Abstract
Growth of the malolactic bacterium Leuconostoc oenos was improved with respect to both the specific growth rate and the biomass yield during the fermentation of glucose-malate mixtures as compared with those in media lacking malate. Such a finding indicates that the malolactic reaction contributed to the energy budget of the bacterium, suggesting that growth is energy limited in the absence of malate. An energetic yield (YATP) of 9.5 g of biomass.mol ATP-1 was found during growth on glucose with an ATP production by substrate-level phosphorylation of 1.2 mol of ATP.mol of glucose-1. During the period of mixed-substrate catabolism, an apparent YATP of 17.7 was observed, indicating a mixotrophy-associated ATP production of 2.2 mol of ATP.mol of glucose-1, or more correctly an energy gain of 0.28 mol of ATP.mol of malate-1, representing proton translocation flux from the cytoplasm to the exterior of 0.56 or 0.84 H+.mol of malate-1(depending on the H+/ATP stoichiometry). The growth-stimulating effect of malate was attributed to chemiosmotic transport mechanisms rather than proton consumption by the malolactic enzyme. Lactate efflux was by electroneutral lactate -/H+ symport having a constant stoichiometry, while malate uptake was predominantly by a malate -/H+ symport, though a low-affinity malate- uniport was also implicated. The measured electrical component (delta psi) of the proton motive force was altered, passing from -30 to -60 mV because of this translocation of dissociated organic acids when malolactic fermentation occurred.
Full text
PDF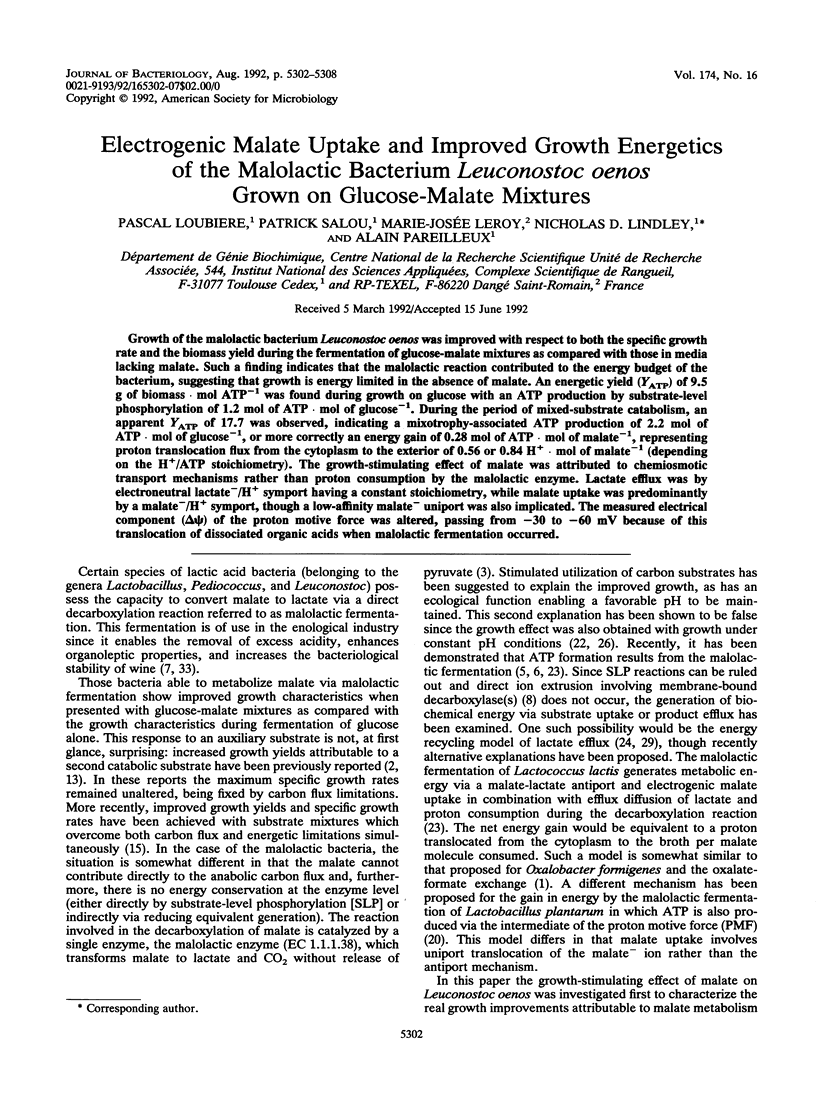
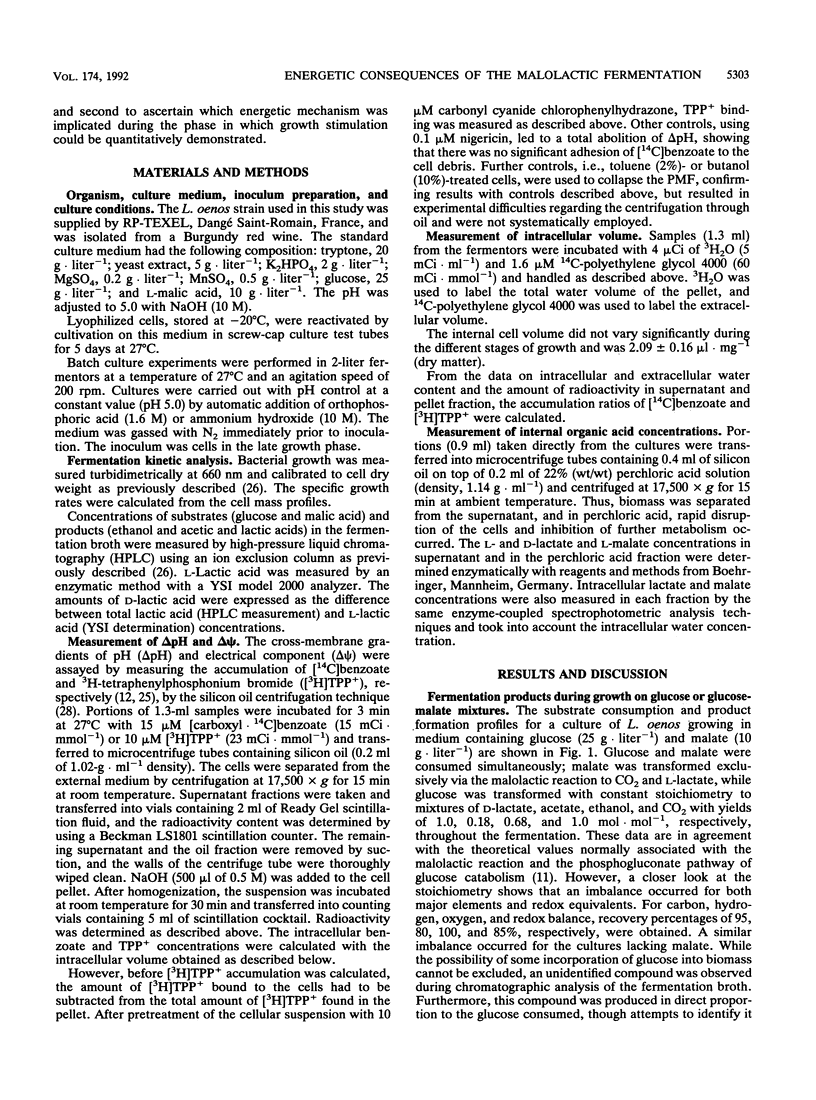
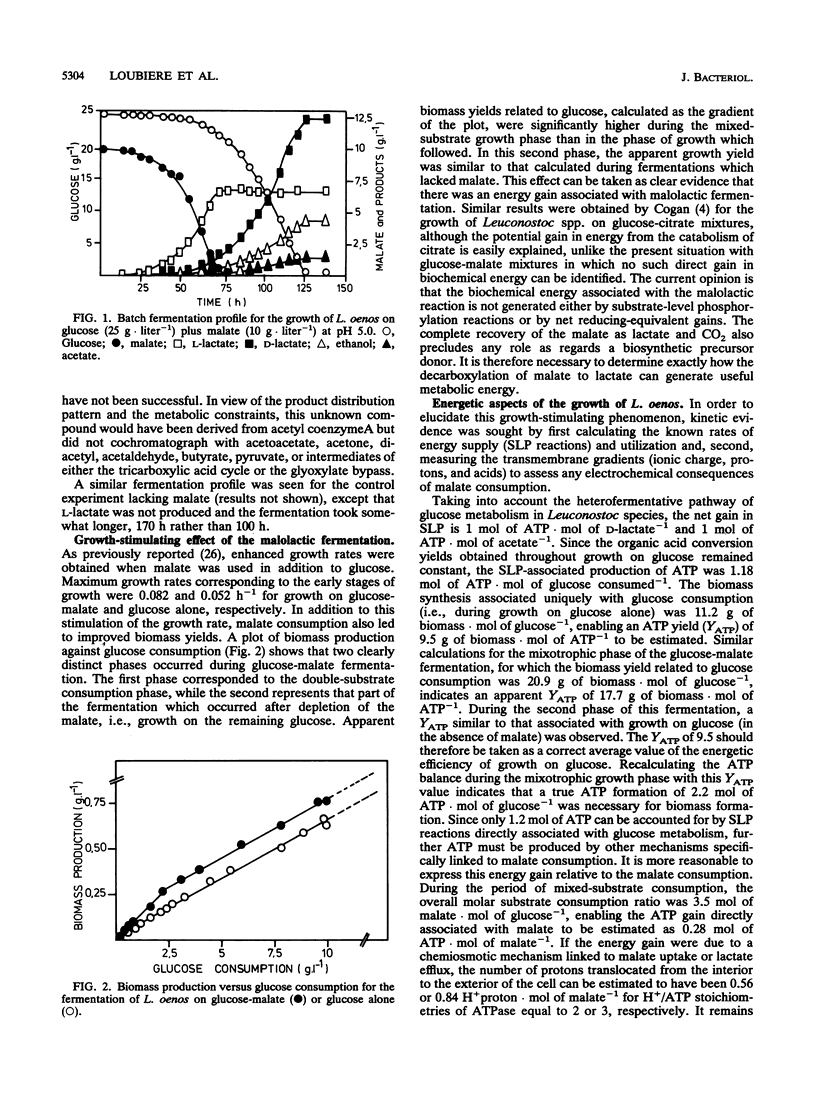
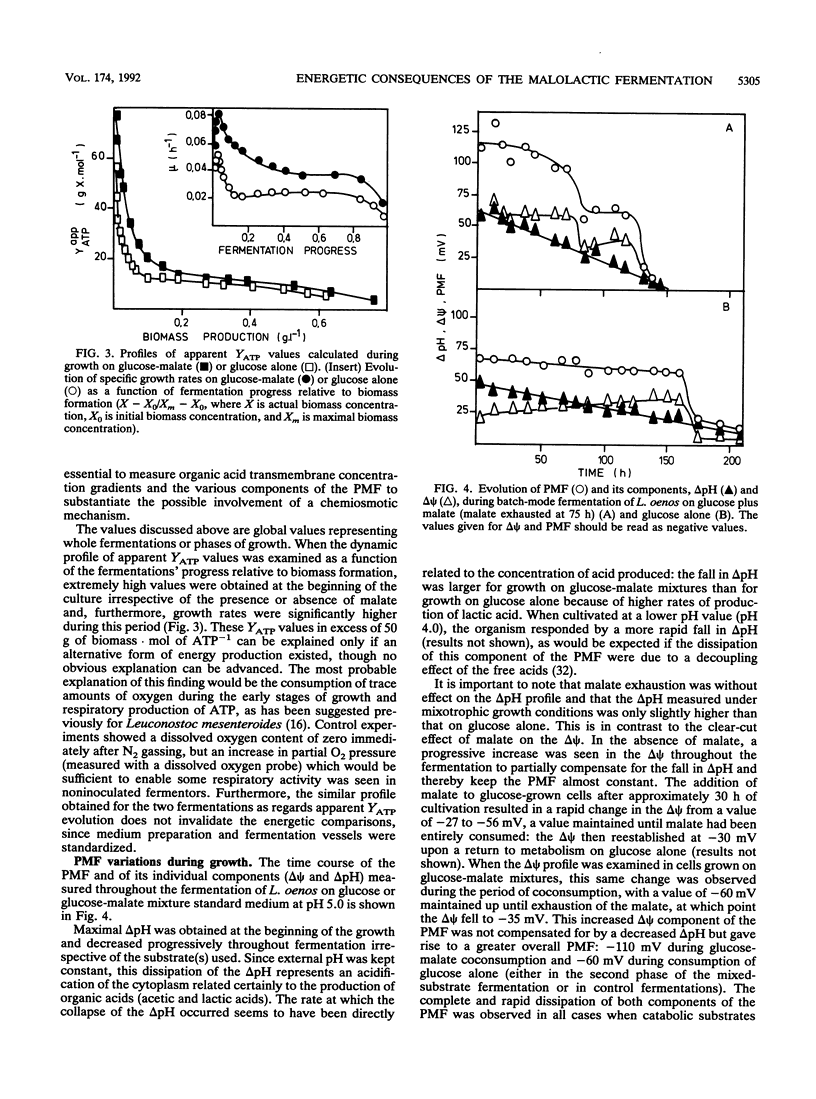
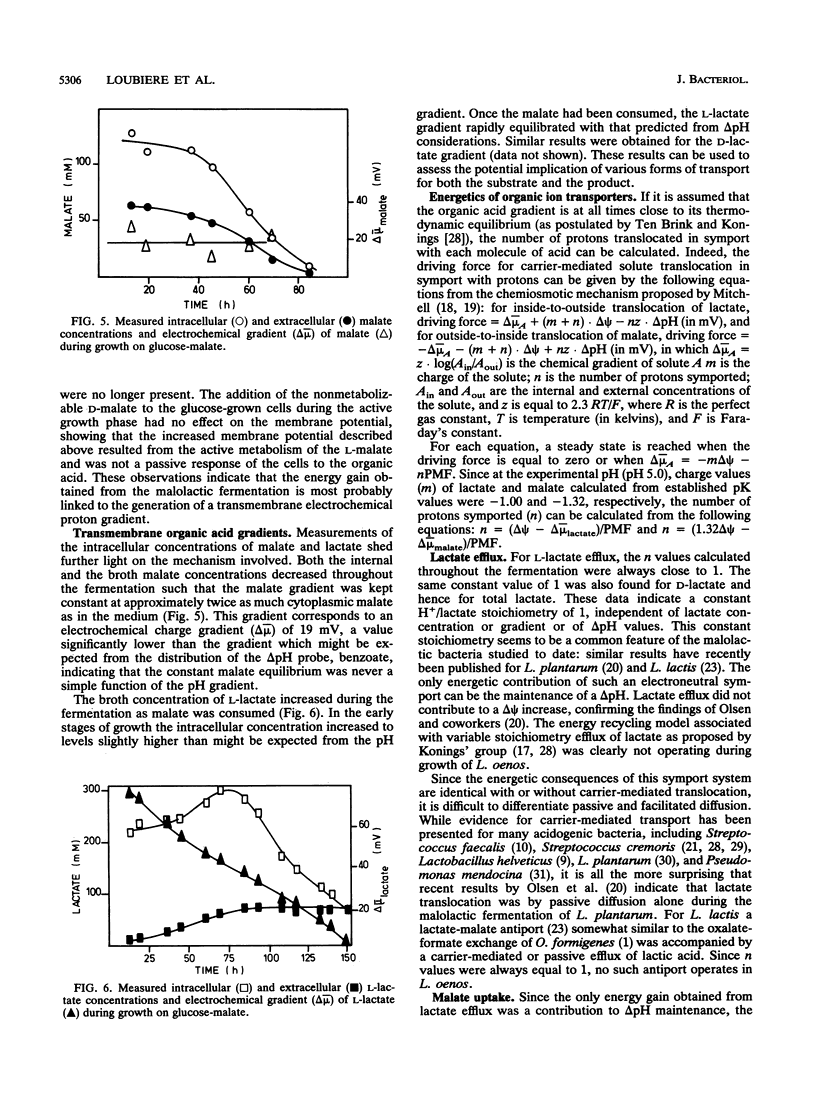
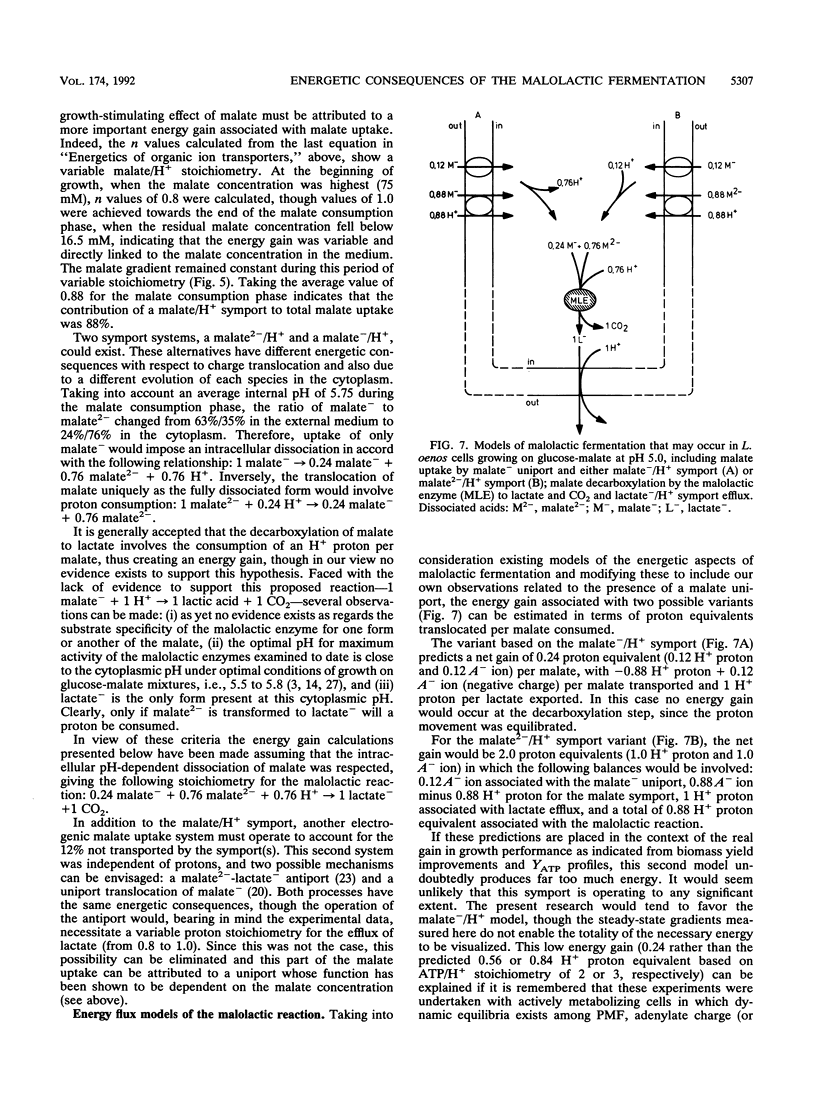
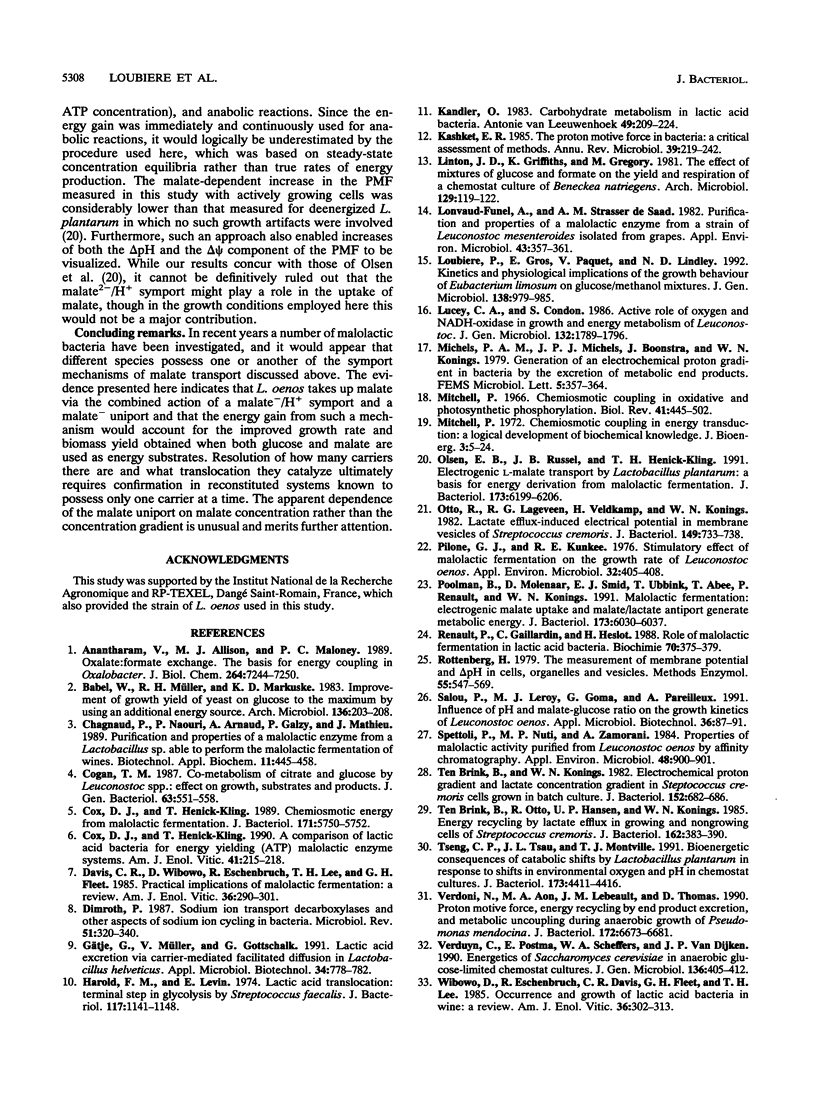
Selected References
These references are in PubMed. This may not be the complete list of references from this article.
- Anantharam V., Allison M. J., Maloney P. C. Oxalate:formate exchange. The basis for energy coupling in Oxalobacter. J Biol Chem. 1989 May 5;264(13):7244–7250. [PubMed] [Google Scholar]
- Cox D. J., Henick-Kling T. Chemiosmotic energy from malolactic fermentation. J Bacteriol. 1989 Oct;171(10):5750–5752. doi: 10.1128/jb.171.10.5750-5752.1989. [DOI] [PMC free article] [PubMed] [Google Scholar]
- Dimroth P. Sodium ion transport decarboxylases and other aspects of sodium ion cycling in bacteria. Microbiol Rev. 1987 Sep;51(3):320–340. doi: 10.1128/mr.51.3.320-340.1987. [DOI] [PMC free article] [PubMed] [Google Scholar]
- Harold F. M., Levin E. Lactic acid translocation: terminal step in glycolysis by Streptococcus faecalis. J Bacteriol. 1974 Mar;117(3):1141–1148. doi: 10.1128/jb.117.3.1141-1148.1974. [DOI] [PMC free article] [PubMed] [Google Scholar]
- Kandler O. Carbohydrate metabolism in lactic acid bacteria. Antonie Van Leeuwenhoek. 1983 Sep;49(3):209–224. doi: 10.1007/BF00399499. [DOI] [PubMed] [Google Scholar]
- Kashket E. R. The proton motive force in bacteria: a critical assessment of methods. Annu Rev Microbiol. 1985;39:219–242. doi: 10.1146/annurev.mi.39.100185.001251. [DOI] [PubMed] [Google Scholar]
- Lonvaud-Funel A., de Saad A. M. Purification and Properties of a Malolactic Enzyme from a Strain of Leuconostoc mesenteroides Isolated from Grapes. Appl Environ Microbiol. 1982 Feb;43(2):357–361. doi: 10.1128/aem.43.2.357-361.1982. [DOI] [PMC free article] [PubMed] [Google Scholar]
- Mitchell P. Chemiosmotic coupling in energy transduction: a logical development of biochemical knowledge. J Bioenerg. 1972 May;3(1):5–24. doi: 10.1007/BF01515993. [DOI] [PubMed] [Google Scholar]
- Mitchell P. Chemiosmotic coupling in oxidative and photosynthetic phosphorylation. Biol Rev Camb Philos Soc. 1966 Aug;41(3):445–502. doi: 10.1111/j.1469-185x.1966.tb01501.x. [DOI] [PubMed] [Google Scholar]
- Olsen E. B., Russell J. B., Henick-Kling T. Electrogenic L-malate transport by Lactobacillus plantarum: a basis for energy derivation from malolactic fermentation. J Bacteriol. 1991 Oct;173(19):6199–6206. doi: 10.1128/jb.173.19.6199-6206.1991. [DOI] [PMC free article] [PubMed] [Google Scholar]
- Otto R., Lageveen R. G., Veldkamp H., Konings W. N. Lactate efflux-induced electrical potential in membrane vesicles of Streptococcus cremoris. J Bacteriol. 1982 Feb;149(2):733–738. doi: 10.1128/jb.149.2.733-738.1982. [DOI] [PMC free article] [PubMed] [Google Scholar]
- Pilone G. J., Kunkee R. E. Stimulatory Effect of Malo-Lactic Fermentation on the Growth Rate of Leuconostoc oenos. Appl Environ Microbiol. 1976 Sep;32(3):405–408. doi: 10.1128/aem.32.3.405-408.1976. [DOI] [PMC free article] [PubMed] [Google Scholar]
- Poolman B., Molenaar D., Smid E. J., Ubbink T., Abee T., Renault P. P., Konings W. N. Malolactic fermentation: electrogenic malate uptake and malate/lactate antiport generate metabolic energy. J Bacteriol. 1991 Oct;173(19):6030–6037. doi: 10.1128/jb.173.19.6030-6037.1991. [DOI] [PMC free article] [PubMed] [Google Scholar]
- Renault P., Gaillardin C., Heslot H. Role of malolactic fermentation in lactic acid bacteria. Biochimie. 1988 Mar;70(3):375–379. doi: 10.1016/0300-9084(88)90210-6. [DOI] [PubMed] [Google Scholar]
- Rottenberg H. The measurement of membrane potential and deltapH in cells, organelles, and vesicles. Methods Enzymol. 1979;55:547–569. doi: 10.1016/0076-6879(79)55066-6. [DOI] [PubMed] [Google Scholar]
- Spettoli P., Nuti M. P., Zamorani A. Properties of Malolactic Activity Purified from Leuconostoc oenos ML34 by Affinity Chromatography. Appl Environ Microbiol. 1984 Oct;48(4):900–901. doi: 10.1128/aem.48.4.900-901.1984. [DOI] [PMC free article] [PubMed] [Google Scholar]
- Tseng C. P., Tsau J. L., Montville T. J. Bioenergetic consequences of catabolic shifts by Lactobacillus plantarum in response to shifts in environmental oxygen and pH in chemostat cultures. J Bacteriol. 1991 Jul;173(14):4411–4416. doi: 10.1128/jb.173.14.4411-4416.1991. [DOI] [PMC free article] [PubMed] [Google Scholar]
- Verdoni N., Aon M. A., Lebeault J. M., Thomas D. Proton motive force, energy recycling by end product excretion, and metabolic uncoupling during anaerobic growth of Pseudomonas mendocina. J Bacteriol. 1990 Dec;172(12):6673–6681. doi: 10.1128/jb.172.12.6673-6681.1990. [DOI] [PMC free article] [PubMed] [Google Scholar]
- Verduyn C., Postma E., Scheffers W. A., van Dijken J. P. Energetics of Saccharomyces cerevisiae in anaerobic glucose-limited chemostat cultures. J Gen Microbiol. 1990 Mar;136(3):405–412. doi: 10.1099/00221287-136-3-405. [DOI] [PubMed] [Google Scholar]
- ten Brink B., Konings W. N. Electrochemical proton gradient and lactate concentration gradient in Streptococcus cremoris cells grown in batch culture. J Bacteriol. 1982 Nov;152(2):682–686. doi: 10.1128/jb.152.2.682-686.1982. [DOI] [PMC free article] [PubMed] [Google Scholar]
- ten Brink B., Otto R., Hansen U. P., Konings W. N. Energy recycling by lactate efflux in growing and nongrowing cells of Streptococcus cremoris. J Bacteriol. 1985 Apr;162(1):383–390. doi: 10.1128/jb.162.1.383-390.1985. [DOI] [PMC free article] [PubMed] [Google Scholar]