem. 1987 Aug 3;166(3):575–579. doi: 10.1111/j.1432-1033.1987.tb13552.x. [DOI] [PubMed] [Google Scholar]
- Rajagopalan K. V., Handler P. Purification and properties of chicken liver xanthine dehydrogenase. J Biol Chem. 1967 Sep 25;242(18):4097–4107. [PubMed] [Google Scholar]
- Tate R. L., Ensign J. C. A new species of Arthrobacter which degrades picolinic acid. Can J Microbiol. 1974 May;20(5):691–694. doi: 10.1139/m74-105. [DOI] [PubMed] [Google Scholar]
- Tate R. L., Ensign J. C. Picolinic acid hydroxylase of Arthrobacter picolinophilus. Can J Microbiol. 1974 May;20(5):695–702. doi: 10.1139/m74-106. [DOI] [PubMed] [Google Scholar]
- Trudgill P. W. The metabolism of 2-furoic acid by Pseudomanas F2. Biochem J. 1969 Jul;113(4):577–587. doi: 10.1042/bj1130577. [DOI] [PMC free article] [PubMed] [Google Scholar]
- Turner N., Barata B., Bray R. C., Deistung J., Le Gall J., Moura J. J. The molybdenum iron-sulphur protein from Desulfovibrio gigas as a form of aldehyde oxidase. Biochem J. 1987 May 1;243(3):755–761. doi: 10.1042/bj2430755. [DOI] [PMC free article] [PubMed] [Google Scholar]