Abstract
It has been reported that Escherichia coli is able to grow in the presence of carbonyl cyanide m-chlorophenylhydrazone (CCCP) when ATP is produced by glycolysis (N. Kinoshita et al., J. Bacteriol. 160:1074-1077, 1984). We investigated the effect of CCCP on the osmotic adaptation of E. coli growing with glucose. When E. coli growing in rich medium containing CCCP was transferred to medium containing sucrose, its growth stopped for a while and then started again. This lag time was negligible in the absence of CCCP. The same results were obtained when the osmolarity was increased by N-methylglucamine-maleic acid. In addition to adapting itself to the hyperosmotic rich medium, E. coli adapted itself to hyperosmolarity in a minimal medium containing CCCP, again with a lag time. Hyperosmotic shock decreased the internal level of potassium ion rather than causing the accumulation of external potassium ion in the presence of CCCP. The internal amount of glutamic acid increased in cells growing in hyperosmotic medium in the presence and absence of CCCP. Large elevations in levels of other amino acids were not observed in the cells adapted to hyperosmolarity. Trehalose was detected only in hyperosmosis-stressed cells in the presence and absence of CCCP. These results suggest that E. coli can adapt to changes in the environmental osmolarity with a negligible accumulation of osmolytes from the external milieu but that the accumulation may promote the adaptation.
Full text
PDF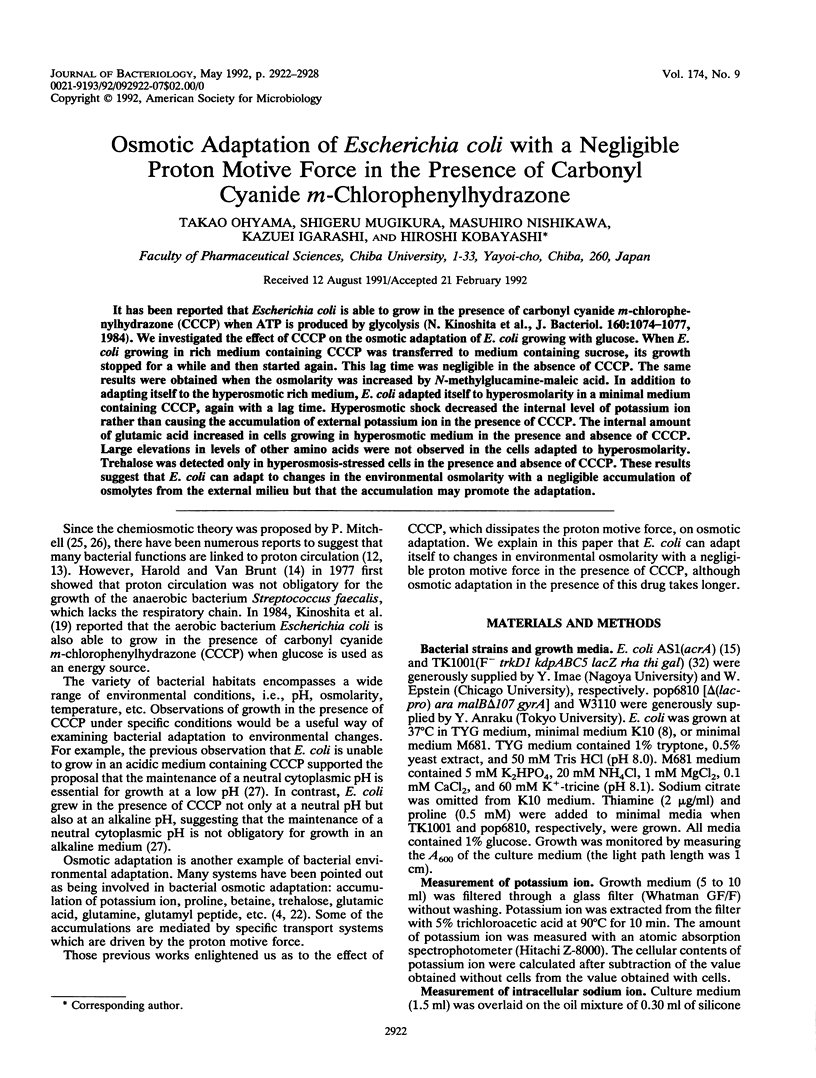
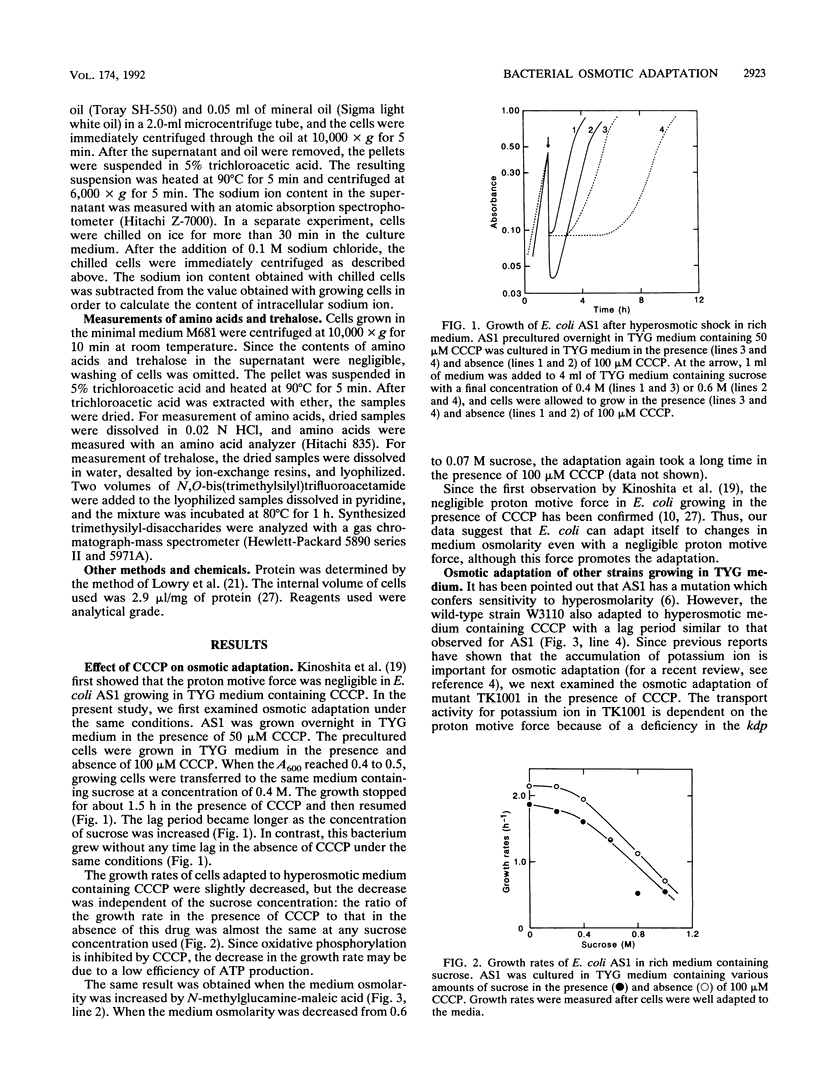
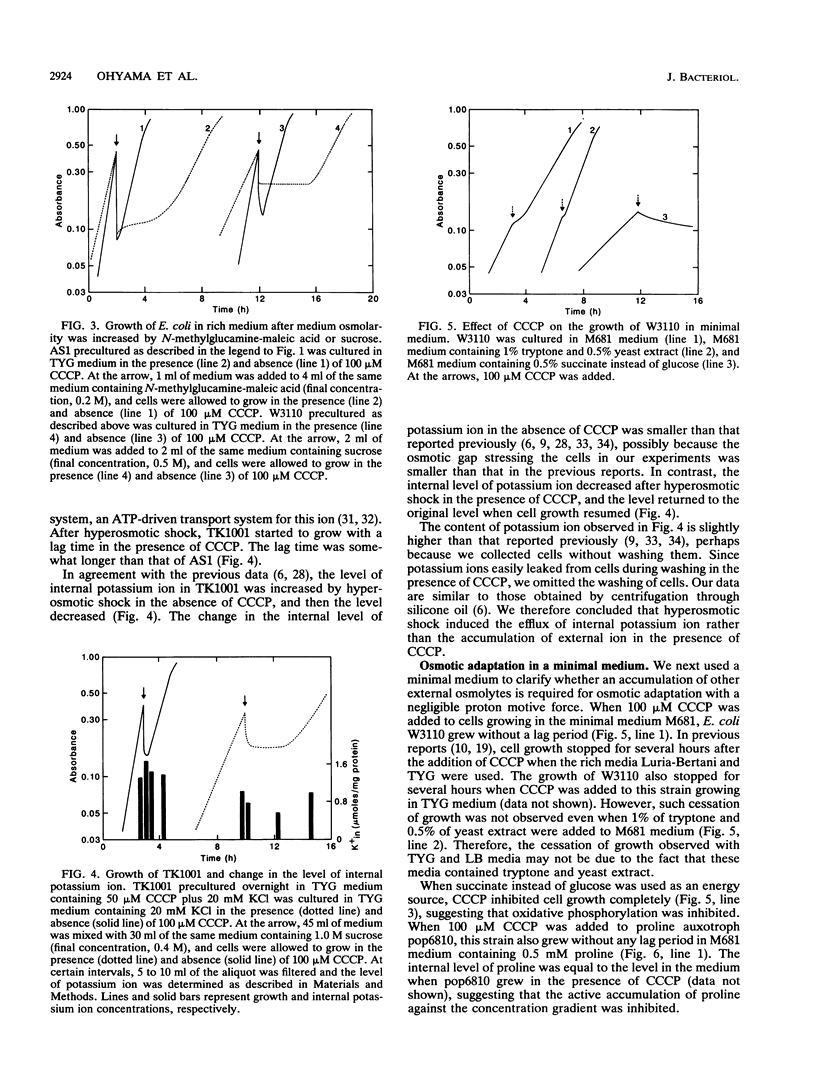
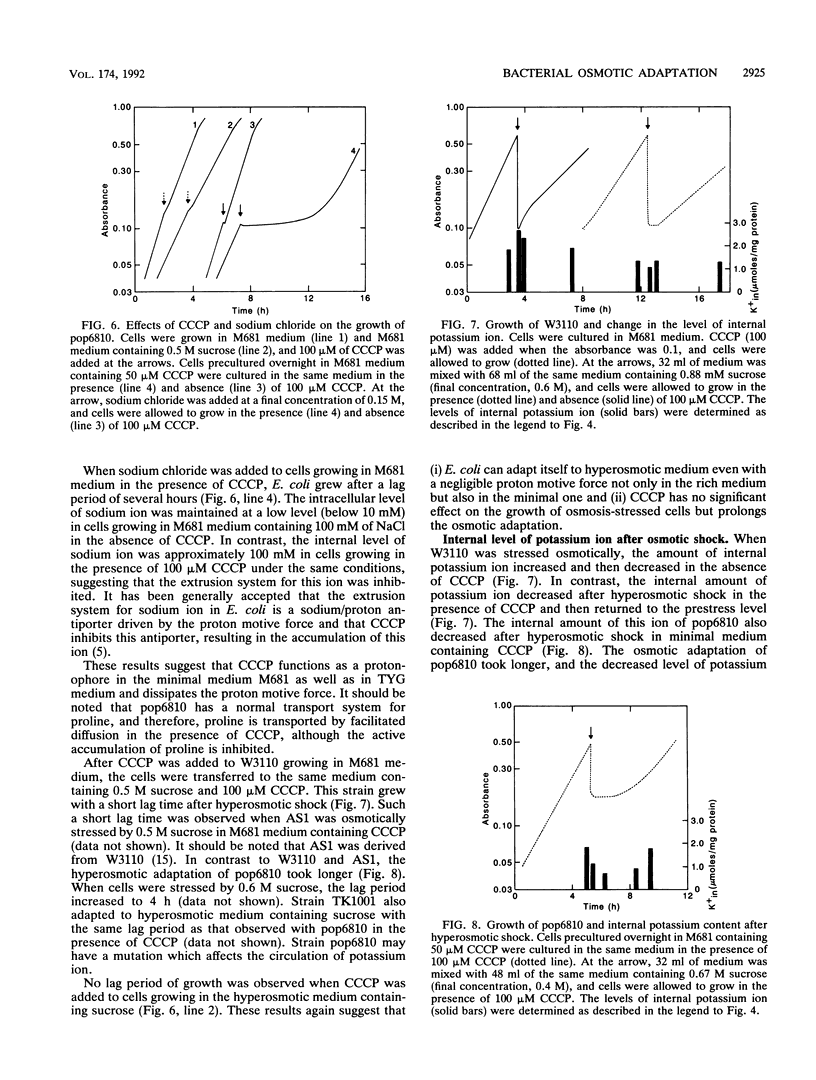
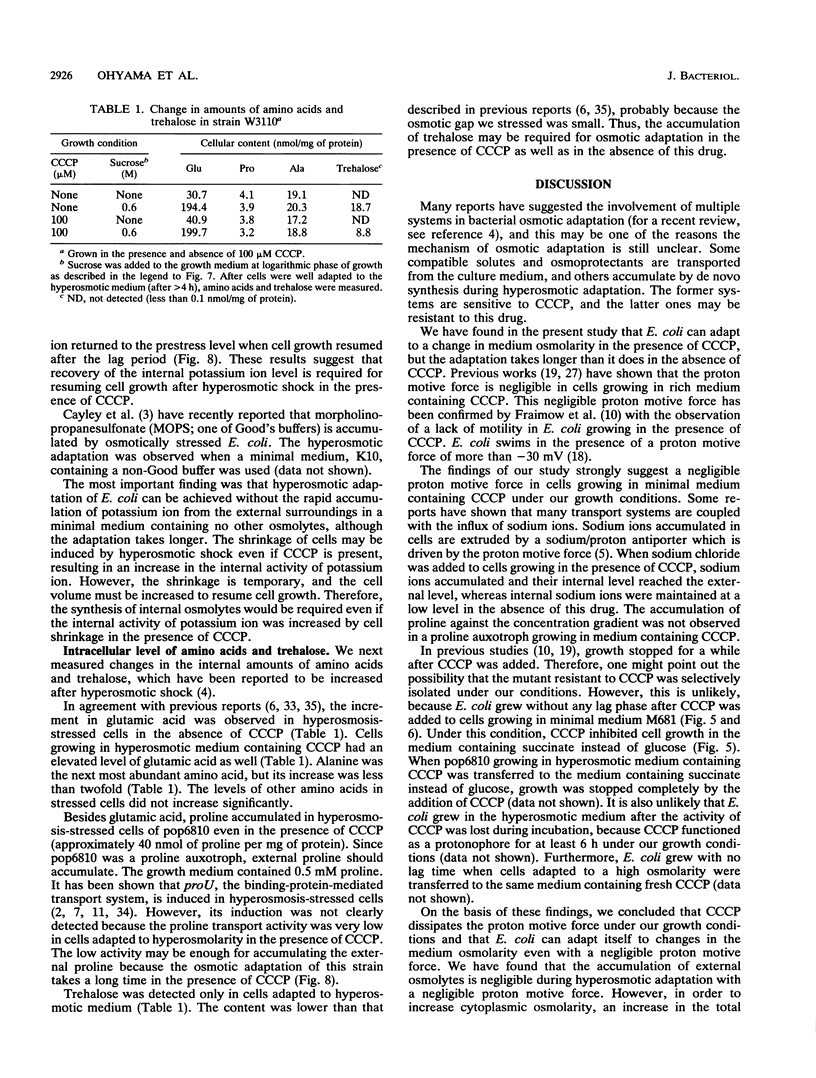
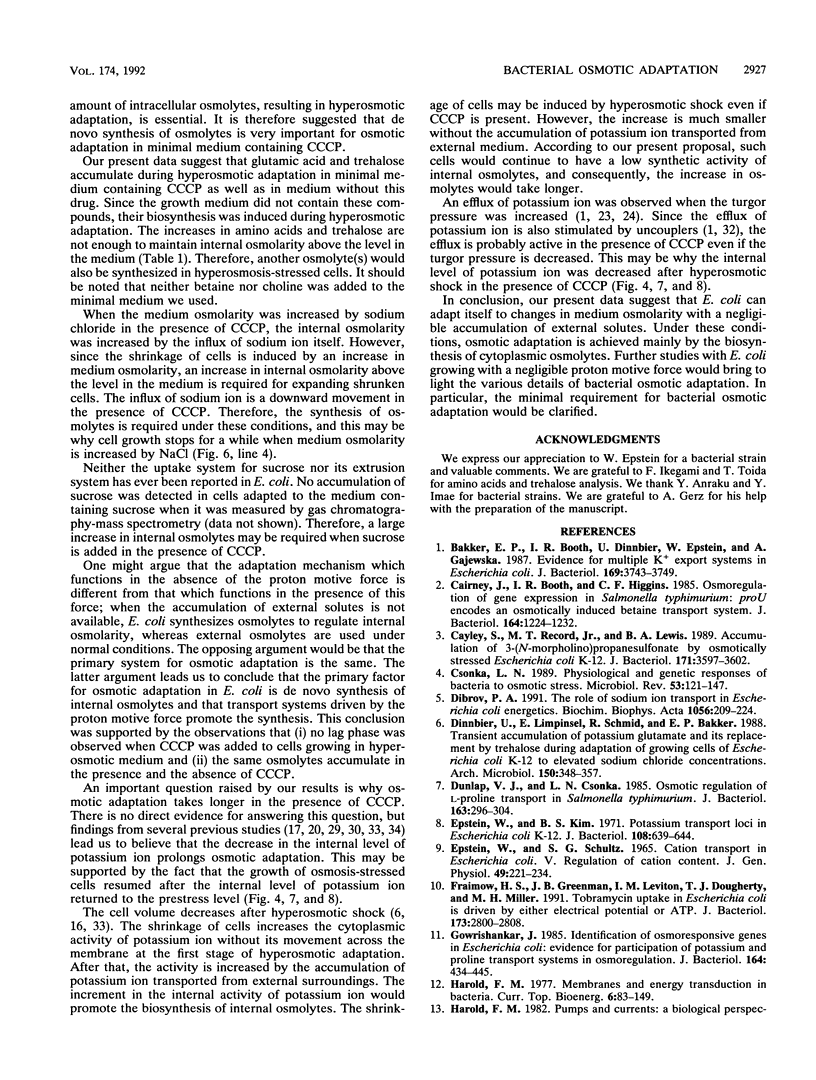
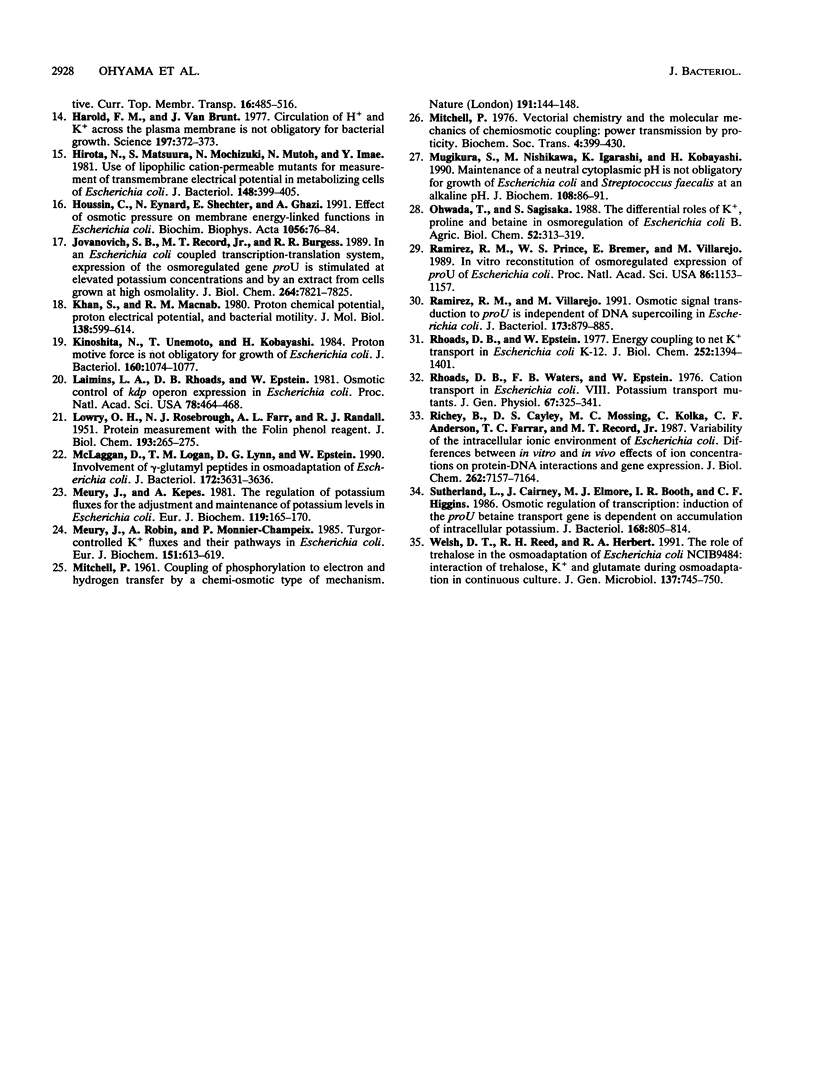
Selected References
These references are in PubMed. This may not be the complete list of references from this article.
- Bakker E. P., Booth I. R., Dinnbier U., Epstein W., Gajewska A. Evidence for multiple K+ export systems in Escherichia coli. J Bacteriol. 1987 Aug;169(8):3743–3749. doi: 10.1128/jb.169.8.3743-3749.1987. [DOI] [PMC free article] [PubMed] [Google Scholar]
- Cairney J., Booth I. R., Higgins C. F. Osmoregulation of gene expression in Salmonella typhimurium: proU encodes an osmotically induced betaine transport system. J Bacteriol. 1985 Dec;164(3):1224–1232. doi: 10.1128/jb.164.3.1224-1232.1985. [DOI] [PMC free article] [PubMed] [Google Scholar]
- Cayley S., Record M. T., Jr, Lewis B. A. Accumulation of 3-(N-morpholino)propanesulfonate by osmotically stressed Escherichia coli K-12. J Bacteriol. 1989 Jul;171(7):3597–3602. doi: 10.1128/jb.171.7.3597-3602.1989. [DOI] [PMC free article] [PubMed] [Google Scholar]
- Csonka L. N. Physiological and genetic responses of bacteria to osmotic stress. Microbiol Rev. 1989 Mar;53(1):121–147. doi: 10.1128/mr.53.1.121-147.1989. [DOI] [PMC free article] [PubMed] [Google Scholar]
- Dibrov P. A. The role of sodium ion transport in Escherichia coli energetics. Biochim Biophys Acta. 1991 Feb 8;1056(3):209–224. doi: 10.1016/s0005-2728(05)80052-0. [DOI] [PubMed] [Google Scholar]
- Dinnbier U., Limpinsel E., Schmid R., Bakker E. P. Transient accumulation of potassium glutamate and its replacement by trehalose during adaptation of growing cells of Escherichia coli K-12 to elevated sodium chloride concentrations. Arch Microbiol. 1988;150(4):348–357. doi: 10.1007/BF00408306. [DOI] [PubMed] [Google Scholar]
- Dunlap V. J., Csonka L. N. Osmotic regulation of L-proline transport in Salmonella typhimurium. J Bacteriol. 1985 Jul;163(1):296–304. doi: 10.1128/jb.163.1.296-304.1985. [DOI] [PMC free article] [PubMed] [Google Scholar]
- Epstein W., Kim B. S. Potassium transport loci in Escherichia coli K-12. J Bacteriol. 1971 Nov;108(2):639–644. doi: 10.1128/jb.108.2.639-644.1971. [DOI] [PMC free article] [PubMed] [Google Scholar]
- Fraimow H. S., Greenman J. B., Leviton I. M., Dougherty T. J., Miller M. H. Tobramycin uptake in Escherichia coli is driven by either electrical potential or ATP. J Bacteriol. 1991 May;173(9):2800–2808. doi: 10.1128/jb.173.9.2800-2808.1991. [DOI] [PMC free article] [PubMed] [Google Scholar]
- Gowrishankar J. Identification of osmoresponsive genes in Escherichia coli: evidence for participation of potassium and proline transport systems in osmoregulation. J Bacteriol. 1985 Oct;164(1):434–445. doi: 10.1128/jb.164.1.434-445.1985. [DOI] [PMC free article] [PubMed] [Google Scholar]
- Harold F. M., Van Brunt J. Circulation of H+ and K+ across the plasma membrane is not obligatory for bacterial growth. Science. 1977 Jul 22;197(4301):372–373. doi: 10.1126/science.69317. [DOI] [PubMed] [Google Scholar]
- Hirota N., Matsuura S., Mochizuki N., Mutoh N., Imae Y. Use of lipophilic cation-permeable mutants for measurement of transmembrane electrical potential in metabolizing cells of Escherichia coli. J Bacteriol. 1981 Nov;148(2):399–405. doi: 10.1128/jb.148.2.399-405.1981. [DOI] [PMC free article] [PubMed] [Google Scholar]
- Houssin C., Eynard N., Shechter E., Ghazi A. Effect of osmotic pressure on membrane energy-linked functions in Escherichia coli. Biochim Biophys Acta. 1991 Jan 3;1056(1):76–84. doi: 10.1016/s0005-2728(05)80075-1. [DOI] [PubMed] [Google Scholar]
- Jovanovich S. B., Record M. T., Jr, Burgess R. R. In an Escherichia coli coupled transcription-translation system, expression of the osmoregulated gene proU is stimulated at elevated potassium concentrations and by an extract from cells grown at high osmolality. J Biol Chem. 1989 May 15;264(14):7821–7825. [PubMed] [Google Scholar]
- Khan S., Macnab R. M. Proton chemical potential, proton electrical potential and bacterial motility. J Mol Biol. 1980 Apr 15;138(3):599–614. doi: 10.1016/s0022-2836(80)80019-2. [DOI] [PubMed] [Google Scholar]
- Kinoshita N., Unemoto T., Kobayashi H. Proton motive force is not obligatory for growth of Escherichia coli. J Bacteriol. 1984 Dec;160(3):1074–1077. doi: 10.1128/jb.160.3.1074-1077.1984. [DOI] [PMC free article] [PubMed] [Google Scholar]
- LOWRY O. H., ROSEBROUGH N. J., FARR A. L., RANDALL R. J. Protein measurement with the Folin phenol reagent. J Biol Chem. 1951 Nov;193(1):265–275. [PubMed] [Google Scholar]
- Laimins L. A., Rhoads D. B., Epstein W. Osmotic control of kdp operon expression in Escherichia coli. Proc Natl Acad Sci U S A. 1981 Jan;78(1):464–468. doi: 10.1073/pnas.78.1.464. [DOI] [PMC free article] [PubMed] [Google Scholar]
- MITCHELL P. Coupling of phosphorylation to electron and hydrogen transfer by a chemi-osmotic type of mechanism. Nature. 1961 Jul 8;191:144–148. doi: 10.1038/191144a0. [DOI] [PubMed] [Google Scholar]
- McLaggan D., Logan T. M., Lynn D. G., Epstein W. Involvement of gamma-glutamyl peptides in osmoadaptation of Escherichia coli. J Bacteriol. 1990 Jul;172(7):3631–3636. doi: 10.1128/jb.172.7.3631-3636.1990. [DOI] [PMC free article] [PubMed] [Google Scholar]
- Meury J., Kepes A. The regulation of potassium fluxes for the adjustment and maintenance of potassium levels in Escherichia coli. Eur J Biochem. 1981 Sep;119(1):165–170. doi: 10.1111/j.1432-1033.1981.tb05589.x. [DOI] [PubMed] [Google Scholar]
- Meury J., Robin A., Monnier-Champeix P. Turgor-controlled K+ fluxes and their pathways in Escherichia coli. Eur J Biochem. 1985 Sep 16;151(3):613–619. doi: 10.1111/j.1432-1033.1985.tb09148.x. [DOI] [PubMed] [Google Scholar]
- Mitchell P. Vectorial chemistry and the molecular mechanics of chemiosmotic coupling: power transmission by proticity. Biochem Soc Trans. 1976;4(3):399–430. doi: 10.1042/bst0040399. [DOI] [PubMed] [Google Scholar]
- Mugikura S., Nishikawa M., Igarashi K., Kobayashi H. Maintenance of a neutral cytoplasmic pH is not obligatory for growth of Escherichia coli and Streptococcus faecalis at an alkaline pH. J Biochem. 1990 Jul;108(1):86–91. doi: 10.1093/oxfordjournals.jbchem.a123168. [DOI] [PubMed] [Google Scholar]
- Ramirez R. M., Prince W. S., Bremer E., Villarejo M. In vitro reconstitution of osmoregulated expression of proU of Escherichia coli. Proc Natl Acad Sci U S A. 1989 Feb;86(4):1153–1157. doi: 10.1073/pnas.86.4.1153. [DOI] [PMC free article] [PubMed] [Google Scholar]
- Ramirez R. M., Villarejo M. Osmotic signal transduction to proU is independent of DNA supercoiling in Escherichia coli. J Bacteriol. 1991 Jan;173(2):879–885. doi: 10.1128/jb.173.2.879-885.1991. [DOI] [PMC free article] [PubMed] [Google Scholar]
- Rhoads D. B., Epstein W. Energy coupling to net K+ transport in Escherichia coli K-12. J Biol Chem. 1977 Feb 25;252(4):1394–1401. [PubMed] [Google Scholar]
- Rhoads D. B., Waters F. B., Epstein W. Cation transport in Escherichia coli. VIII. Potassium transport mutants. J Gen Physiol. 1976 Mar;67(3):325–341. doi: 10.1085/jgp.67.3.325. [DOI] [PMC free article] [PubMed] [Google Scholar]
- Richey B., Cayley D. S., Mossing M. C., Kolka C., Anderson C. F., Farrar T. C., Record M. T., Jr Variability of the intracellular ionic environment of Escherichia coli. Differences between in vitro and in vivo effects of ion concentrations on protein-DNA interactions and gene expression. J Biol Chem. 1987 May 25;262(15):7157–7164. [PubMed] [Google Scholar]
- Sutherland L., Cairney J., Elmore M. J., Booth I. R., Higgins C. F. Osmotic regulation of transcription: induction of the proU betaine transport gene is dependent on accumulation of intracellular potassium. J Bacteriol. 1986 Nov;168(2):805–814. doi: 10.1128/jb.168.2.805-814.1986. [DOI] [PMC free article] [PubMed] [Google Scholar]
- Welsh D. T., Reed R. H., Herbert R. A. The role of trehalose in the osmoadaptation of Escherichia coli NCIB 9484: interaction of trehalose, K+ and glutamate during osmoadaptation in continuous culture. J Gen Microbiol. 1991 Apr;137(4):745–750. doi: 10.1099/00221287-137-4-745. [DOI] [PubMed] [Google Scholar]