Abstract
Bacteroides ruminicola B(1)4, a predominant ruminal and cecal bacterium, was grown in batch and continuous cultures, and beta-glucosidase activity was measured by following the hydrolysis of p-nitrophenyl-beta-glucopyranoside. Specific activity was high when the bacterium was grown in batch cultures containing cellobiose, mannose, or lactose (greater than 286 U/g of protein). Activity was reduced approximately 90% when the organism was grown on glucose, sucrose, fructose, maltose, or arabinose. The specific activity of cells fermenting glucose was initially low but increased as glucose was depleted. When glucose was added to cultures growing on cellobiose, beta-glucosidase synthesis ceased immediately. Catabolite repression by glucose was not accompanied by diauxic growth and was not relieved by cyclic AMP. Since glucose-grown cultures eventually exhibited high beta-glucosidase activity, cellobiose was not needed as an inducer. Catabolite repression explained beta-glucosidase activity of batch cultures and high-dilution-rate chemostats where glucose accumulated, but it could not account for activity at slow dilution rates. Maximal beta-glucosidase activity was observed at a dilution rate of approximately 0.35 h-1, and cellobiose-limited chemostats showed a 15-fold decrease in activity as the dilution rate declined. An eightfold decline was observed in glucose-limited chemostats. Since inducer availability was not a confounding factor in glucose-limited chemostats, the growth rate-dependent derepression could not be explained by other mechanisms.
Full text
PDF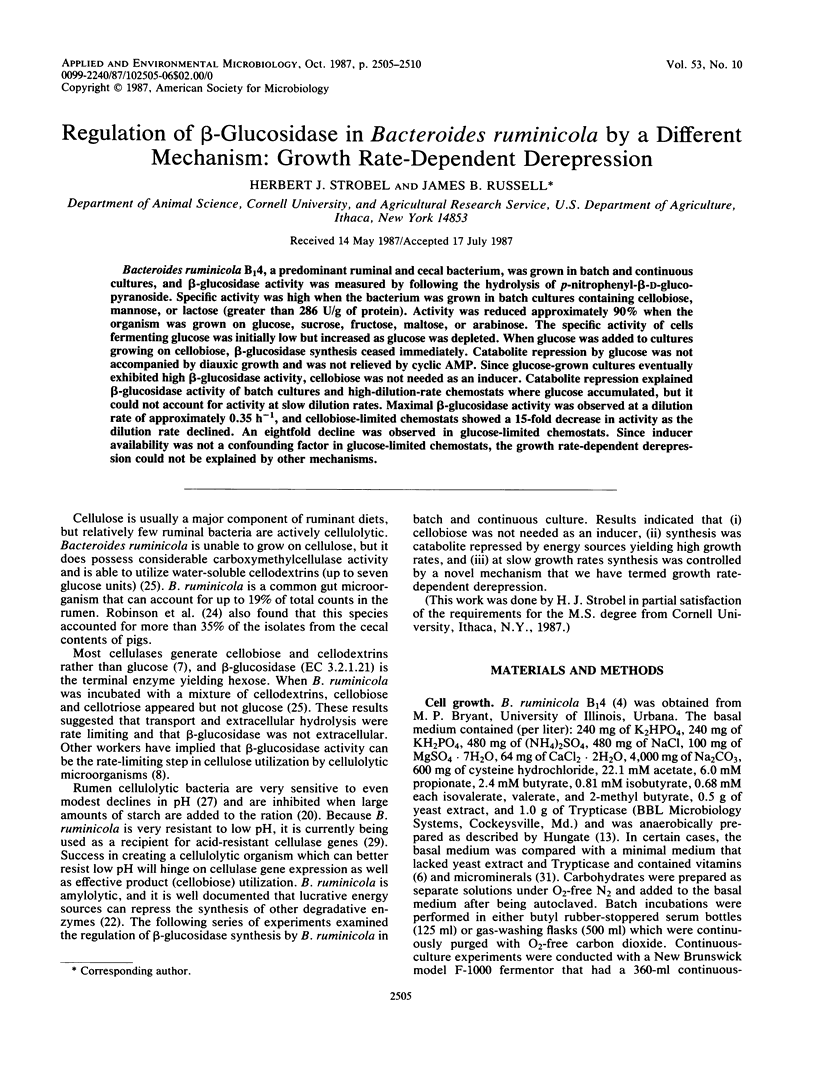
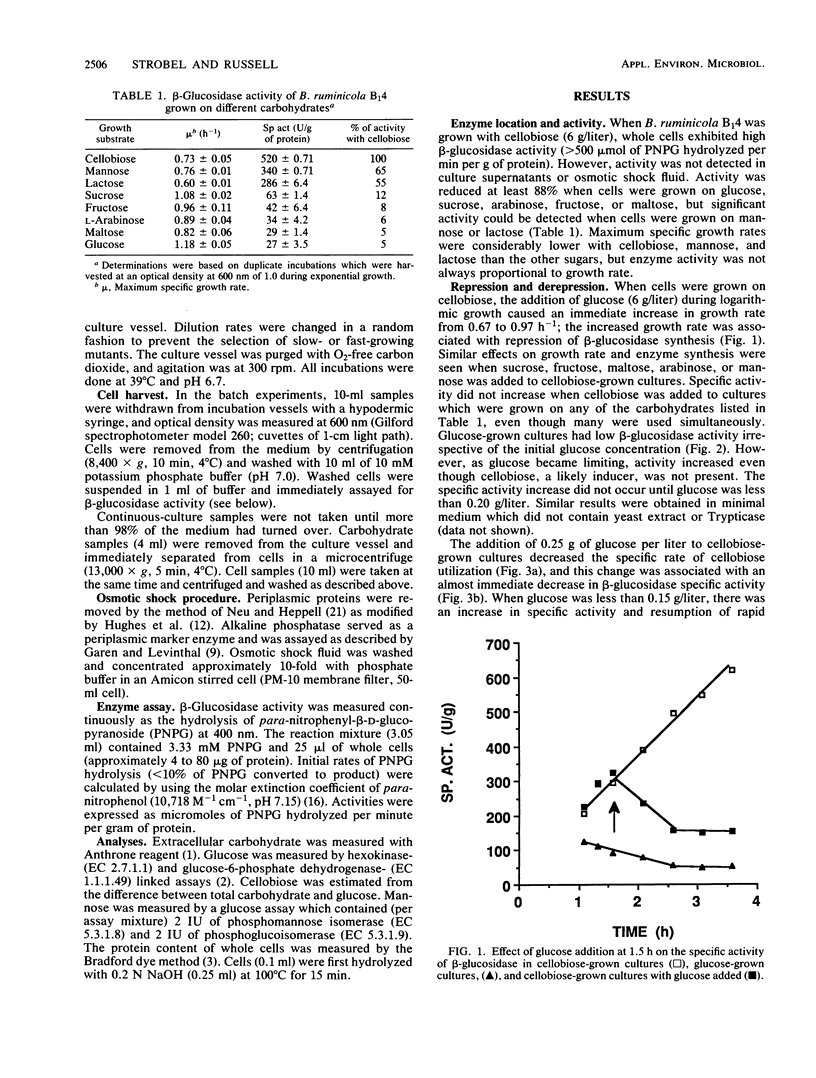
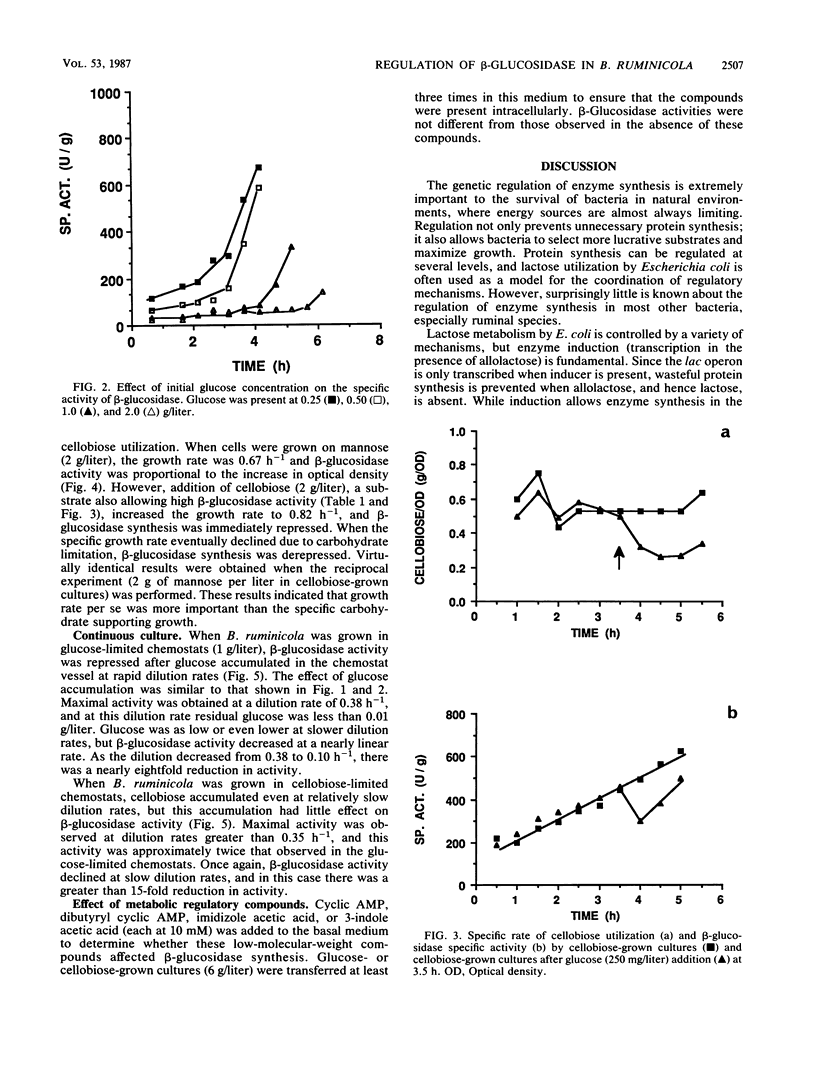
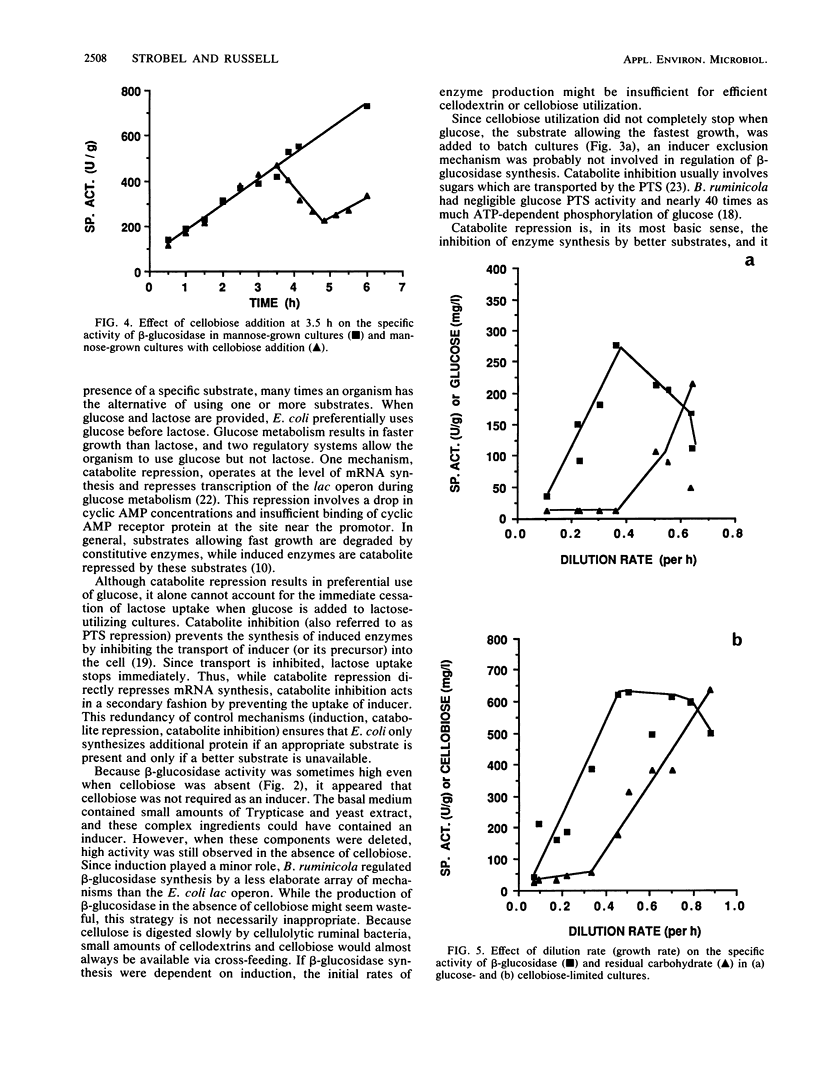
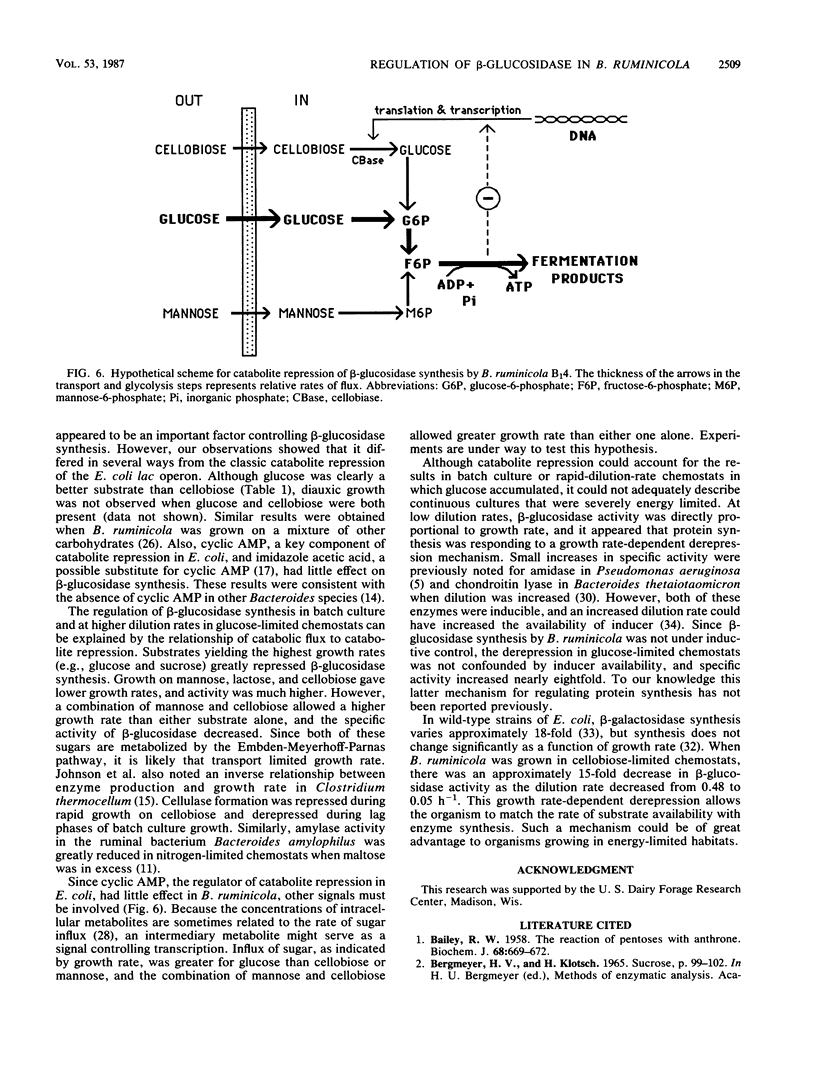
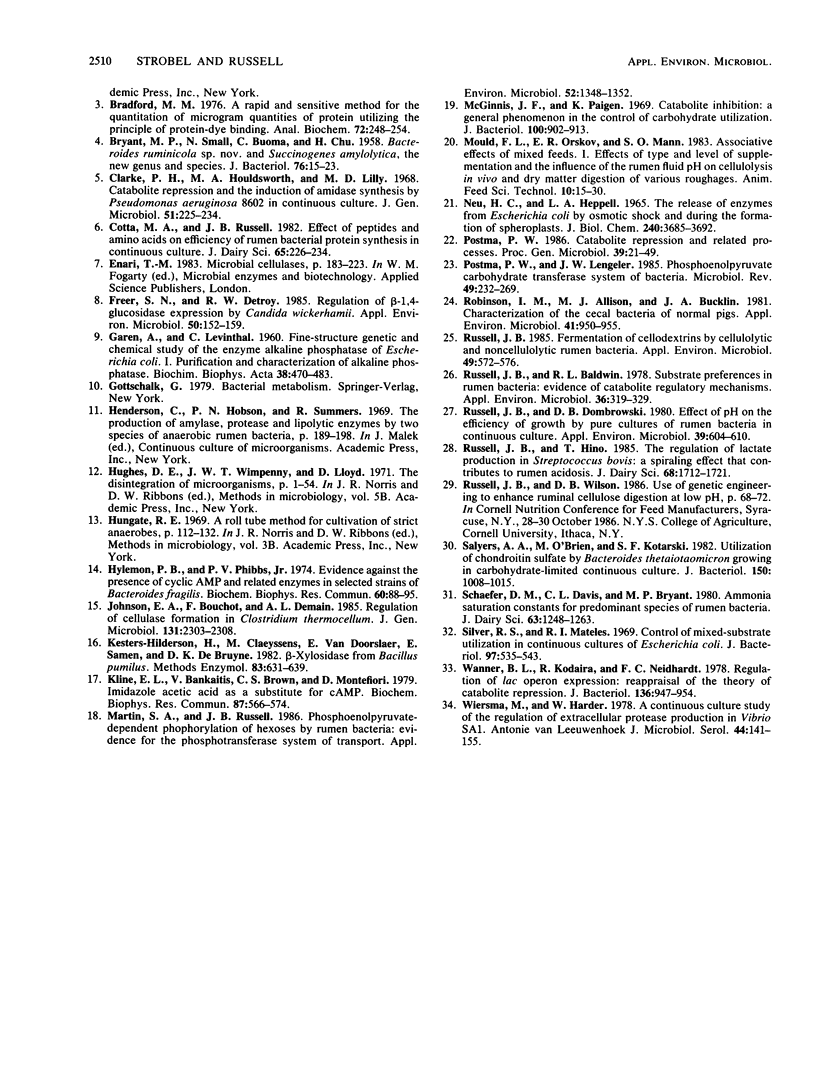
Selected References
These references are in PubMed. This may not be the complete list of references from this article.
- BAILEY R. W. The reaction of pentoses with anthrone. Biochem J. 1958 Apr;68(4):669–672. doi: 10.1042/bj0680669. [DOI] [PMC free article] [PubMed] [Google Scholar]
- BRYANT M. P., SMALL N., BOUMA C., CHU H. Bacteroides ruminicola n. sp. and Succinimonas amylolytica; the new genus and species; species of succinic acid-producing anaerobic bacteria of the bovine rumen. J Bacteriol. 1958 Jul;76(1):15–23. doi: 10.1128/jb.76.1.15-23.1958. [DOI] [PMC free article] [PubMed] [Google Scholar]
- Bradford M. M. A rapid and sensitive method for the quantitation of microgram quantities of protein utilizing the principle of protein-dye binding. Anal Biochem. 1976 May 7;72:248–254. doi: 10.1016/0003-2697(76)90527-3. [DOI] [PubMed] [Google Scholar]
- Clarke P. H., Houldsworth M. A., Lilly M. D. Catabolite repression and the induction of amidase synthesis by Pseudomonas aeruginosa 8602 in continuous culture. J Gen Microbiol. 1968 Apr;51(2):225–234. doi: 10.1099/00221287-51-2-225. [DOI] [PubMed] [Google Scholar]
- Freer S. N., Detroy R. W. Regulation of beta-1, 4-Glucosidase Expression by Candida wickerhamii. Appl Environ Microbiol. 1985 Jul;50(1):152–159. doi: 10.1128/aem.50.1.152-159.1985. [DOI] [PMC free article] [PubMed] [Google Scholar]
- GAREN A., LEVINTHAL C. A fine-structure genetic and chemical study of the enzyme alkaline phosphatase of E. coli. I. Purification and characterization of alkaline phosphatase. Biochim Biophys Acta. 1960 Mar 11;38:470–483. doi: 10.1016/0006-3002(60)91282-8. [DOI] [PubMed] [Google Scholar]
- Hylemon P. B., Phibbs P. V., Jr Evidence against the presence of cyclic AMP and related enzymes in selected strains of Bacteroides fragilis. Biochem Biophys Res Commun. 1974 Sep 9;60(1):88–95. doi: 10.1016/0006-291x(74)90176-4. [DOI] [PubMed] [Google Scholar]
- Kersters-Hilderson H., Claeyssens M., Van Doorslaer E., Saman E., De Bruyne C. K. beta-D-xylosidase from Bacillus pumilus. Methods Enzymol. 1982;83:631–639. doi: 10.1016/0076-6879(82)83062-0. [DOI] [PubMed] [Google Scholar]
- Kline E. L., Bankaitis V., Brown C. S., Montefiori D. Imidazole acetic acid as a substitute for cAMP. Biochem Biophys Res Commun. 1979 Mar 30;87(2):566–574. doi: 10.1016/0006-291x(79)91832-1. [DOI] [PubMed] [Google Scholar]
- Martin S. A., Russell J. B. Phosphoenolpyruvate-dependent phosphorylation of hexoses by ruminal bacteria: evidence for the phosphotransferase transport system. Appl Environ Microbiol. 1986 Dec;52(6):1348–1352. doi: 10.1128/aem.52.6.1348-1352.1986. [DOI] [PMC free article] [PubMed] [Google Scholar]
- McGinnis J. F., Paigen K. Catabolite inhibition: a general phenomenon in the control of carbohydrate utilization. J Bacteriol. 1969 Nov;100(2):902–913. doi: 10.1128/jb.100.2.902-913.1969. [DOI] [PMC free article] [PubMed] [Google Scholar]
- Neu H. C., Heppel L. A. The release of enzymes from Escherichia coli by osmotic shock and during the formation of spheroplasts. J Biol Chem. 1965 Sep;240(9):3685–3692. [PubMed] [Google Scholar]
- Postma P. W., Lengeler J. W. Phosphoenolpyruvate:carbohydrate phosphotransferase system of bacteria. Microbiol Rev. 1985 Sep;49(3):232–269. doi: 10.1128/mr.49.3.232-269.1985. [DOI] [PMC free article] [PubMed] [Google Scholar]
- Robinson I. M., Allison M. J., Bucklin J. A. Characterization of the cecal bacteria of normal pigs. Appl Environ Microbiol. 1981 Apr;41(4):950–955. doi: 10.1128/aem.41.4.950-955.1981. [DOI] [PMC free article] [PubMed] [Google Scholar]
- Russell J. B., Baldwin R. L. Substrate preferences in rumen bacteria: evidence of catabolite regulatory mechanisms. Appl Environ Microbiol. 1978 Aug;36(2):319–329. doi: 10.1128/aem.36.2.319-329.1978. [DOI] [PMC free article] [PubMed] [Google Scholar]
- Russell J. B., Dombrowski D. B. Effect of pH on the efficiency of growth by pure cultures of rumen bacteria in continuous culture. Appl Environ Microbiol. 1980 Mar;39(3):604–610. doi: 10.1128/aem.39.3.604-610.1980. [DOI] [PMC free article] [PubMed] [Google Scholar]
- Russell J. B. Fermentation of cellodextrins by cellulolytic and noncellulolytic rumen bacteria. Appl Environ Microbiol. 1985 Mar;49(3):572–576. doi: 10.1128/aem.49.3.572-576.1985. [DOI] [PMC free article] [PubMed] [Google Scholar]
- Salyers A. A., O'Brien M., Kotarski S. F. Utilization of chondroitin sulfate by Bacteroides thetaiotaomicron growing in carbohydrate-limited continuous culture. J Bacteriol. 1982 Jun;150(3):1008–1015. doi: 10.1128/jb.150.3.1008-1015.1982. [DOI] [PMC free article] [PubMed] [Google Scholar]
- Schaefer D. M., Davis C. L., Bryant M. P. Ammonia saturation constants for predominant species of rumen bacteria. J Dairy Sci. 1980 Aug;63(8):1248–1263. doi: 10.3168/jds.S0022-0302(80)83076-1. [DOI] [PubMed] [Google Scholar]
- Silver R. S., Mateles R. I. Control of mixed-substrate utilization in continuous cultures of Escherichia coli. J Bacteriol. 1969 Feb;97(2):535–543. doi: 10.1128/jb.97.2.535-543.1969. [DOI] [PMC free article] [PubMed] [Google Scholar]
- Wanner B. L., Kodaira R., Neidhardt F. C. Regulation of lac operon expression: reappraisal of the theory of catabolite repression. J Bacteriol. 1978 Dec;136(3):947–954. doi: 10.1128/jb.136.3.947-954.1978. [DOI] [PMC free article] [PubMed] [Google Scholar]
- Wiersma M., Harder W. A continuous culture study of the regulation of extracellular protease production in Vibrio SA1. Antonie Van Leeuwenhoek. 1978;44(2):141–155. doi: 10.1007/BF00643217. [DOI] [PubMed] [Google Scholar]