Abstract
DNA, RNA, and protein concentrations from starved ANT-300 cell populations grown at different growth rates fluctuated corresponding to the three stages of starvation-survival on total and viable cell bases. During stage 1 of starvation-survival, two to three peaks in the concentration levels for all three macromolecules were characteristic. During stage 2, DNA per total cell dropped to between 4.2 and 8.3% of the original amount for all of the cell populations examined, and it stabilized throughout stage 3. The decrease in DNA per cell was also observed in electron micrographs of cellular DNA in unstarved compared with starved cells. The fluctuations of RNA and protein per total cell concentrations observed during stage 2 coincided in all cases, except for the cells from dilution rate (D) = 0.015 h−1. This ANT-300 cell population showed a decrease in RNA per total cell to only 29.2% and an increase in protein to 129.7% of the original amount after 98 days of starvation. During stage 3, DNA, RNA, and protein concentrations per total cell also stabilized to continuous levels. Cells from the faster-growth-rate cell populations of D = 0.170 h−1 and batch culture had elevated protein per total cell concentrations, which remained primarily residual during the starvation period. Starved cells from D = 0.015 h−1 had estimated nucleoid and cell volumes of 0.018 and 0.05 μm3, respectively, yielding a nucleoid volume/cell volume ratio of 0.40. We consider these data to indicate that slow-growth-rate cells are better adapted for starvation-survival than their faster-growth-rate counterparts.
Full text
PDF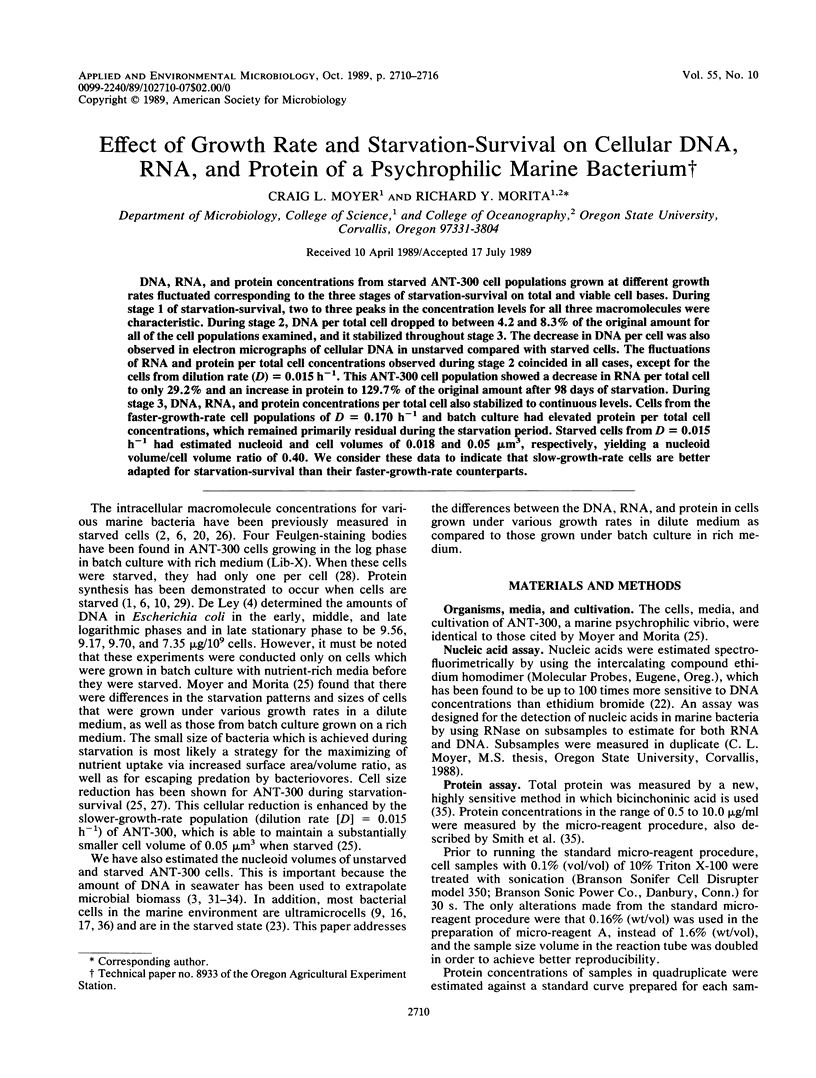
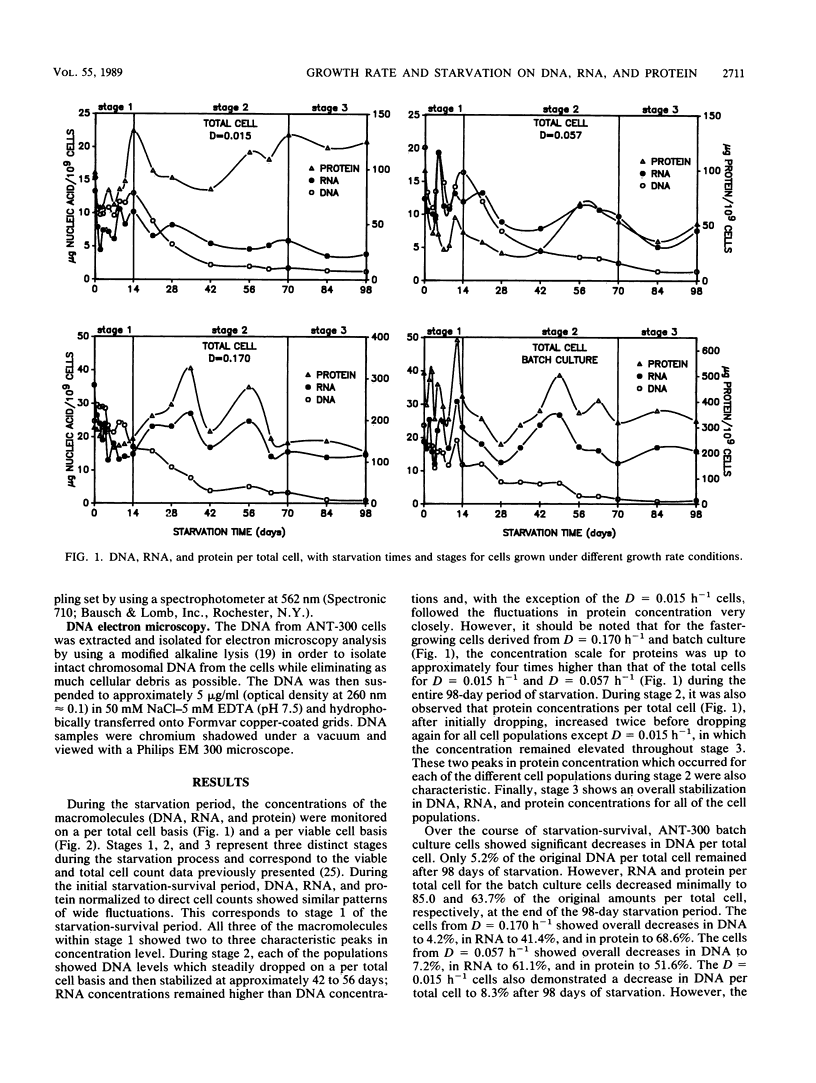
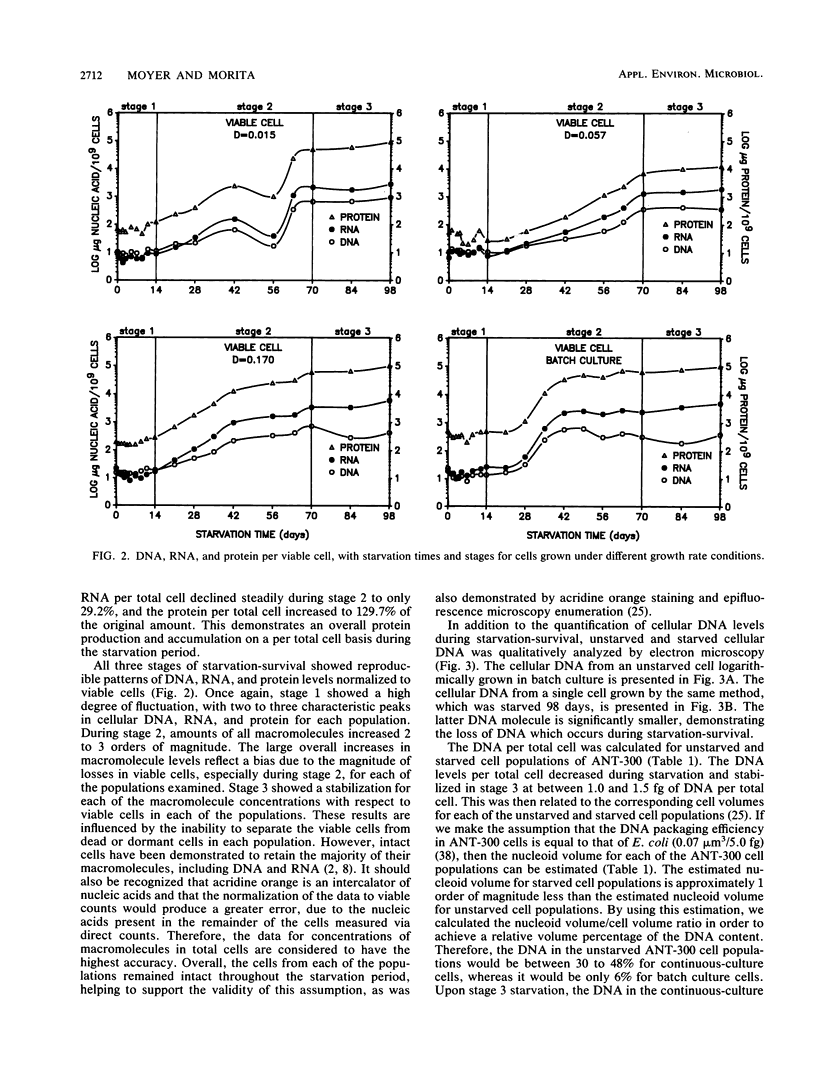
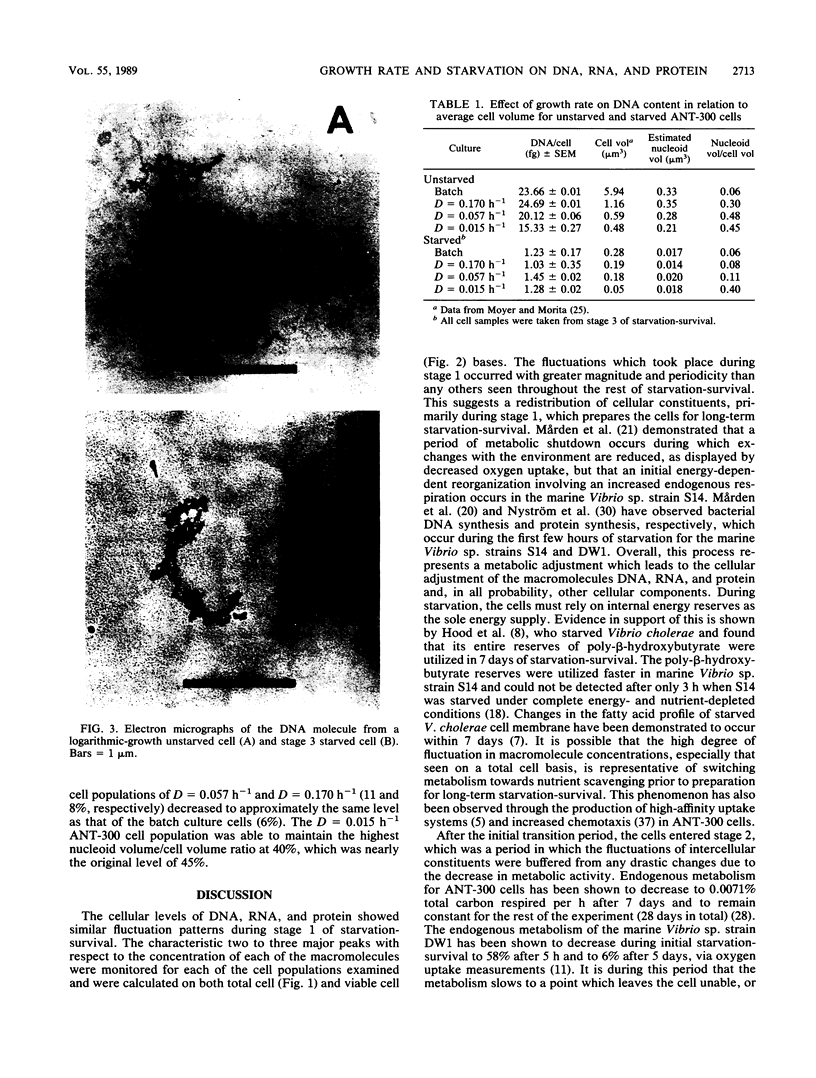
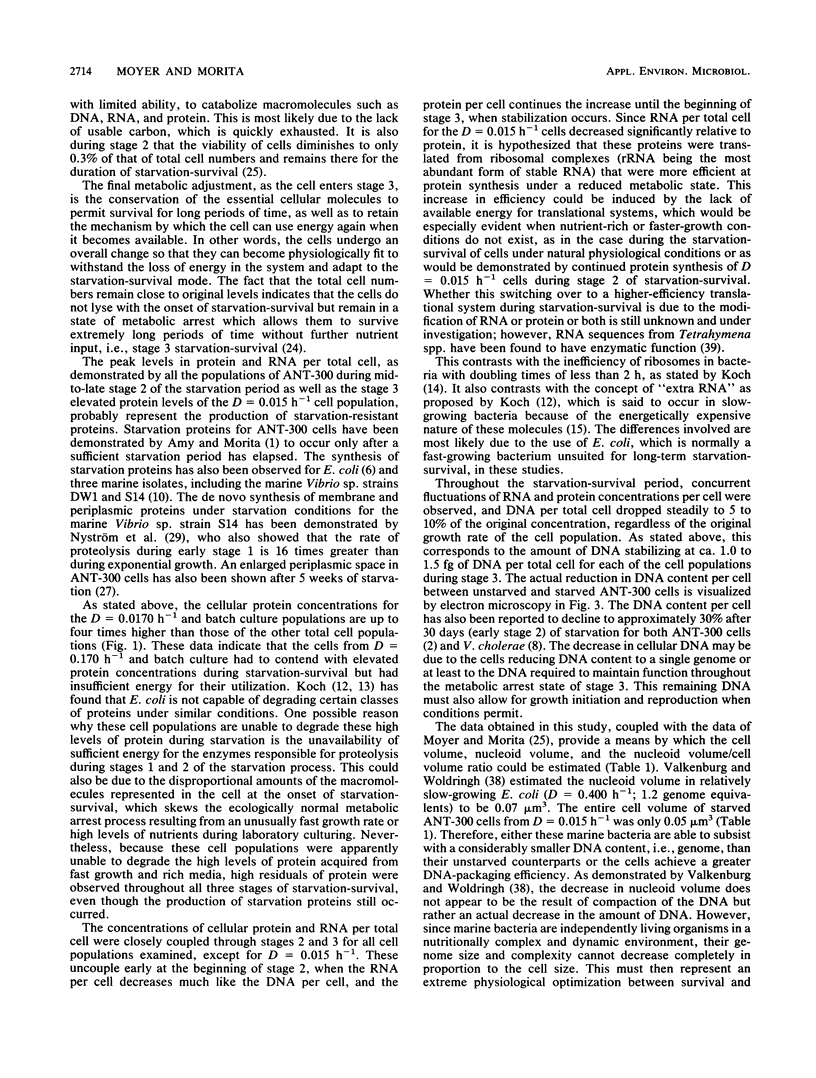
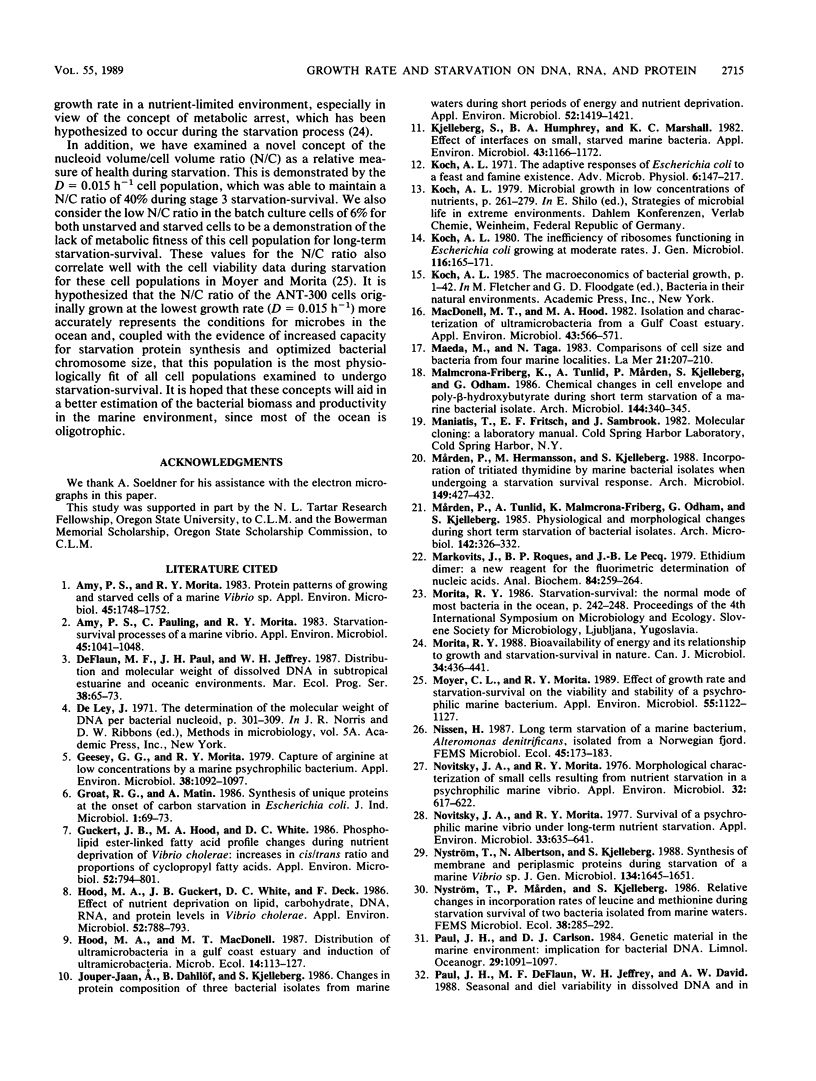
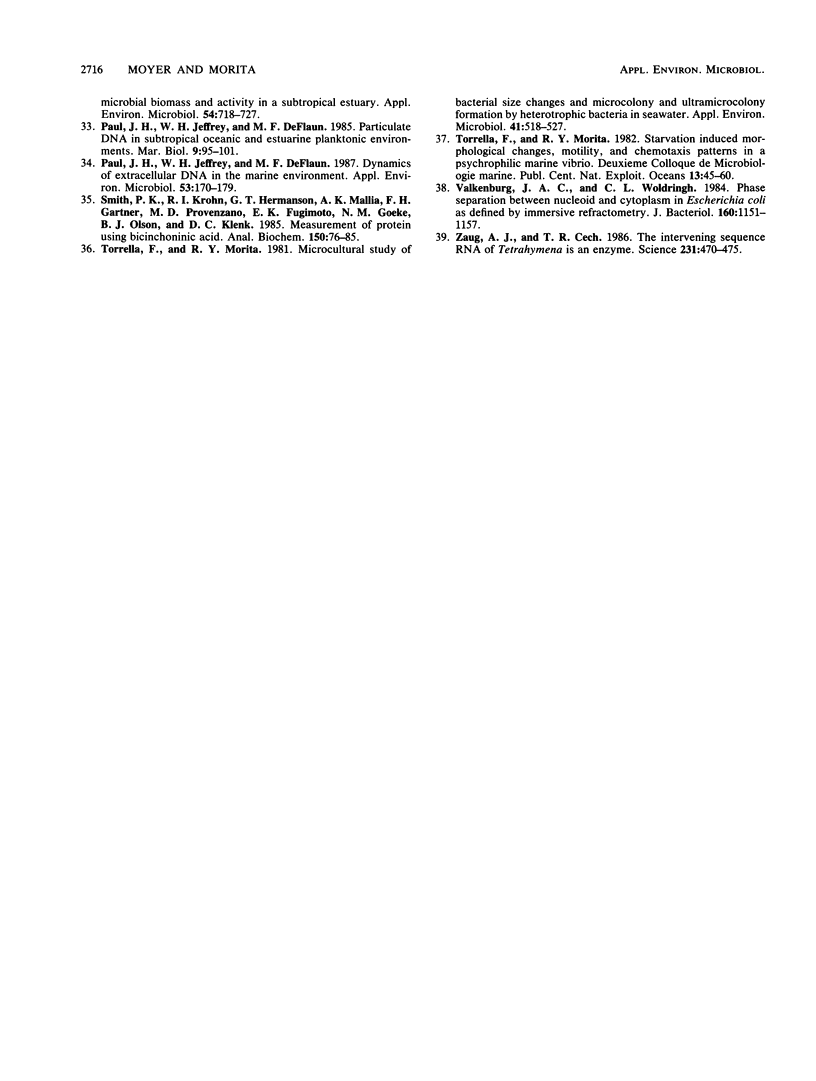
Images in this article
Selected References
These references are in PubMed. This may not be the complete list of references from this article.
- Amy P. S., Morita R. Y. Protein Patterns of Growing and Starved Cells of a Marine Vibrio sp. Appl Environ Microbiol. 1983 Jun;45(6):1748–1752. doi: 10.1128/aem.45.6.1748-1752.1983. [DOI] [PMC free article] [PubMed] [Google Scholar]
- Amy P. S., Pauling C., Morita R. Y. Starvation-survival processes of a marine Vibrio. Appl Environ Microbiol. 1983 Mar;45(3):1041–1048. doi: 10.1128/aem.45.3.1041-1048.1983. [DOI] [PMC free article] [PubMed] [Google Scholar]
- Geesey G. G., Morita R. Y. Capture of arginine at low concentrations by a marine psychrophilic bacterium. Appl Environ Microbiol. 1979 Dec;38(6):1092–1097. doi: 10.1128/aem.38.6.1092-1097.1979. [DOI] [PMC free article] [PubMed] [Google Scholar]
- Guckert J. B., Hood M. A., White D. C. Phospholipid ester-linked fatty acid profile changes during nutrient deprivation of Vibrio cholerae: increases in the trans/cis ratio and proportions of cyclopropyl fatty acids. Appl Environ Microbiol. 1986 Oct;52(4):794–801. doi: 10.1128/aem.52.4.794-801.1986. [DOI] [PMC free article] [PubMed] [Google Scholar]
- Hood M. A., Guckert J. B., White D. C., Deck F. Effect of nutrient deprivation on lipid, carbohydrate, DNA, RNA, and protein levels in Vibrio cholerae. Appl Environ Microbiol. 1986 Oct;52(4):788–793. doi: 10.1128/aem.52.4.788-793.1986. [DOI] [PMC free article] [PubMed] [Google Scholar]
- Jaan A. J., Dahllöf B., Kjelleberg S. Changes in Protein Composition of Three Bacterial Isolates from Marine Waters during Short Periods of Energy and Nutrient Deprivation. Appl Environ Microbiol. 1986 Dec;52(6):1419–1421. doi: 10.1128/aem.52.6.1419-1421.1986. [DOI] [PMC free article] [PubMed] [Google Scholar]
- Kjelleberg S., Humphrey B. A., Marshall K. C. Effect of interfaces on small, starved marine bacteria. Appl Environ Microbiol. 1982 May;43(5):1166–1172. doi: 10.1128/aem.43.5.1166-1172.1982. [DOI] [PMC free article] [PubMed] [Google Scholar]
- Koch A. L. The adaptive responses of Escherichia coli to a feast and famine existence. Adv Microb Physiol. 1971;6:147–217. doi: 10.1016/s0065-2911(08)60069-7. [DOI] [PubMed] [Google Scholar]
- Koch A. L. The inefficiency of ribosomes functioning in Escherichia coli growing at moderate rates. J Gen Microbiol. 1980 Jan;116(1):165–171. doi: 10.1099/00221287-116-1-165. [DOI] [PubMed] [Google Scholar]
- Macdonell M. T., Hood M. A. Isolation and characterization of ultramicrobacteria from a gulf coast estuary. Appl Environ Microbiol. 1982 Mar;43(3):566–571. doi: 10.1128/aem.43.3.566-571.1982. [DOI] [PMC free article] [PubMed] [Google Scholar]
- Markovits J., Roques B. P., Le Pecq J. B. Ethidium dimer: a new reagent for the fluorimetric determination of nucleic acids. Anal Biochem. 1979 Apr 15;94(2):259–264. doi: 10.1016/0003-2697(79)90357-9. [DOI] [PubMed] [Google Scholar]
- Moyer C. L., Morita R. Y. Effect of growth rate and starvation-survival on the viability and stability of a psychrophilic marine bacterium. Appl Environ Microbiol. 1989 May;55(5):1122–1127. doi: 10.1128/aem.55.5.1122-1127.1989. [DOI] [PMC free article] [PubMed] [Google Scholar]
- Novitsky J. A., Morita R. Y. Morphological characterization of small cells resulting from nutrient starvation of a psychrophilic marine vibrio. Appl Environ Microbiol. 1976 Oct;32(4):617–622. doi: 10.1128/aem.32.4.617-622.1976. [DOI] [PMC free article] [PubMed] [Google Scholar]
- Novitsky J. A., Morita R. Y. Survival of a psychrophilic marine Vibrio under long-term nutrient starvation. Appl Environ Microbiol. 1977 Mar;33(3):635–641. doi: 10.1128/aem.33.3.635-641.1977. [DOI] [PMC free article] [PubMed] [Google Scholar]
- Nyström T., Albertson N., Kjelleberg S. Synthesis of membrane and periplasmic proteins during starvation of a marine Vibrio sp. J Gen Microbiol. 1988 Jun;134(6):1645–1651. doi: 10.1099/00221287-134-6-1645. [DOI] [PubMed] [Google Scholar]
- Paul J. H., Jeffrey W. H., DeFlaun M. F. Dynamics of extracellular DNA in the marine environment. Appl Environ Microbiol. 1987 Jan;53(1):170–179. doi: 10.1128/aem.53.1.170-179.1987. [DOI] [PMC free article] [PubMed] [Google Scholar]
- Paul John H., Deflaun Mary F., Jeffrey Wade H., David Andrew W. Seasonal and Diel Variability in Dissolved DNA and in Microbial Biomass and Activity in a Subtropical Estuary. Appl Environ Microbiol. 1988 Mar;54(3):718–727. doi: 10.1128/aem.54.3.718-727.1988. [DOI] [PMC free article] [PubMed] [Google Scholar]
- Smith P. K., Krohn R. I., Hermanson G. T., Mallia A. K., Gartner F. H., Provenzano M. D., Fujimoto E. K., Goeke N. M., Olson B. J., Klenk D. C. Measurement of protein using bicinchoninic acid. Anal Biochem. 1985 Oct;150(1):76–85. doi: 10.1016/0003-2697(85)90442-7. [DOI] [PubMed] [Google Scholar]
- Torrella F., Morita R. Y. Microcultural study of bacterial size changes and microcolony and ultramicrocolony formation by heterotrophic bacteria in seawater. Appl Environ Microbiol. 1981 Feb;41(2):518–527. doi: 10.1128/aem.41.2.518-527.1981. [DOI] [PMC free article] [PubMed] [Google Scholar]
- Valkenburg J. A., Woldringh C. L. Phase separation between nucleoid and cytoplasm in Escherichia coli as defined by immersive refractometry. J Bacteriol. 1984 Dec;160(3):1151–1157. doi: 10.1128/jb.160.3.1151-1157.1984. [DOI] [PMC free article] [PubMed] [Google Scholar]
- Zaug A. J., Cech T. R. The intervening sequence RNA of Tetrahymena is an enzyme. Science. 1986 Jan 31;231(4737):470–475. doi: 10.1126/science.3941911. [DOI] [PubMed] [Google Scholar]