Abstract
The energetics and accumulation of solutes in Saccharomyces cerevisiae were investigated for cells grown aerobically in a chemostat under NaCl stress and glucose limitation. Changed energy requirements in relation to external salinity were examined by energy balance determinations performed by substrate and product analyses, with the latter including heat measurements by microcalorimetry. In both 0 and 0.9 M NaCl cultures, the catabolism was entirely respiratory at the lowest dilution rates tested but shifted to a mixed respiratory-fermentative metabolism at higher dilution rates. This shift occurred at a considerably lower dilution rate for salt-grown cells. The intracellular solute concentrations, as calculated on the basis of intracellular soluble space determinations, showed that the internal Na+ concentration increased from about 0.02 molal in basal medium to about 0.18 molal in 0.9 M NaCl medium, while intracellular K+ was maintained around 0.29 molal despite the variation in external salinity. The intracellular glycerol concentration increased from below 0.05 molal at low salinity to about 1.2 molal at 0.9 M NaCl. The concentrations of the internal solutes, however, changed insignificantly with growth rate and energy metabolism. The additional maintenance energy expenditure for growth at 0.9 M NaCl was, depending on the growth rate, 14 to 31% of the total energy requirement for growth at 0 M NaCl. Including the energy conserved in glycerol, the total additional energy demand for growth at 0.9 M NaCl corresponded to 28 to 51% of the energy required for growth at 0 M NaCl.
Full text
PDF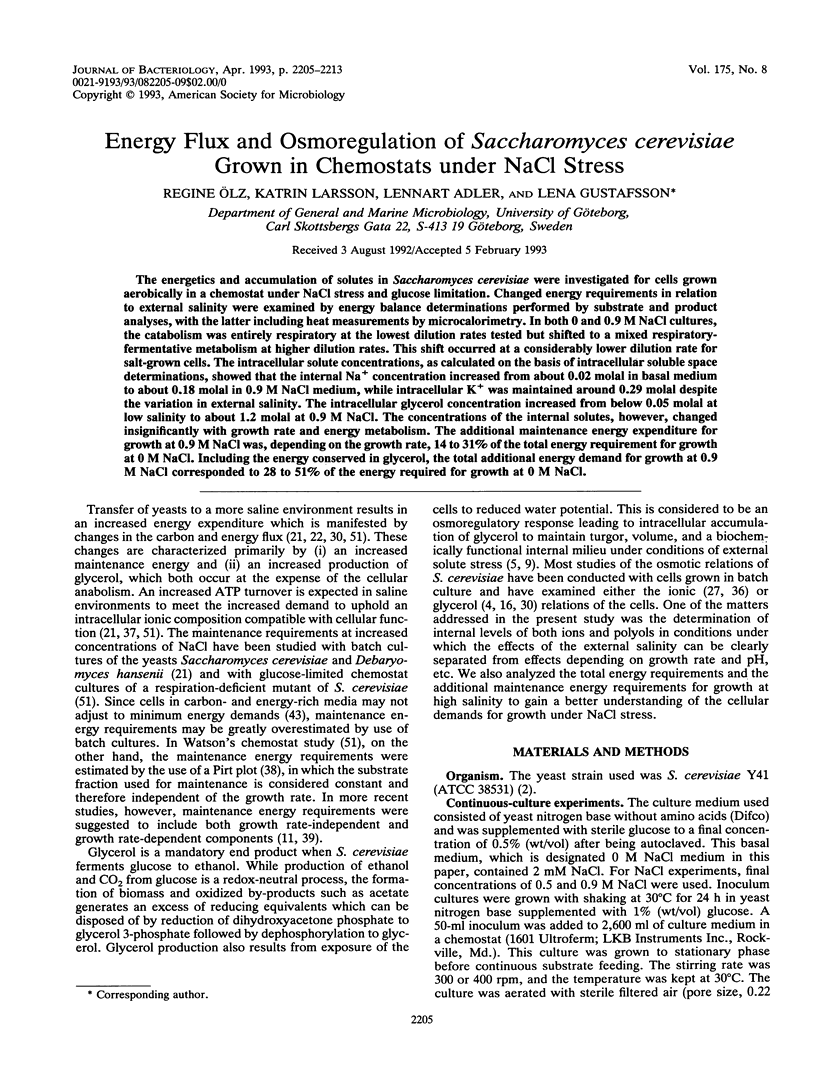
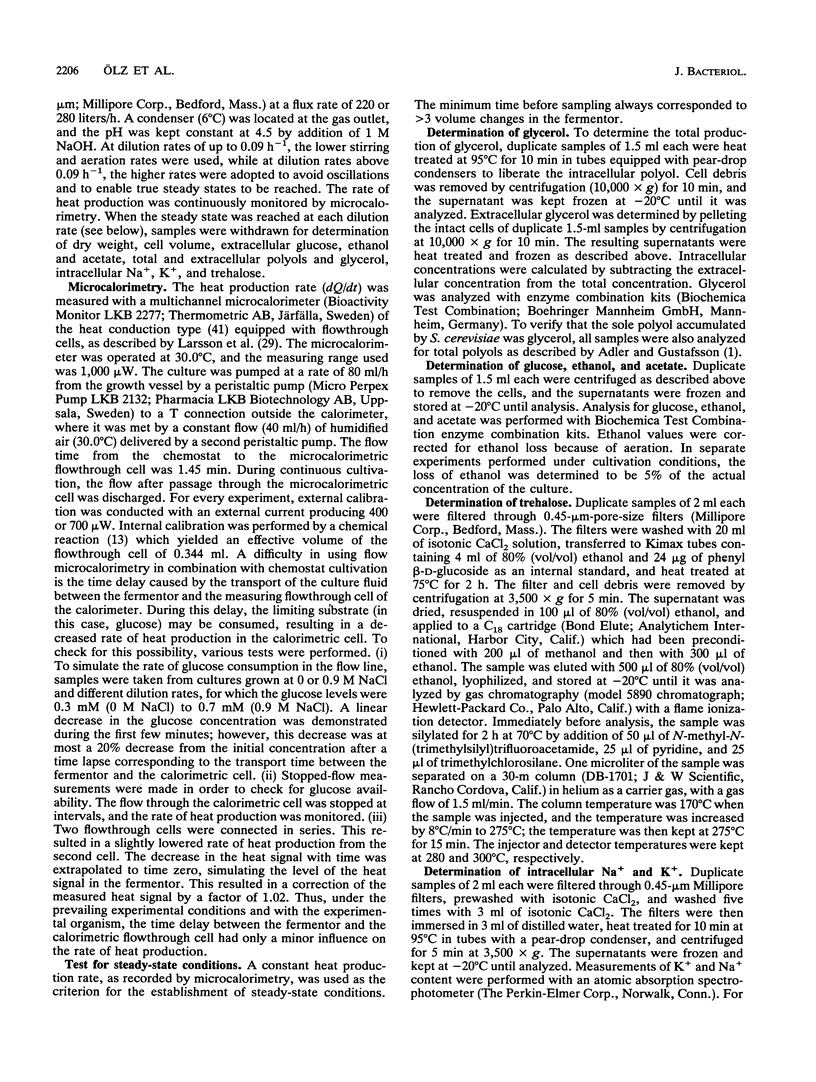
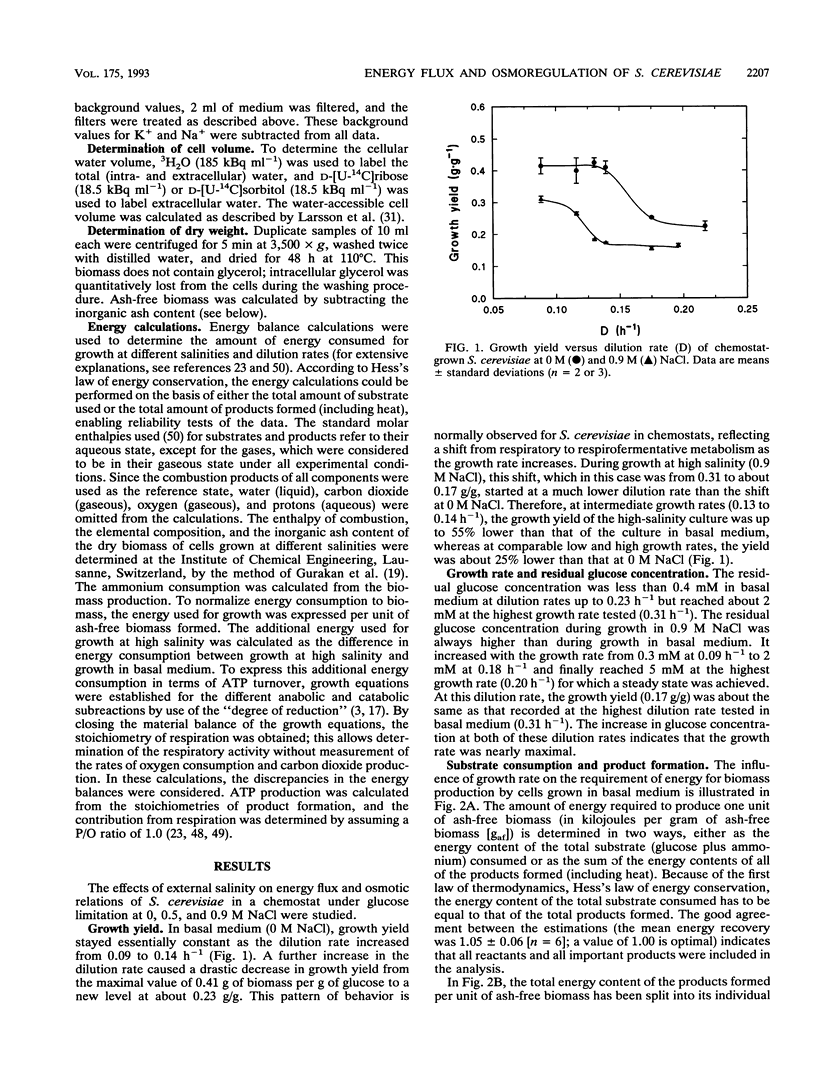
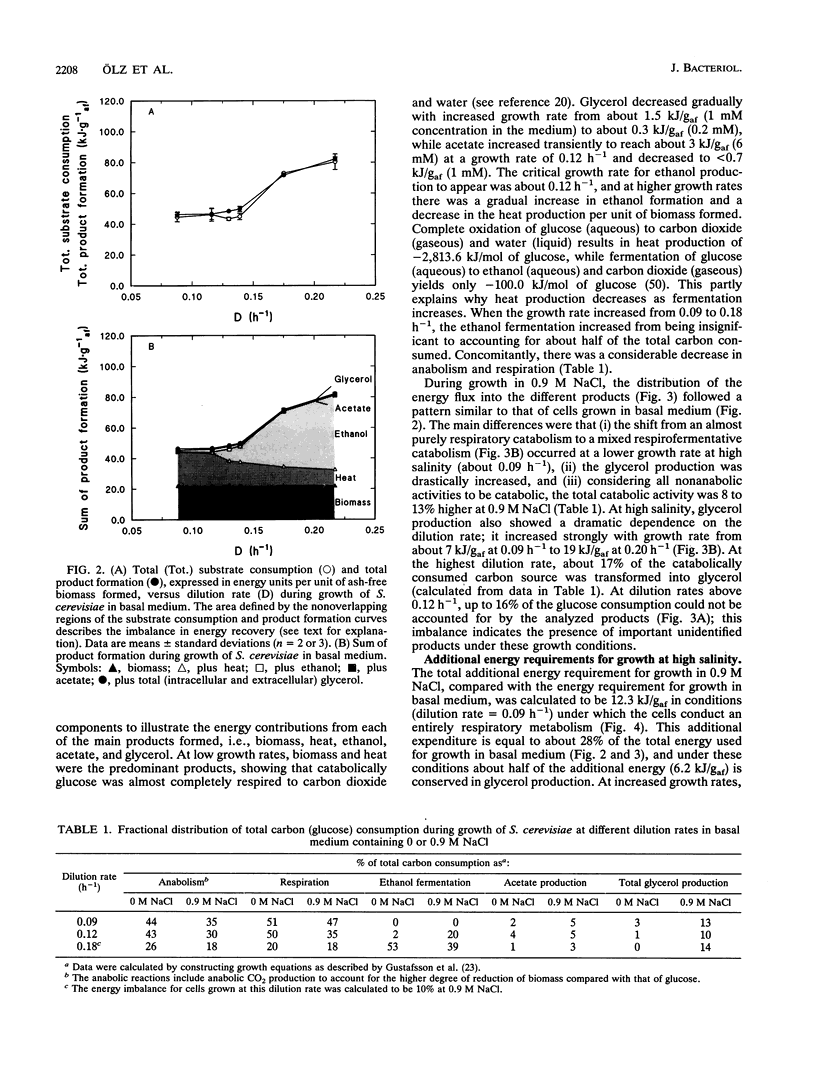
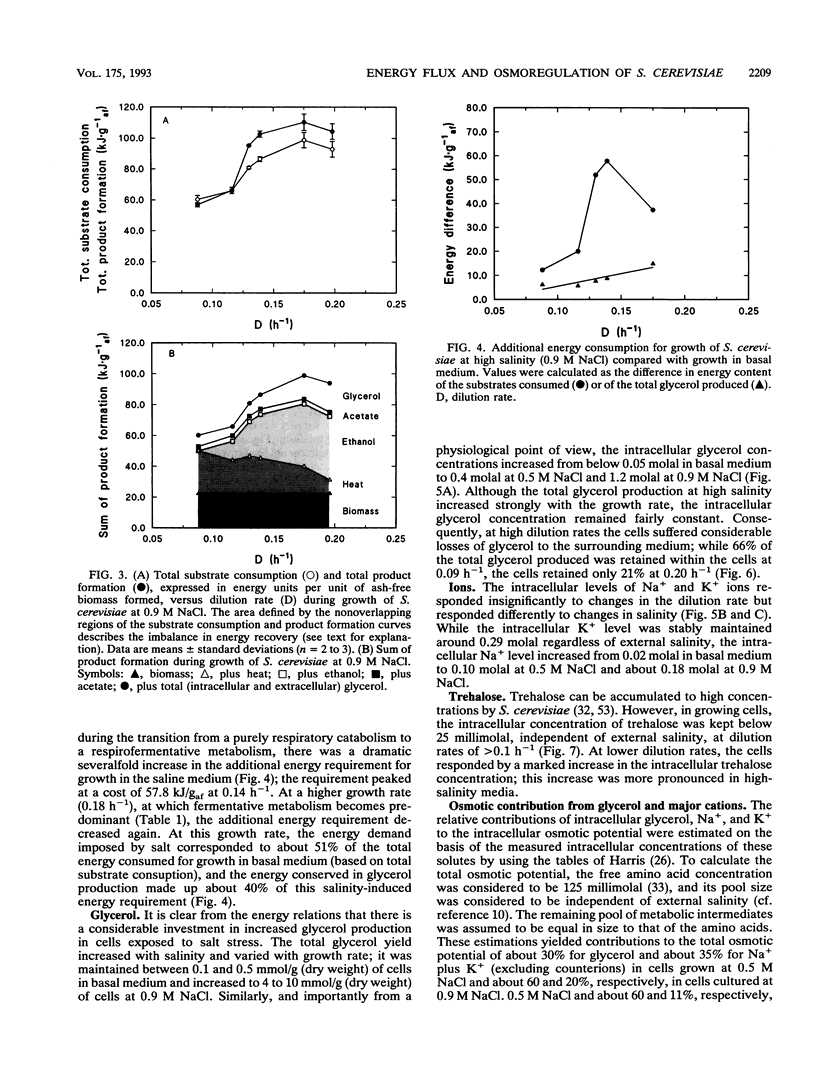
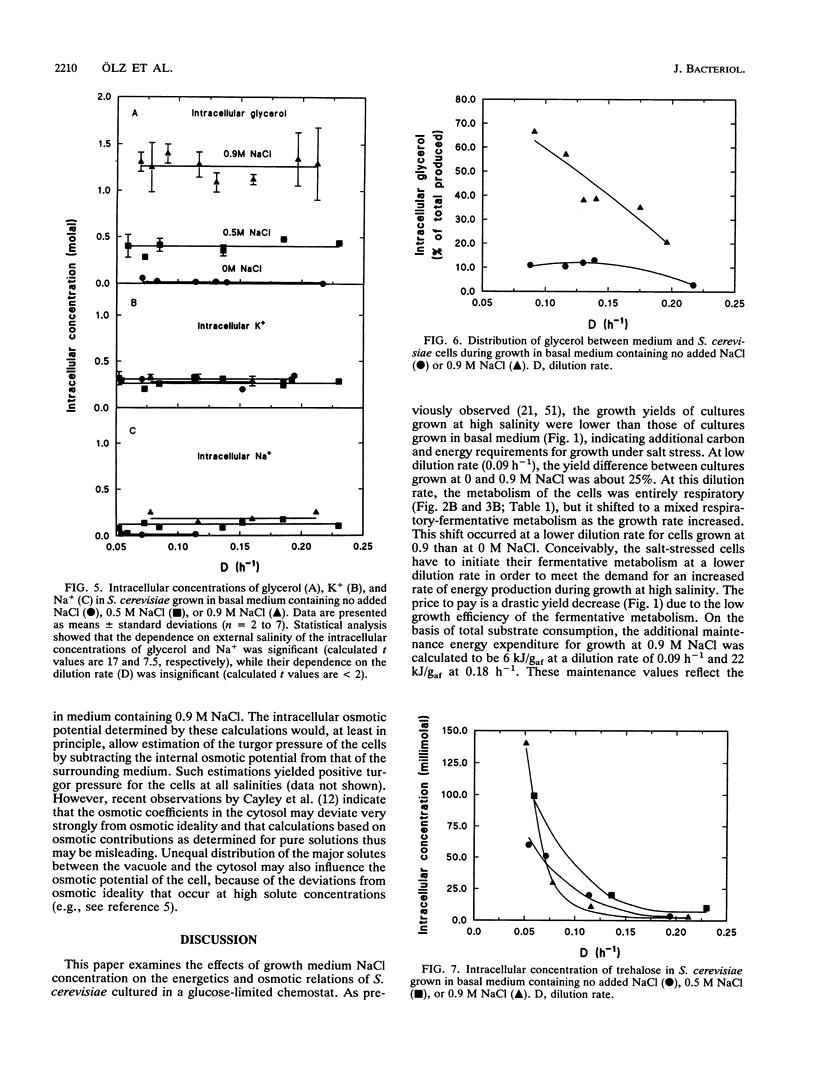
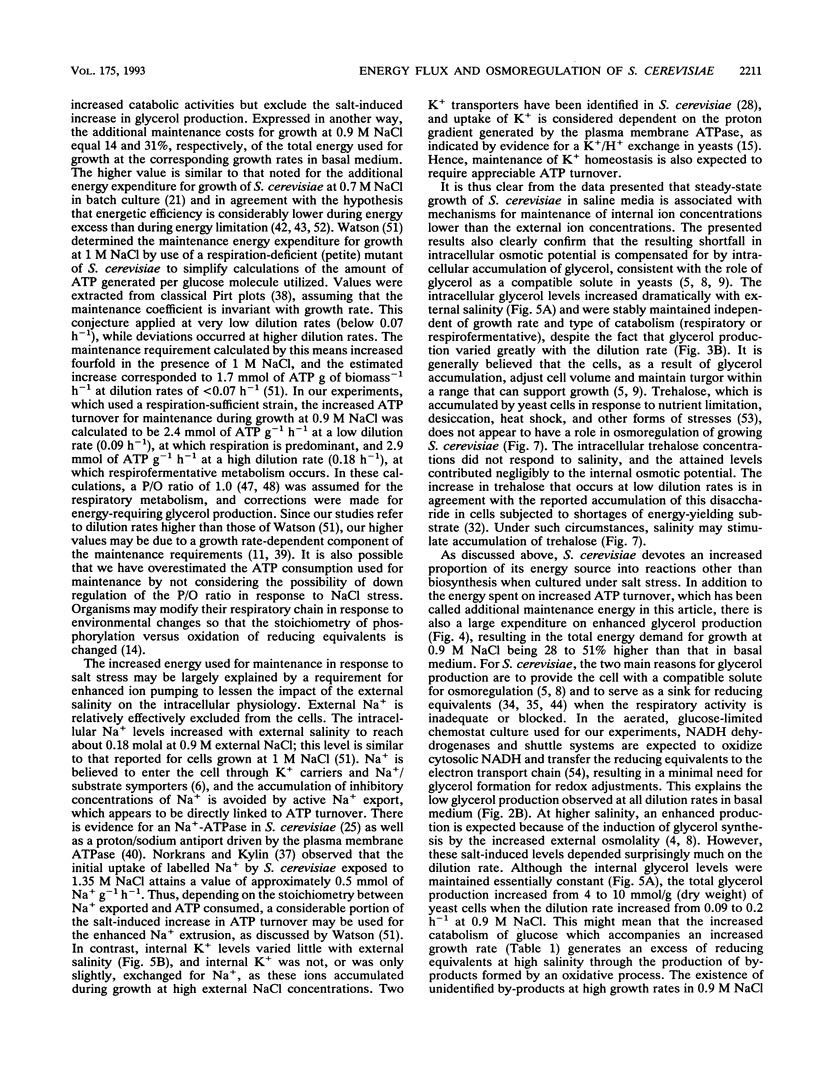
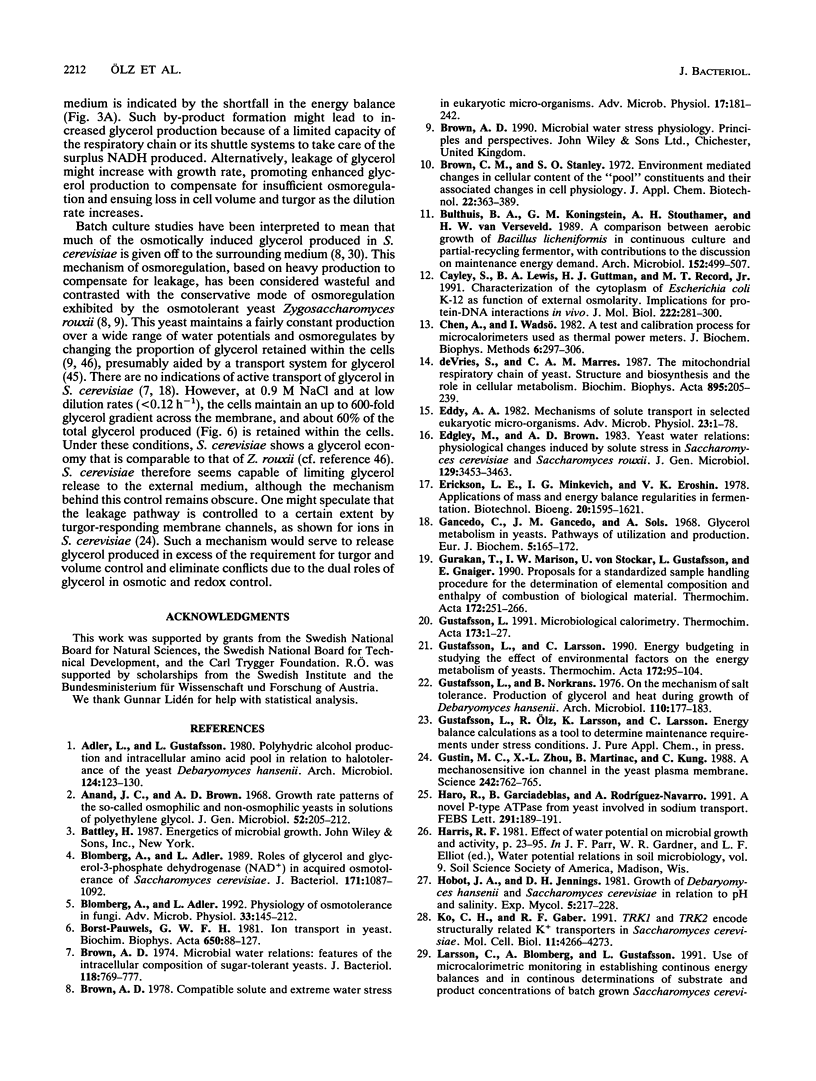
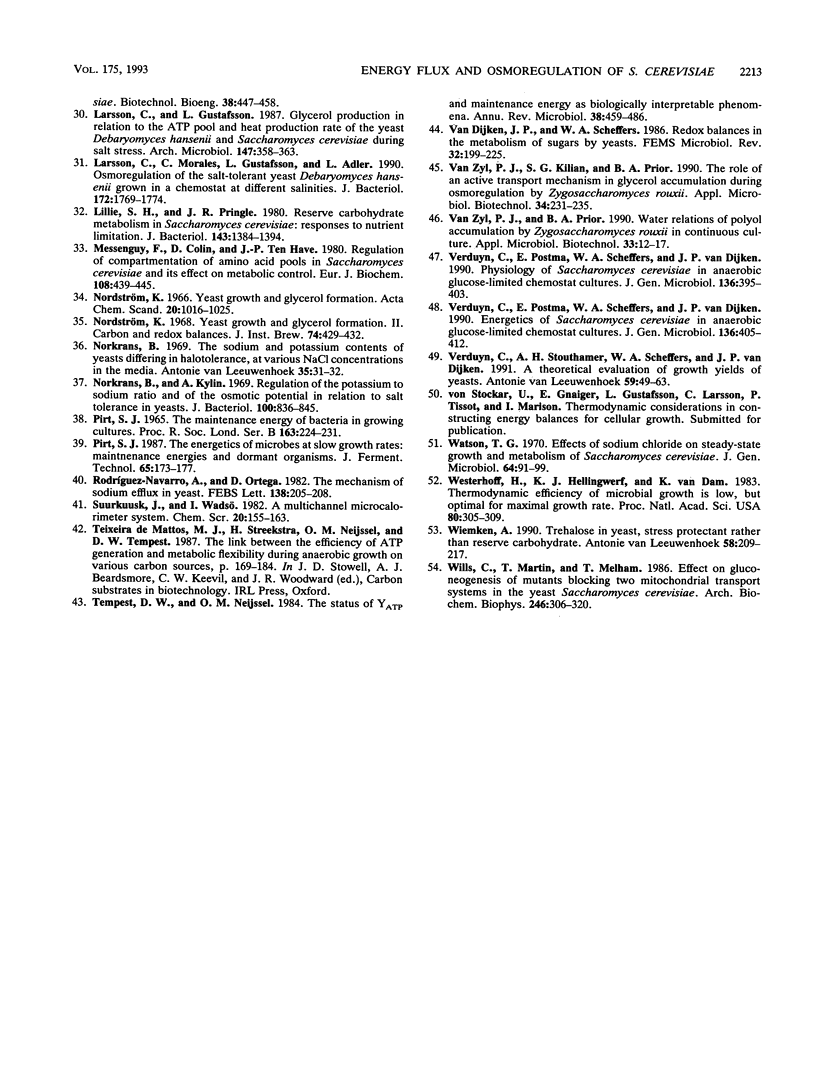
Images in this article
Selected References
These references are in PubMed. This may not be the complete list of references from this article.
- Blomberg A., Adler L. Physiology of osmotolerance in fungi. Adv Microb Physiol. 1992;33:145–212. doi: 10.1016/s0065-2911(08)60217-9. [DOI] [PubMed] [Google Scholar]
- Blomberg A., Adler L. Roles of glycerol and glycerol-3-phosphate dehydrogenase (NAD+) in acquired osmotolerance of Saccharomyces cerevisiae. J Bacteriol. 1989 Feb;171(2):1087–1092. doi: 10.1128/jb.171.2.1087-1092.1989. [DOI] [PMC free article] [PubMed] [Google Scholar]
- Borst-Pauwels G. W. Ion transport in yeast. Biochim Biophys Acta. 1981 Dec;650(2-3):88–127. doi: 10.1016/0304-4157(81)90002-2. [DOI] [PubMed] [Google Scholar]
- Brown A. D. Compatible solutes and extreme water stress in eukaryotic micro-organisms. Adv Microb Physiol. 1978;17:181–242. doi: 10.1016/s0065-2911(08)60058-2. [DOI] [PubMed] [Google Scholar]
- Brown A. D. Microbial water relations: features of the intracellular composition of sugar-tolerant yeasts. J Bacteriol. 1974 Jun;118(3):769–777. doi: 10.1128/jb.118.3.769-777.1974. [DOI] [PMC free article] [PubMed] [Google Scholar]
- Cayley S., Lewis B. A., Guttman H. J., Record M. T., Jr Characterization of the cytoplasm of Escherichia coli K-12 as a function of external osmolarity. Implications for protein-DNA interactions in vivo. J Mol Biol. 1991 Nov 20;222(2):281–300. doi: 10.1016/0022-2836(91)90212-o. [DOI] [PubMed] [Google Scholar]
- Chen A., Wadsö I. A test and calibration process for microcalorimeters used as thermal power meters. J Biochem Biophys Methods. 1982 Sep;6(4):297–306. doi: 10.1016/0165-022x(82)90011-2. [DOI] [PubMed] [Google Scholar]
- Eddy A. A. Mechanisms of solute transport in selected eukaryotic micro-organisms. Adv Microb Physiol. 1982;23:1-78, 269-70. doi: 10.1016/s0065-2911(08)60335-5. [DOI] [PubMed] [Google Scholar]
- Gancedo C., Gancedo J. M., Sols A. Glycerol metabolism in yeasts. Pathways of utilization and production. Eur J Biochem. 1968 Jul;5(2):165–172. doi: 10.1111/j.1432-1033.1968.tb00353.x. [DOI] [PubMed] [Google Scholar]
- Gustafsson L., Norkrans B. On the mechanism of salt tolerance. Production of glycerol and heat during growth of Debaryomyces hansenii. Arch Microbiol. 1976 Nov 2;110(23):177–183. doi: 10.1007/BF00690226. [DOI] [PubMed] [Google Scholar]
- Gustin M. C., Zhou X. L., Martinac B., Kung C. A mechanosensitive ion channel in the yeast plasma membrane. Science. 1988 Nov 4;242(4879):762–765. doi: 10.1126/science.2460920. [DOI] [PubMed] [Google Scholar]
- Haro R., Garciadeblas B., Rodríguez-Navarro A. A novel P-type ATPase from yeast involved in sodium transport. FEBS Lett. 1991 Oct 21;291(2):189–191. doi: 10.1016/0014-5793(91)81280-l. [DOI] [PubMed] [Google Scholar]
- Ko C. H., Gaber R. F. TRK1 and TRK2 encode structurally related K+ transporters in Saccharomyces cerevisiae. Mol Cell Biol. 1991 Aug;11(8):4266–4273. doi: 10.1128/mcb.11.8.4266. [DOI] [PMC free article] [PubMed] [Google Scholar]
- Larsson C., Gustafsson L. Glycerol production in relation to the ATP pool and heat production rate of the yeasts Debaryomyces hansenii and Saccharomyces cerevisiae during salt stress. Arch Microbiol. 1987 May;147(4):358–363. doi: 10.1007/BF00406133. [DOI] [PubMed] [Google Scholar]
- Larsson C., Morales C., Gustafsson L., Adler L. Osmoregulation of the salt-tolerant yeast Debaryomyces hansenii grown in a chemostat at different salinities. J Bacteriol. 1990 Apr;172(4):1769–1774. doi: 10.1128/jb.172.4.1769-1774.1990. [DOI] [PMC free article] [PubMed] [Google Scholar]
- Lillie S. H., Pringle J. R. Reserve carbohydrate metabolism in Saccharomyces cerevisiae: responses to nutrient limitation. J Bacteriol. 1980 Sep;143(3):1384–1394. doi: 10.1128/jb.143.3.1384-1394.1980. [DOI] [PMC free article] [PubMed] [Google Scholar]
- Messenguy F., Colin D., ten Have J. P. Regulation of compartmentation of amino acid pools in Saccharomyces cerevisiae and its effects on metabolic control. Eur J Biochem. 1980 Jul;108(2):439–447. doi: 10.1111/j.1432-1033.1980.tb04740.x. [DOI] [PubMed] [Google Scholar]
- Nordström K. Yeast growth and glycerol formation. Acta Chem Scand. 1966;20(4):1016–1025. doi: 10.3891/acta.chem.scand.20-1016. [DOI] [PubMed] [Google Scholar]
- Norkrans B., Kylin A. Regulation of the potassium to sodium ratio and of the osmotic potential in relation to salt tolerance in yeasts. J Bacteriol. 1969 Nov;100(2):836–845. doi: 10.1128/jb.100.2.836-845.1969. [DOI] [PMC free article] [PubMed] [Google Scholar]
- Pirt S. J. The maintenance energy of bacteria in growing cultures. Proc R Soc Lond B Biol Sci. 1965 Oct 12;163(991):224–231. doi: 10.1098/rspb.1965.0069. [DOI] [PubMed] [Google Scholar]
- Rodríguez-Navarro A., Ortega M. D. The mechanism of sodium efflux in yeast. FEBS Lett. 1982 Feb 22;138(2):205–208. doi: 10.1016/0014-5793(82)80442-0. [DOI] [PubMed] [Google Scholar]
- Tempest D. W., Neijssel O. M. The status of YATP and maintenance energy as biologically interpretable phenomena. Annu Rev Microbiol. 1984;38:459–486. doi: 10.1146/annurev.mi.38.100184.002331. [DOI] [PubMed] [Google Scholar]
- Verduyn C., Postma E., Scheffers W. A., van Dijken J. P. Energetics of Saccharomyces cerevisiae in anaerobic glucose-limited chemostat cultures. J Gen Microbiol. 1990 Mar;136(3):405–412. doi: 10.1099/00221287-136-3-405. [DOI] [PubMed] [Google Scholar]
- Verduyn C., Postma E., Scheffers W. A., van Dijken J. P. Physiology of Saccharomyces cerevisiae in anaerobic glucose-limited chemostat cultures. J Gen Microbiol. 1990 Mar;136(3):395–403. doi: 10.1099/00221287-136-3-395. [DOI] [PubMed] [Google Scholar]
- Verduyn C., Stouthamer A. H., Scheffers W. A., van Dijken J. P. A theoretical evaluation of growth yields of yeasts. Antonie Van Leeuwenhoek. 1991 Jan;59(1):49–63. doi: 10.1007/BF00582119. [DOI] [PubMed] [Google Scholar]
- Watson T. G. Effects of sodium chloride on steady-state growth and metabolism of Saccharomyces cerevisiae. J Gen Microbiol. 1970 Nov;64(1):91–99. doi: 10.1099/00221287-64-1-91. [DOI] [PubMed] [Google Scholar]
- Westerhoff H. V., Hellingwerf K. J., Van Dam K. Thermodynamic efficiency of microbial growth is low but optimal for maximal growth rate. Proc Natl Acad Sci U S A. 1983 Jan;80(1):305–309. doi: 10.1073/pnas.80.1.305. [DOI] [PMC free article] [PubMed] [Google Scholar]
- Wickerham L. J. Yeast taxonomy in relation to ecology, genetics, and phylogeny. Antonie Van Leeuwenhoek. 1969 Jun;35(Suppl):31–58. [PubMed] [Google Scholar]
- Wiemken A. Trehalose in yeast, stress protectant rather than reserve carbohydrate. Antonie Van Leeuwenhoek. 1990 Oct;58(3):209–217. doi: 10.1007/BF00548935. [DOI] [PubMed] [Google Scholar]
- Wills C., Martin T., Melham T. Effect on gluconeogenesis of mutants blocking two mitochondrial transport systems in the yeast Saccharomyces cerevisiae. Arch Biochem Biophys. 1986 Apr;246(1):306–320. doi: 10.1016/0003-9861(86)90476-5. [DOI] [PubMed] [Google Scholar]
- de Vries S., Marres C. A. The mitochondrial respiratory chain of yeast. Structure and biosynthesis and the role in cellular metabolism. Biochim Biophys Acta. 1987;895(3):205–239. doi: 10.1016/s0304-4173(87)80003-4. [DOI] [PubMed] [Google Scholar]