Abstract
A mixed culture of human fecal bacteria was grown for 120 days in a three-stage continuous culture system. To reproduce some of the nutritional and pH characteristics of the large gut, each vessel had a different operating volume (0.3, 0.5, and 0.8 liter) and pH (6.0, 6.5, and 7.0). A mixture of polysaccharides and proteins was used as carbon and nitrogen sources. Measurements of H2, CH4, S2-, sulfate reduction rates, sulfate-reducing bacteria (SRB), and volatile fatty acids were made throughout the experiment. After 48 days of running, porcine gastric mucin (5.8 g/day) was independently fed to vessel 1 of the multichamber system. The mucin was extensively degraded as evidenced by the stimulation of volatile fatty acid production. In the absence of mucin, sulfate-reducing activity was comparatively insignificant and methanogenesis was the major route for the disposal of electrons. The reverse occurred upon the addition of mucin; sulfate reduction predominated and methanogenesis was completely inhibited. This was attributed to release of sulfate from the mucin which enabled SRB to outcompete methanogenic bacteria for H2. SRB stimulated by mucin were acetate-utilizing Desulfobacter spp., lactate- and H2-utilizing Desulfovibrio spp., and propionate-utilizing Desulfobulbus spp. When the mucin pump was switched off, the multichamber system reverted to a state close to its original equilibrium. These data provide further evidence that sulfated polysaccharides such as mucin may be a source of sulfate for SRB in the human large gut.
Full text
PDF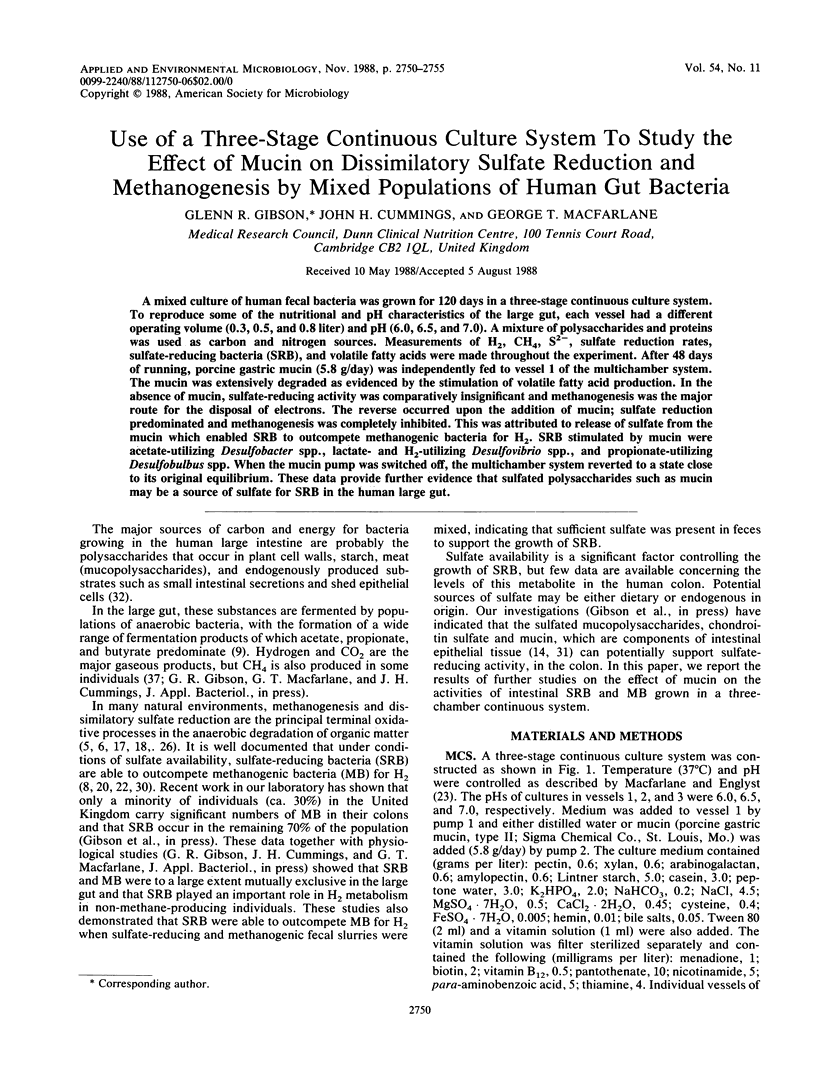
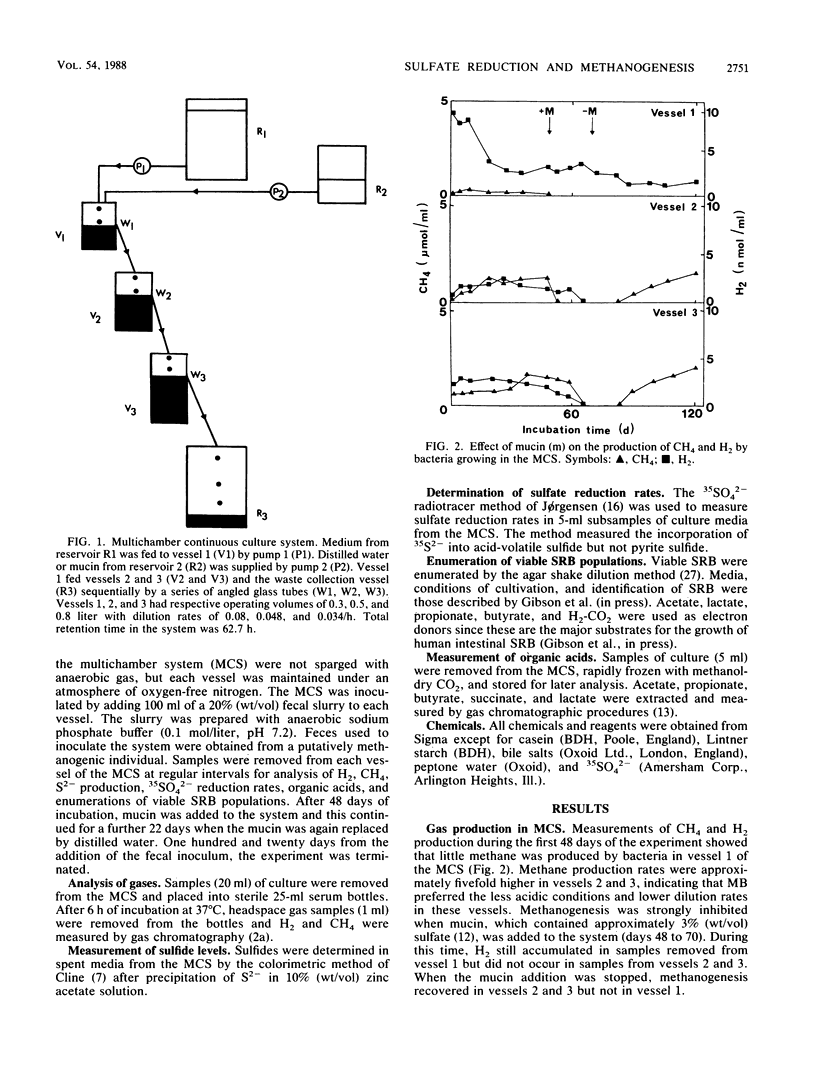
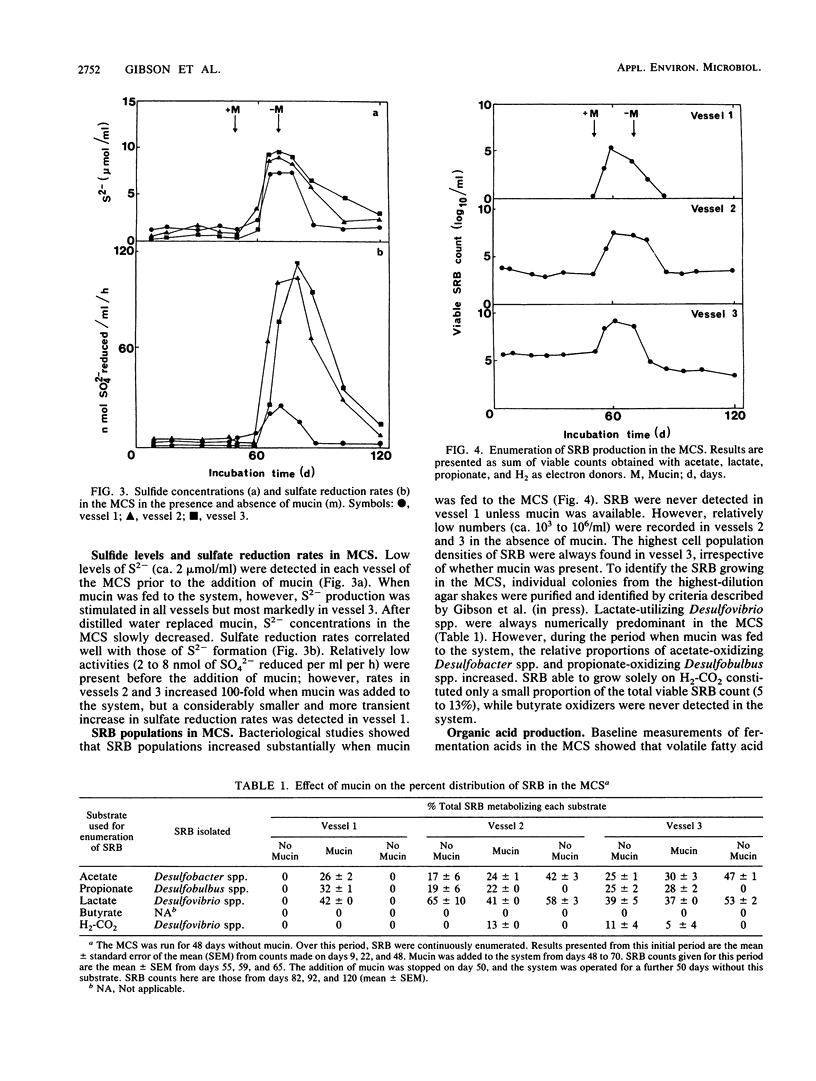
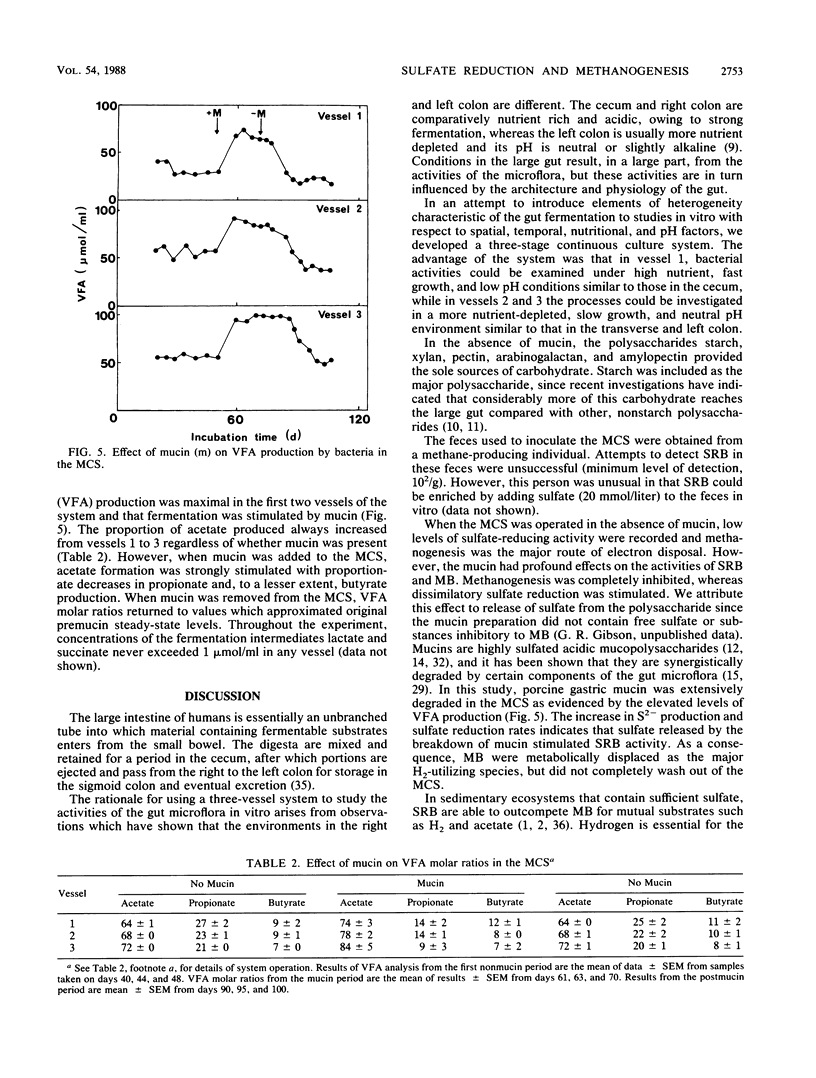
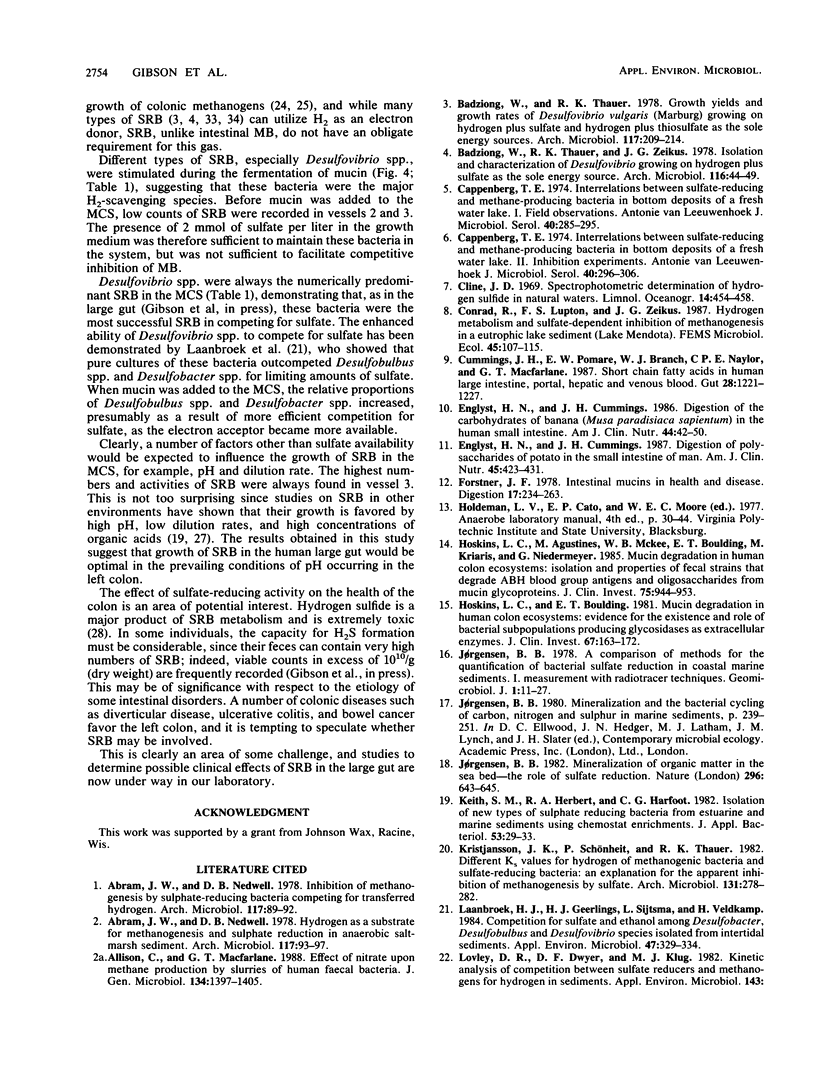
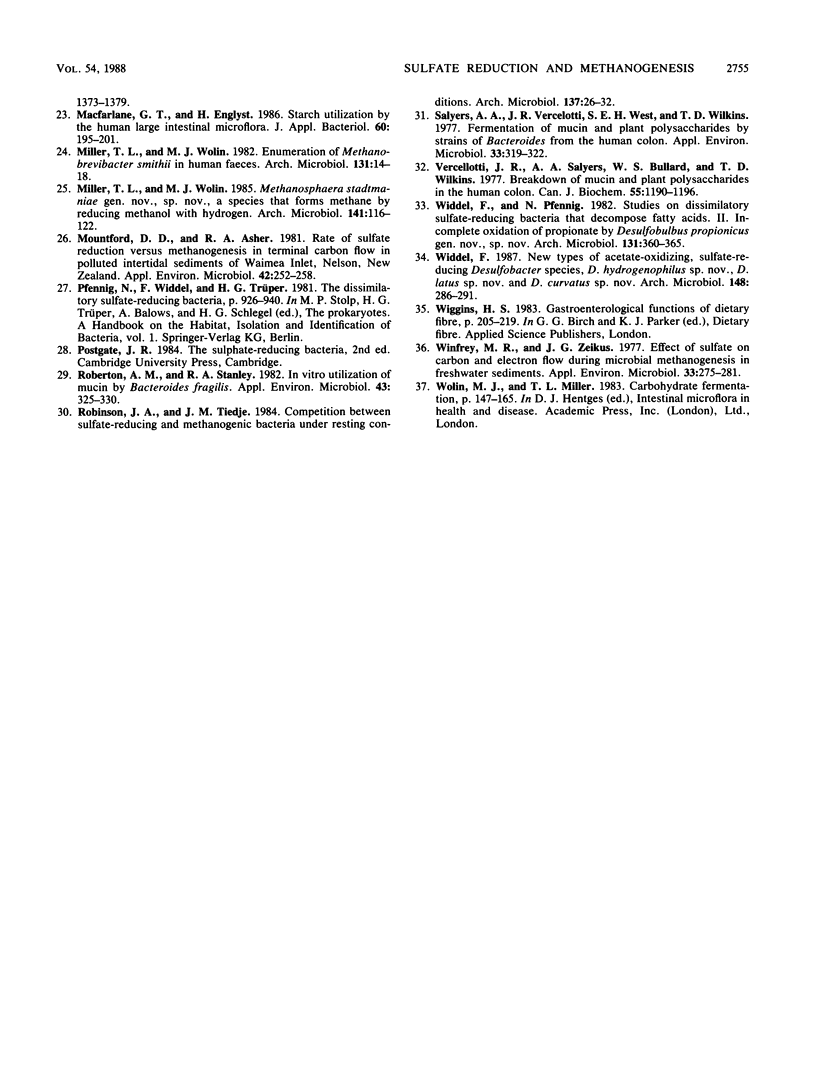
Selected References
These references are in PubMed. This may not be the complete list of references from this article.
- Abram J. W., Nedwell D. B. Hydrogen as a substrate for methanogenesis and sulphate reduction in anaerobic saltmarsh sediment. Arch Microbiol. 1978 Apr 27;117(1):93–97. doi: 10.1007/BF00689357. [DOI] [PubMed] [Google Scholar]
- Abram J. W., Nedwell D. B. Inhibition of methanogenesis by sulphate reducing bacteria competing for transferred hydrogen. Arch Microbiol. 1978 Apr 27;117(1):89–92. doi: 10.1007/BF00689356. [DOI] [PubMed] [Google Scholar]
- Allison C., Macfarlane G. T. Effect of nitrate on methane production and fermentation by slurries of human faecal bacteria. J Gen Microbiol. 1988 Jun;134(6):1397–1405. doi: 10.1099/00221287-134-6-1397. [DOI] [PubMed] [Google Scholar]
- Badziong W., Thauer R. K. Growth yields and growth rates of Desulfovibrio vulgaris (Marburg) growing on hydrogen plus sulfate and hydrogen plus thiosulfate as the sole energy sources. Arch Microbiol. 1978 May 30;117(2):209–214. doi: 10.1007/BF00402310. [DOI] [PubMed] [Google Scholar]
- Cappenberg T. E. Interrelations between sulfate-reducing and methane-producing bacteria in bottom deposits of a fresh-water lake. I. Field observations. Antonie Van Leeuwenhoek. 1974;40(2):285–295. doi: 10.1007/BF00394387. [DOI] [PubMed] [Google Scholar]
- Cappenberg T. E. Interrelations between sulfate-reducing and methane-producing bacteria in bottom deposits of a fresh-water lake. II. Inhibition experiments. Antonie Van Leeuwenhoek. 1974;40(2):297–306. doi: 10.1007/BF00394388. [DOI] [PubMed] [Google Scholar]
- Cummings J. H., Pomare E. W., Branch W. J., Naylor C. P., Macfarlane G. T. Short chain fatty acids in human large intestine, portal, hepatic and venous blood. Gut. 1987 Oct;28(10):1221–1227. doi: 10.1136/gut.28.10.1221. [DOI] [PMC free article] [PubMed] [Google Scholar]
- Englyst H. N., Cummings J. H. Digestion of polysaccharides of potato in the small intestine of man. Am J Clin Nutr. 1987 Feb;45(2):423–431. doi: 10.1093/ajcn/45.2.423. [DOI] [PubMed] [Google Scholar]
- Englyst H. N., Cummings J. H. Digestion of the carbohydrates of banana (Musa paradisiaca sapientum) in the human small intestine. Am J Clin Nutr. 1986 Jul;44(1):42–50. doi: 10.1093/ajcn/44.1.42. [DOI] [PubMed] [Google Scholar]
- Forstner J. F. Intestinal mucins in health and disease. Digestion. 1978;17(3):234–263. doi: 10.1159/000198115. [DOI] [PubMed] [Google Scholar]
- Hoskins L. C., Agustines M., McKee W. B., Boulding E. T., Kriaris M., Niedermeyer G. Mucin degradation in human colon ecosystems. Isolation and properties of fecal strains that degrade ABH blood group antigens and oligosaccharides from mucin glycoproteins. J Clin Invest. 1985 Mar;75(3):944–953. doi: 10.1172/JCI111795. [DOI] [PMC free article] [PubMed] [Google Scholar]
- Hoskins L. C., Boulding E. T. Mucin degradation in human colon ecosystems. Evidence for the existence and role of bacterial subpopulations producing glycosidases as extracellular enzymes. J Clin Invest. 1981 Jan;67(1):163–172. doi: 10.1172/JCI110009. [DOI] [PMC free article] [PubMed] [Google Scholar]
- Laanbroek H. J., Geerligs H. J., Sijtsma L., Veldkamp H. Competition for sulfate and ethanol among desulfobacter, desulfobulbus, and desulfovibrio species isolated from intertidal sediments. Appl Environ Microbiol. 1984 Feb;47(2):329–334. doi: 10.1128/aem.47.2.329-334.1984. [DOI] [PMC free article] [PubMed] [Google Scholar]
- Macfarlane G. T., Englyst H. N. Starch utilization by the human large intestinal microflora. J Appl Bacteriol. 1986 Mar;60(3):195–201. doi: 10.1111/j.1365-2672.1986.tb01073.x. [DOI] [PubMed] [Google Scholar]
- Miller T. L., Wolin M. J. Enumeration of Methanobrevibacter smithii in human feces. Arch Microbiol. 1982 Feb;131(1):14–18. doi: 10.1007/BF00451492. [DOI] [PubMed] [Google Scholar]
- Miller T. L., Wolin M. J. Methanosphaera stadtmaniae gen. nov., sp. nov.: a species that forms methane by reducing methanol with hydrogen. Arch Microbiol. 1985 Mar;141(2):116–122. doi: 10.1007/BF00423270. [DOI] [PubMed] [Google Scholar]
- Mountfort D. O., Asher R. A. Role of sulfate reduction versus methanogenesis in terminal carbon flow in polluted intertidal sediment of waimea inlet, nelson, new zealand. Appl Environ Microbiol. 1981 Aug;42(2):252–258. doi: 10.1128/aem.42.2.252-258.1981. [DOI] [PMC free article] [PubMed] [Google Scholar]
- Roberton A. M., Stanley R. A. In vitro utilization of mucin by Bacteroides fragilis. Appl Environ Microbiol. 1982 Feb;43(2):325–330. doi: 10.1128/aem.43.2.325-330.1982. [DOI] [PMC free article] [PubMed] [Google Scholar]
- Salyers A. A., Vercellotti J. R., West S. E., Wilkins T. D. Fermentation of mucin and plant polysaccharides by strains of Bacteroides from the human colon. Appl Environ Microbiol. 1977 Feb;33(2):319–322. doi: 10.1128/aem.33.2.319-322.1977. [DOI] [PMC free article] [PubMed] [Google Scholar]
- Vercellotti J. R., Salyers A. A., Bullard W. S., Wilkins D. Breakdown of mucin and plant polysaccharides in the human colon. Can J Biochem. 1977 Nov;55(11):1190–1196. doi: 10.1139/o77-178. [DOI] [PubMed] [Google Scholar]
- Winfrey M. R., Zeikus J. G. Effect of sulfate on carbon and electron flow during microbial methanogenesis in freshwater sediments. Appl Environ Microbiol. 1977 Feb;33(2):275–281. doi: 10.1128/aem.33.2.275-281.1977. [DOI] [PMC free article] [PubMed] [Google Scholar]