Abstract
When exponentially growing hyphae of Neurospora crassa in aerated liquid cultures are filtered and the resulting mycelial mat is exposed to air, aerial hyphae develop and synchronous conidiation is obtained. The hyphae in direct contact with air adhere to each other within minutes and form aerial hyphae during the following 12 h; the hyphae which are not in direct contact with air do not adhere to each other and do not form aerial hyphae. Previous data indicated that oxidative stress was generated in the adhering hyphae; proteins and specific enzymes were found to be oxidatively modified and degraded. In this work, we report a dramatic fall in the reduced-to-oxidized ratio of NAD and NADP coenzymes during the first 6 min of exposure to air. This drop did not occur in a mycelial mat exposed to a N2-enriched atmosphere. Adding a carbon source to the mycelial mat did not abolish the loss of NAD(P)-reducing power. After the initial fall, the reducing levels of the coenzymes returned to the starting value in about 30 min. A peak of extracellular glutathione disulfide occurred simultaneously with the loss of NAD(P)-reducing power. The reducing power loss and the excretion of glutathione disulfide are thought to be consequences of a hyperoxidant state; the adhesion of hyphae is thought to be a response to the hyperoxidant state.
Full text
PDF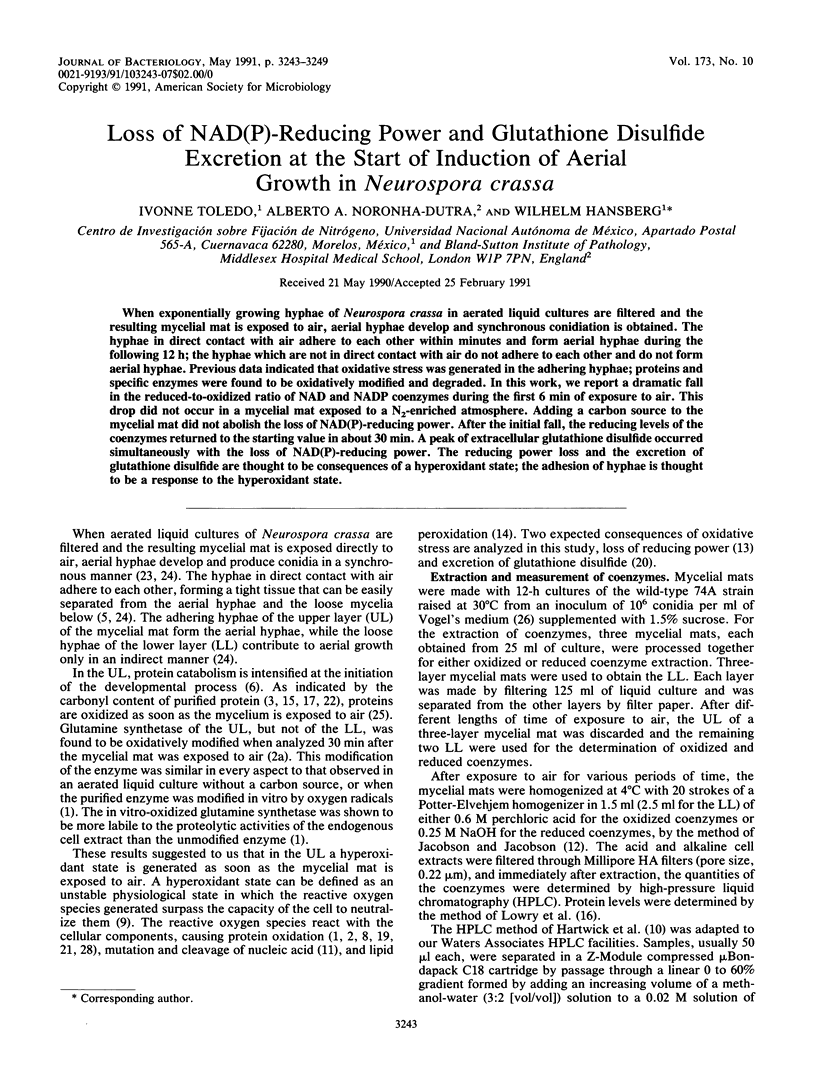
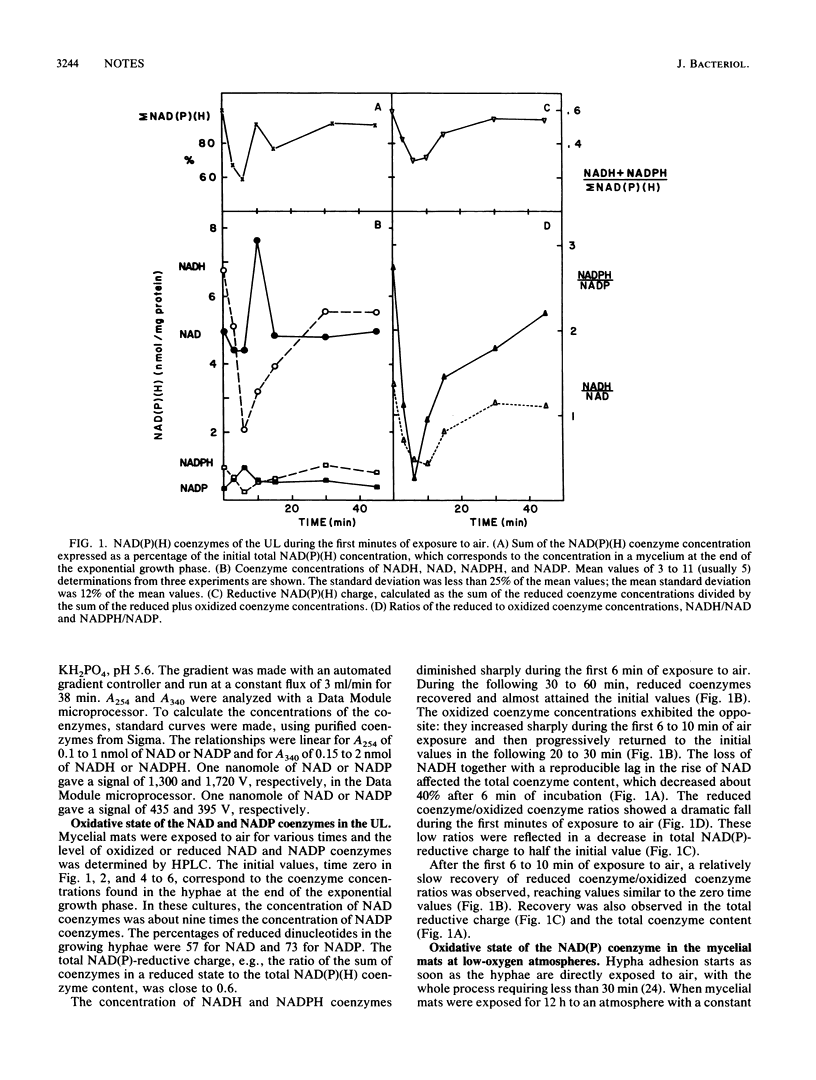
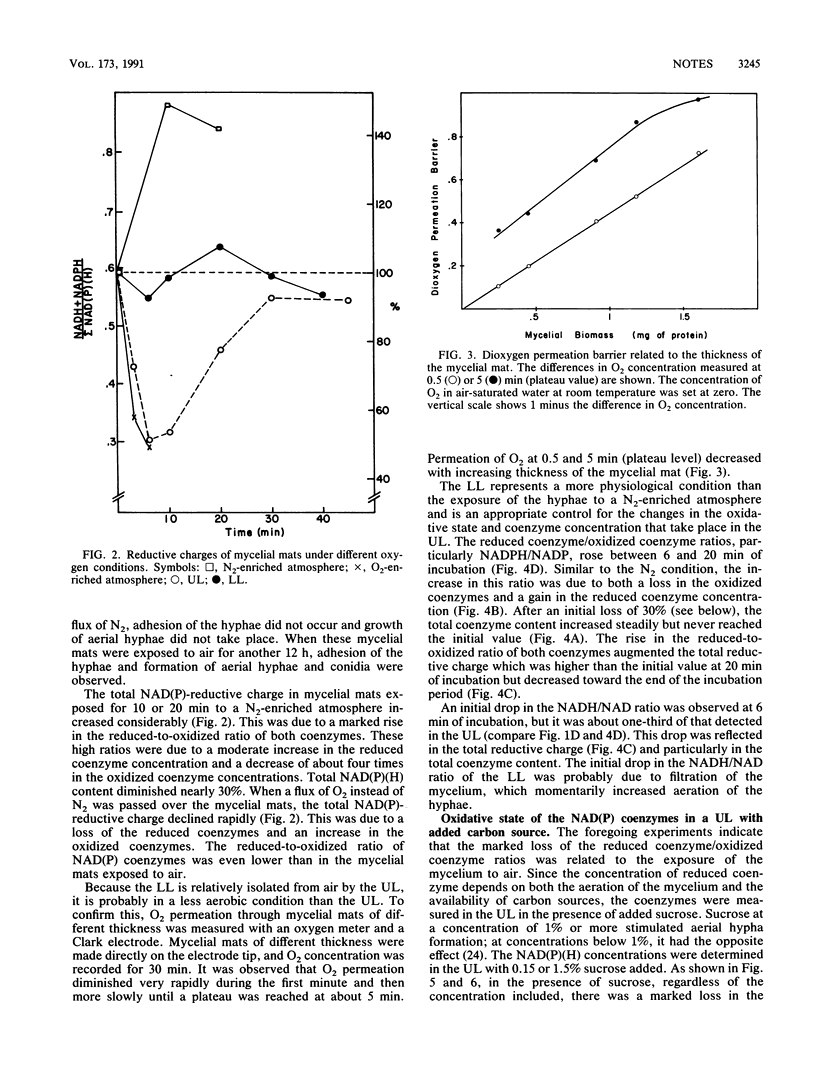
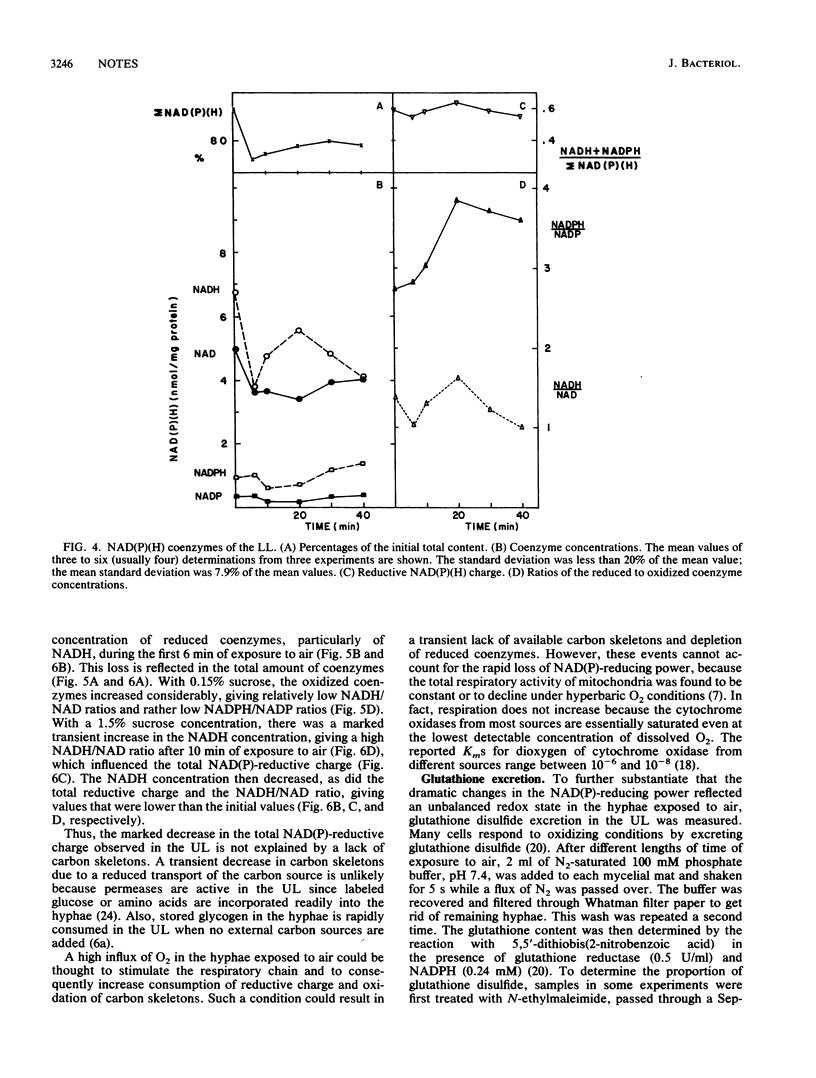
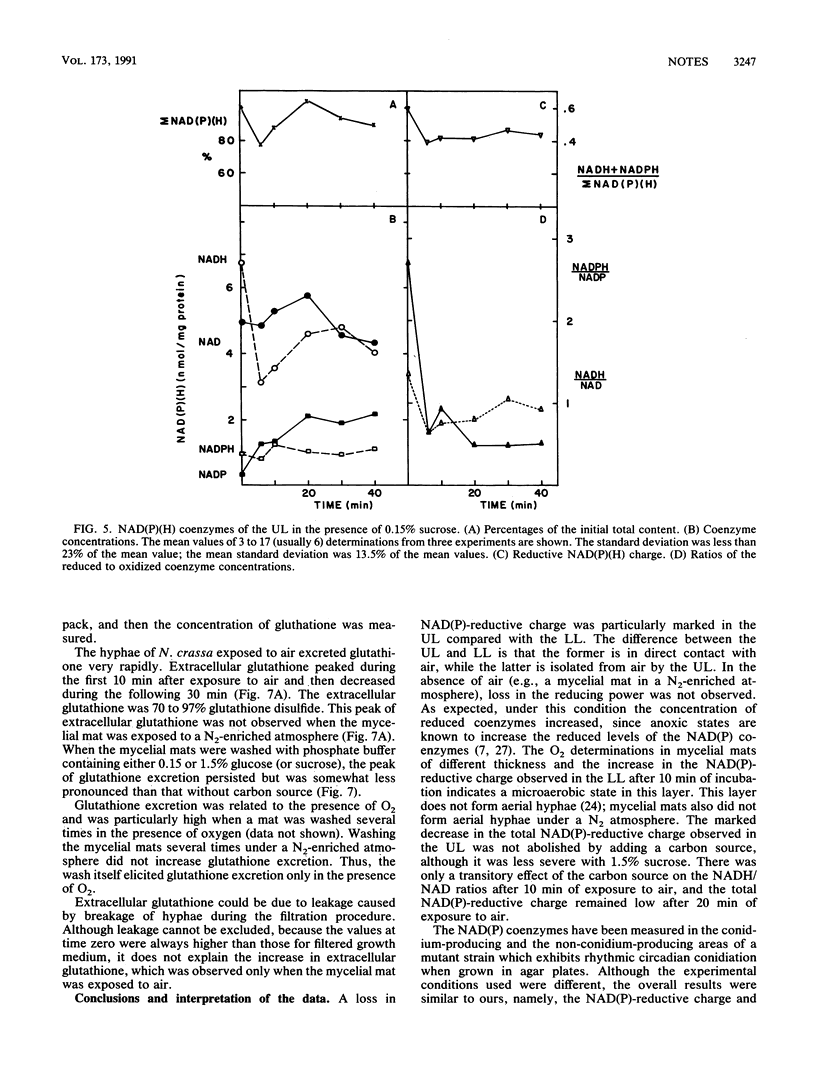
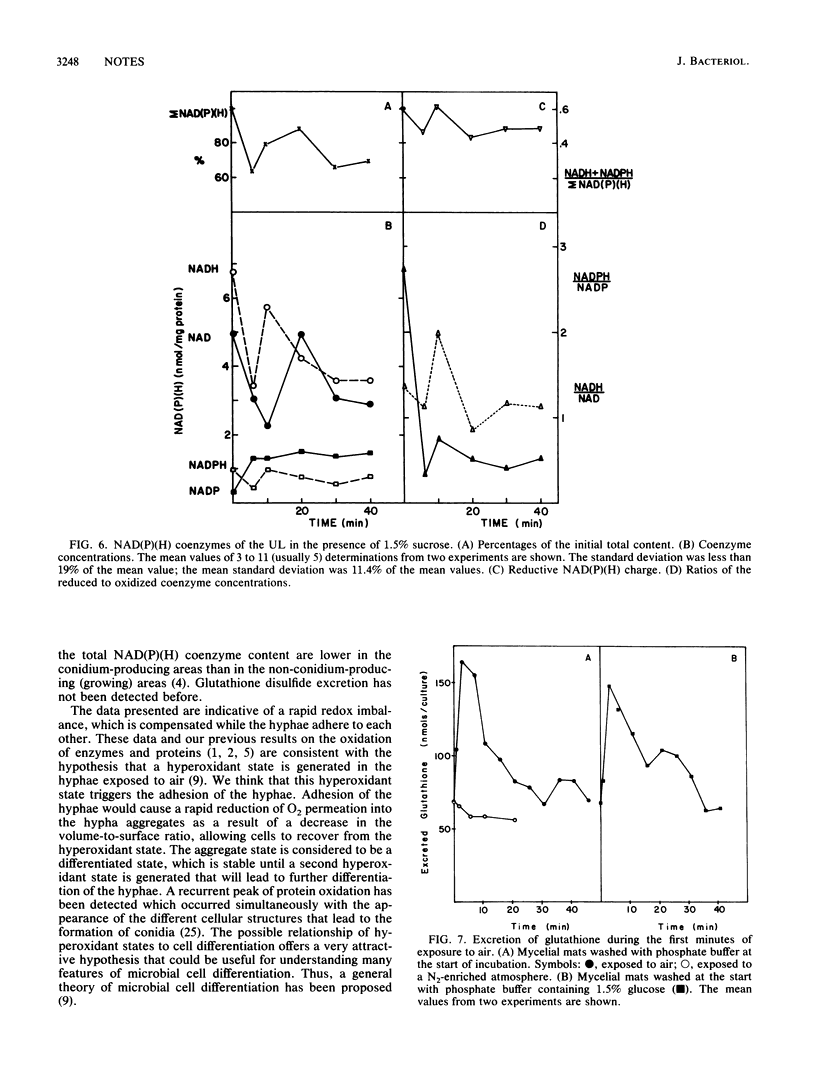
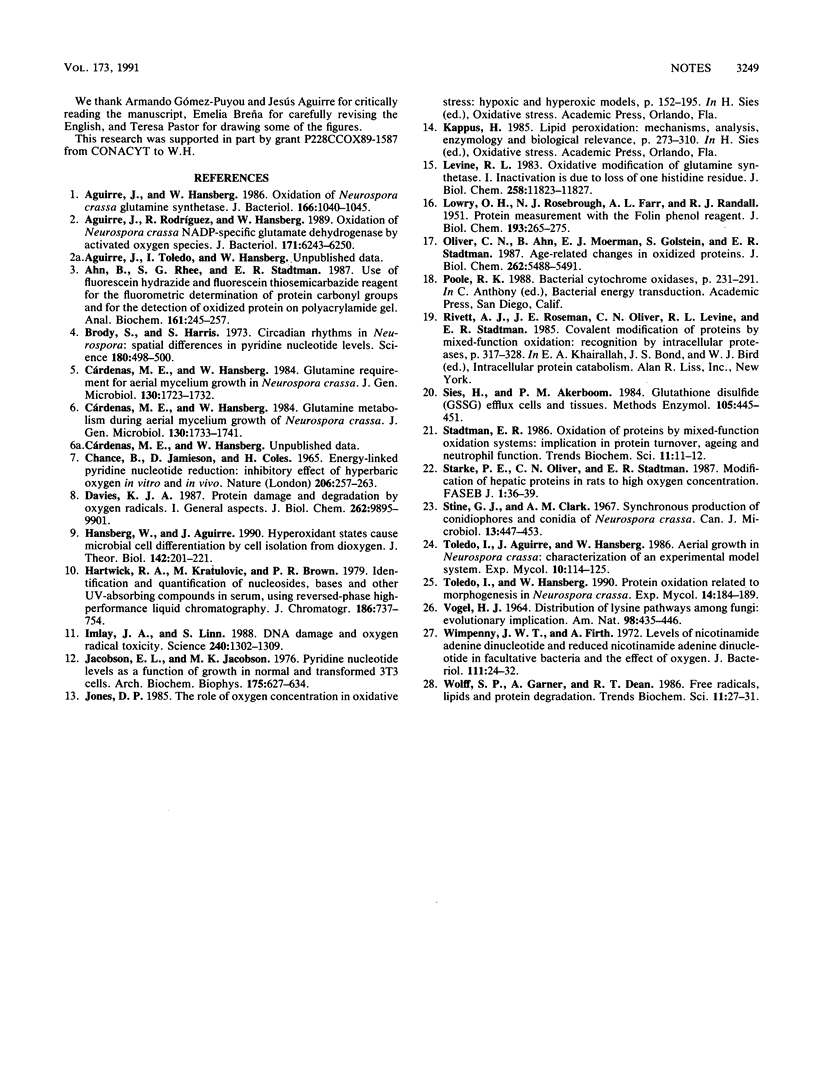
Selected References
These references are in PubMed. This may not be the complete list of references from this article.
- Aguirre J., Hansberg W. Oxidation of Neurospora crassa glutamine synthetase. J Bacteriol. 1986 Jun;166(3):1040–1045. doi: 10.1128/jb.166.3.1040-1045.1986. [DOI] [PMC free article] [PubMed] [Google Scholar]
- Aguirre J., Rodríguez R., Hansberg W. Oxidation of Neurospora crassa NADP-specific glutamate dehydrogenase by activated oxygen species. J Bacteriol. 1989 Nov;171(11):6243–6250. doi: 10.1128/jb.171.11.6243-6250.1989. [DOI] [PMC free article] [PubMed] [Google Scholar]
- Ahn B., Rhee S. G., Stadtman E. R. Use of fluorescein hydrazide and fluorescein thiosemicarbazide reagents for the fluorometric determination of protein carbonyl groups and for the detection of oxidized protein on polyacrylamide gels. Anal Biochem. 1987 Mar;161(2):245–257. doi: 10.1016/0003-2697(87)90448-9. [DOI] [PubMed] [Google Scholar]
- Brody S., Harris S. Circadian rhythms in neurospora: spatial differences in pyridine nucleotide levels. Science. 1973 May 4;180(4085):498–500. doi: 10.1126/science.180.4085.498. [DOI] [PubMed] [Google Scholar]
- Chance B., Jamieson D., Coles H. Energy-linked pyridine nucleotide reduction: inhibitory effects of hyperbaric oxygen in vitro and in vivo. Nature. 1965 Apr 17;206(981):257–263. doi: 10.1038/206257a0. [DOI] [PubMed] [Google Scholar]
- Davies K. J. Protein damage and degradation by oxygen radicals. I. general aspects. J Biol Chem. 1987 Jul 15;262(20):9895–9901. [PubMed] [Google Scholar]
- Hansberg W., Aguirre J. Hyperoxidant states cause microbial cell differentiation by cell isolation from dioxygen. J Theor Biol. 1990 Jan 23;142(2):201–221. doi: 10.1016/s0022-5193(05)80222-x. [DOI] [PubMed] [Google Scholar]
- Imlay J. A., Linn S. DNA damage and oxygen radical toxicity. Science. 1988 Jun 3;240(4857):1302–1309. doi: 10.1126/science.3287616. [DOI] [PubMed] [Google Scholar]
- Jacobson E. L., Jacobson M. K. Pyridine nucleotide levels as a function of growth in normal and transformed 3T3 cells. Arch Biochem Biophys. 1976 Aug;175(2):627–634. doi: 10.1016/0003-9861(76)90553-1. [DOI] [PubMed] [Google Scholar]
- LOWRY O. H., ROSEBROUGH N. J., FARR A. L., RANDALL R. J. Protein measurement with the Folin phenol reagent. J Biol Chem. 1951 Nov;193(1):265–275. [PubMed] [Google Scholar]
- Levine R. L. Oxidative modification of glutamine synthetase. I. Inactivation is due to loss of one histidine residue. J Biol Chem. 1983 Oct 10;258(19):11823–11827. [PubMed] [Google Scholar]
- Oliver C. N., Ahn B. W., Moerman E. J., Goldstein S., Stadtman E. R. Age-related changes in oxidized proteins. J Biol Chem. 1987 Apr 25;262(12):5488–5491. [PubMed] [Google Scholar]
- Rivett A. J., Roseman J. E., Oliver C. N., Levine R. L., Stadtman E. R. Covalent modification of proteins by mixed-function oxidation: recognition by intracellular proteases. Prog Clin Biol Res. 1985;180:317–328. [PubMed] [Google Scholar]
- Sies H., Akerboom T. P. Glutathione disulfide (GSSG) efflux from cells and tissues. Methods Enzymol. 1984;105:445–451. doi: 10.1016/s0076-6879(84)05062-x. [DOI] [PubMed] [Google Scholar]
- Starke P. E., Oliver C. N., Stadtman E. R. Modification of hepatic proteins in rats exposed to high oxygen concentration. FASEB J. 1987 Jul;1(1):36–39. doi: 10.1096/fasebj.1.1.2886388. [DOI] [PubMed] [Google Scholar]
- Stine G. J., Clark A. M. Synchronous production of conidiophores and conidia of Neurospora crassa. Can J Microbiol. 1967 May;13(5):447–453. doi: 10.1139/m67-060. [DOI] [PubMed] [Google Scholar]
- Wimpenny J. W., Firth A. Levels of nicotinamide adenine dinucleotide and reduced nicotinamide adenine dinucleotide in facultative bacteria and the effect of oxygen. J Bacteriol. 1972 Jul;111(1):24–32. doi: 10.1128/jb.111.1.24-32.1972. [DOI] [PMC free article] [PubMed] [Google Scholar]