Abstract
L-Malate transport in Lactobacillus plantarum was inducible, and the pH optimum was 4.5. Malate uptake could be driven by an artificial proton gradient (delta pH) or an electroneutral lactate efflux. Because L-lactate efflux was unable to drive L-malate transport in the absence of a delta pH, it did not appear that the carrier was a malate-lactate exchanger. The kinetics of malate transport were, however, biphasic, suggesting that the external malate concentration was also serving as a driving force for low-affinity malate uptake. Because the electrical potential (delta psi, inside negative) inhibited malate transport, it appeared that the malate transport-lactate efflux couple was electrogenic (net negative) at high concentrations of malate. De-energized cells that were provided with malate only generated a large proton motive force (greater than 100 mV) when the malate concentration was greater than 5 mM, and malate only caused an increase in cell yield (glucose-limited chemostats) when malate accumulated in the culture vessel. The use of the malate gradient to drive malate transport (facilitated diffusion) explains how L. plantarum derives energy from malolactic fermentation, a process which does not involve substrate-level phosphorylation.
Full text
PDF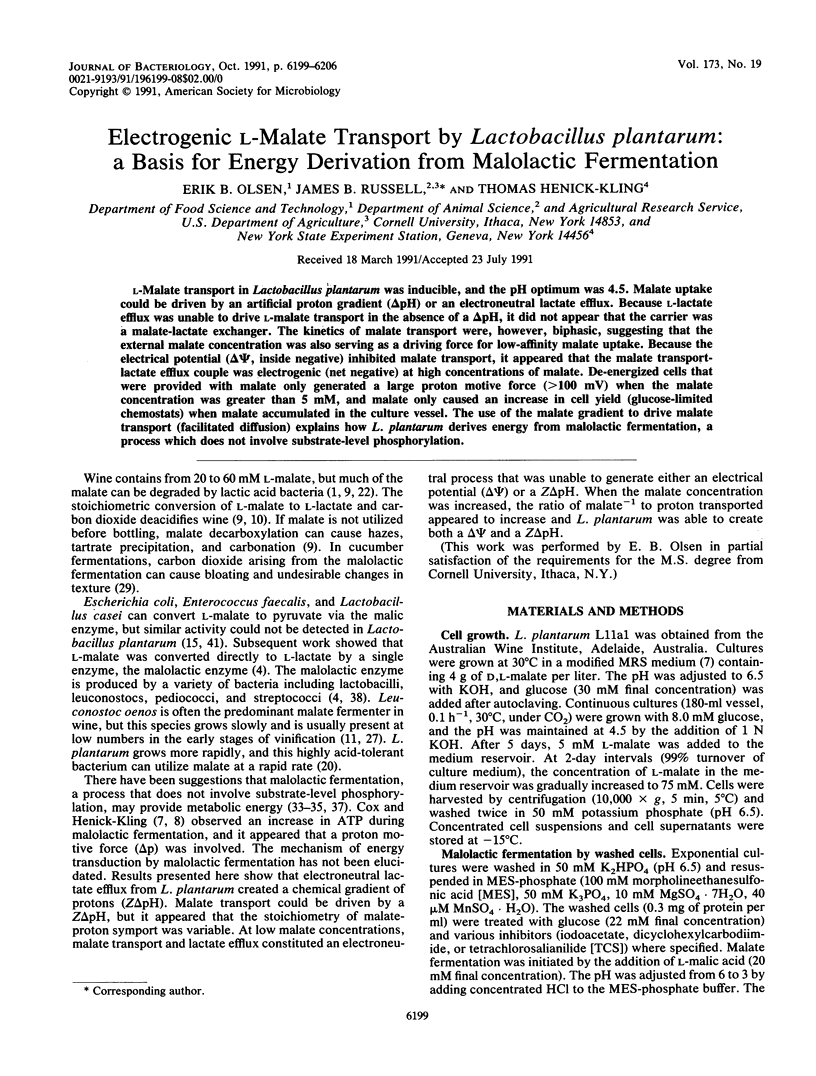
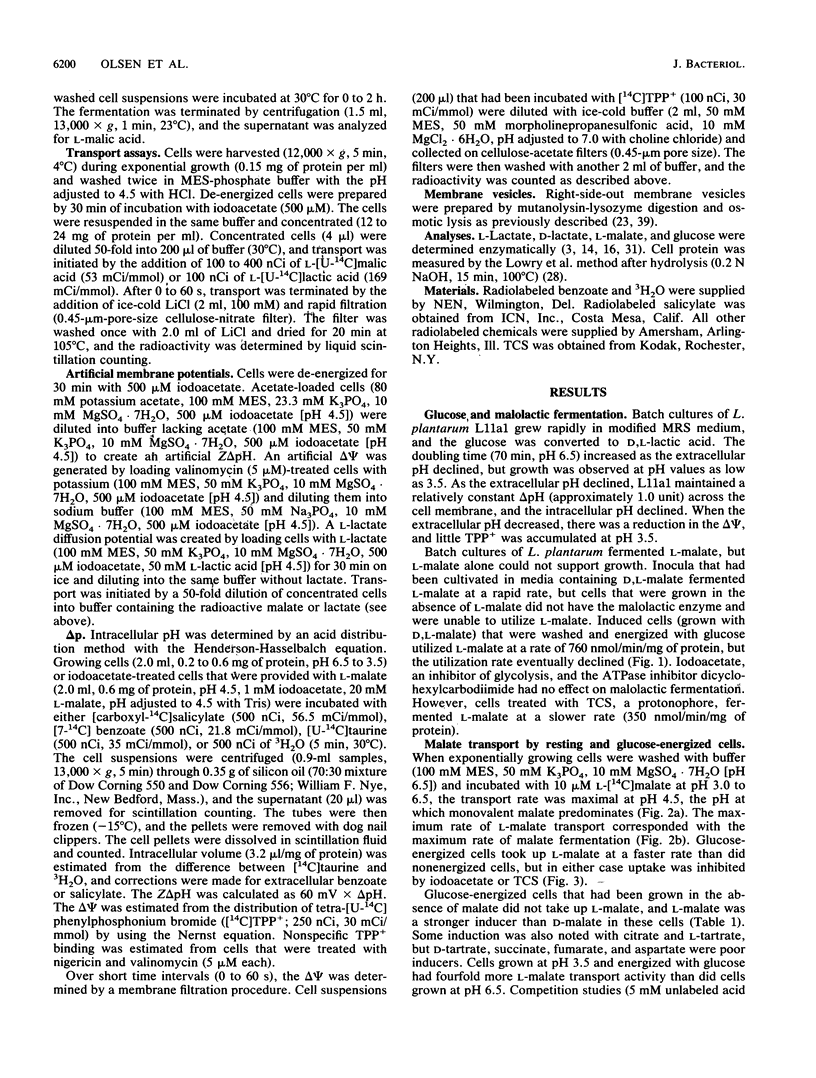
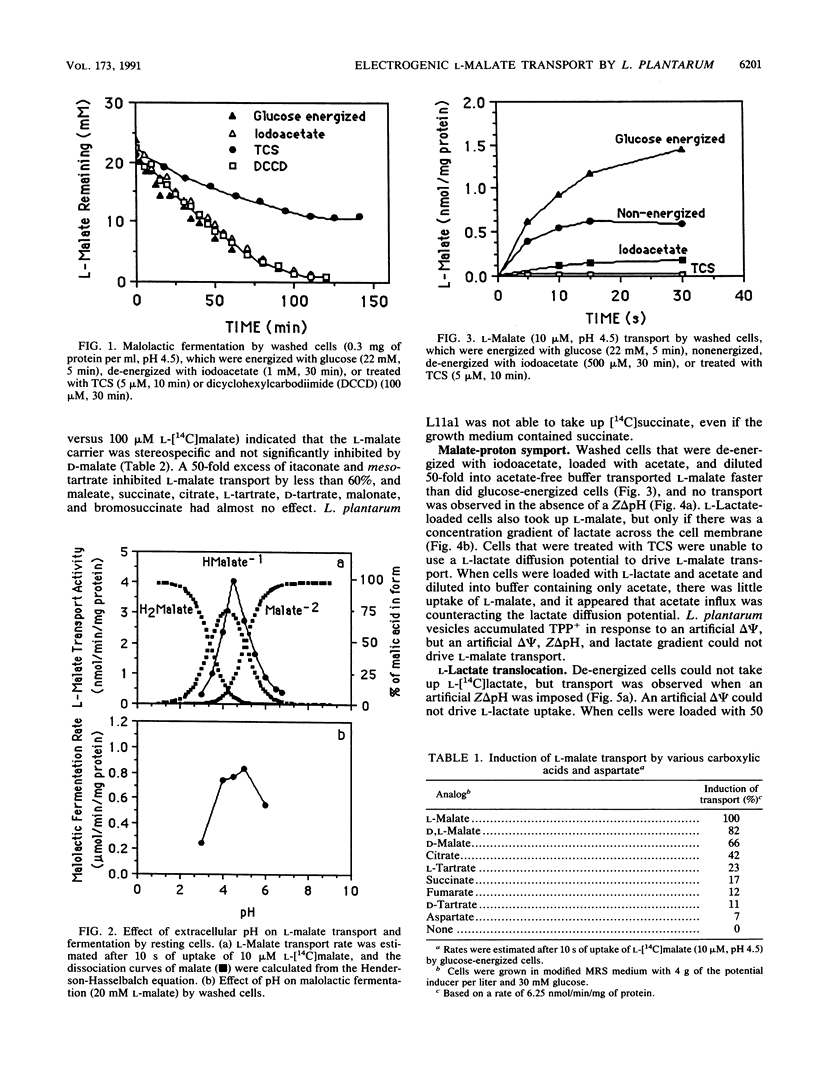
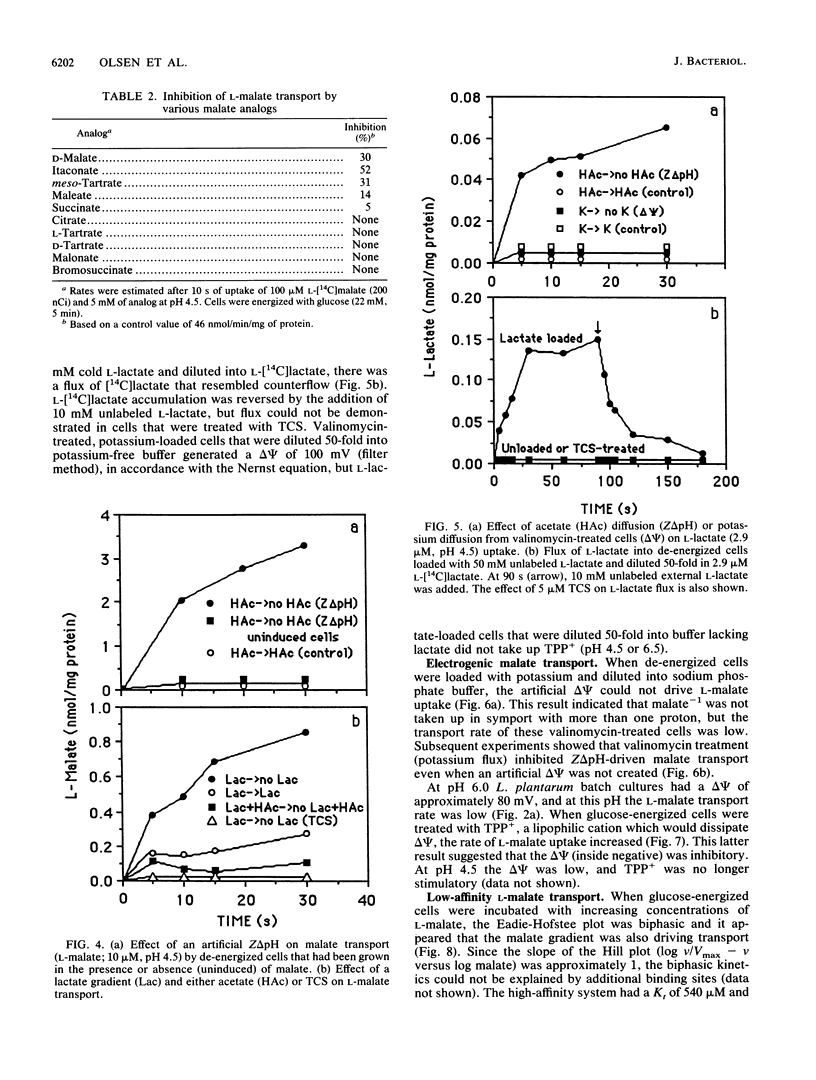
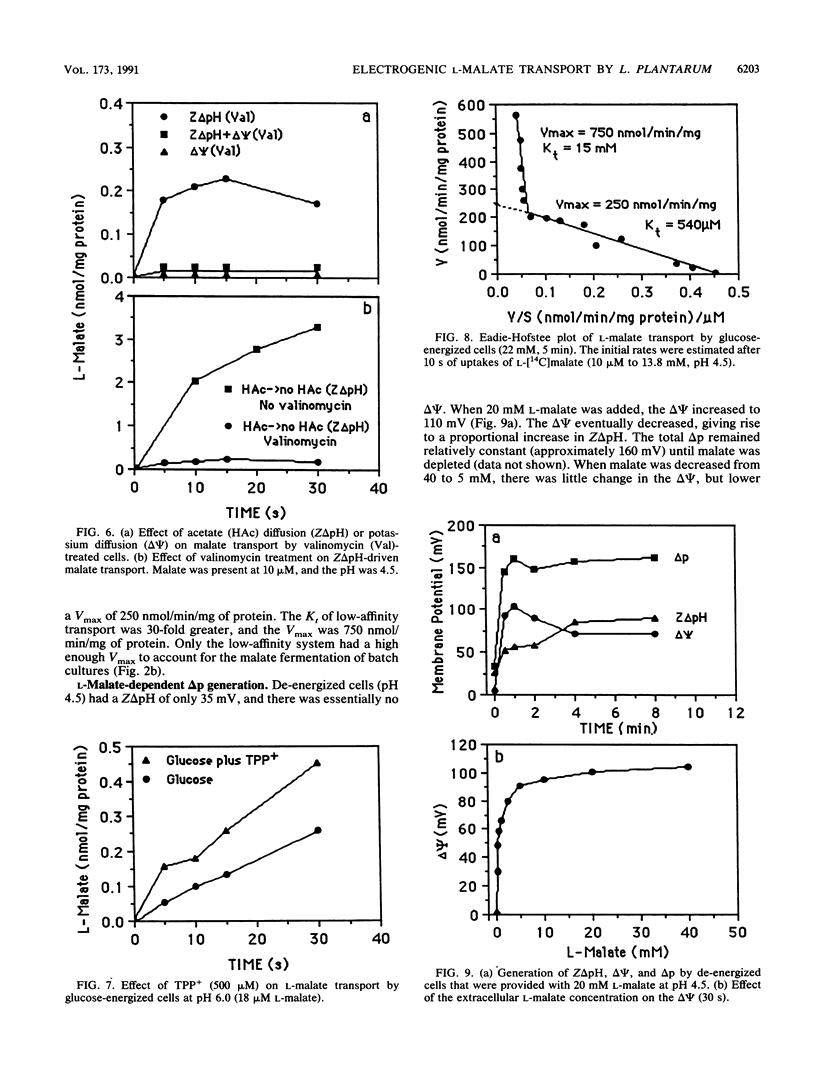
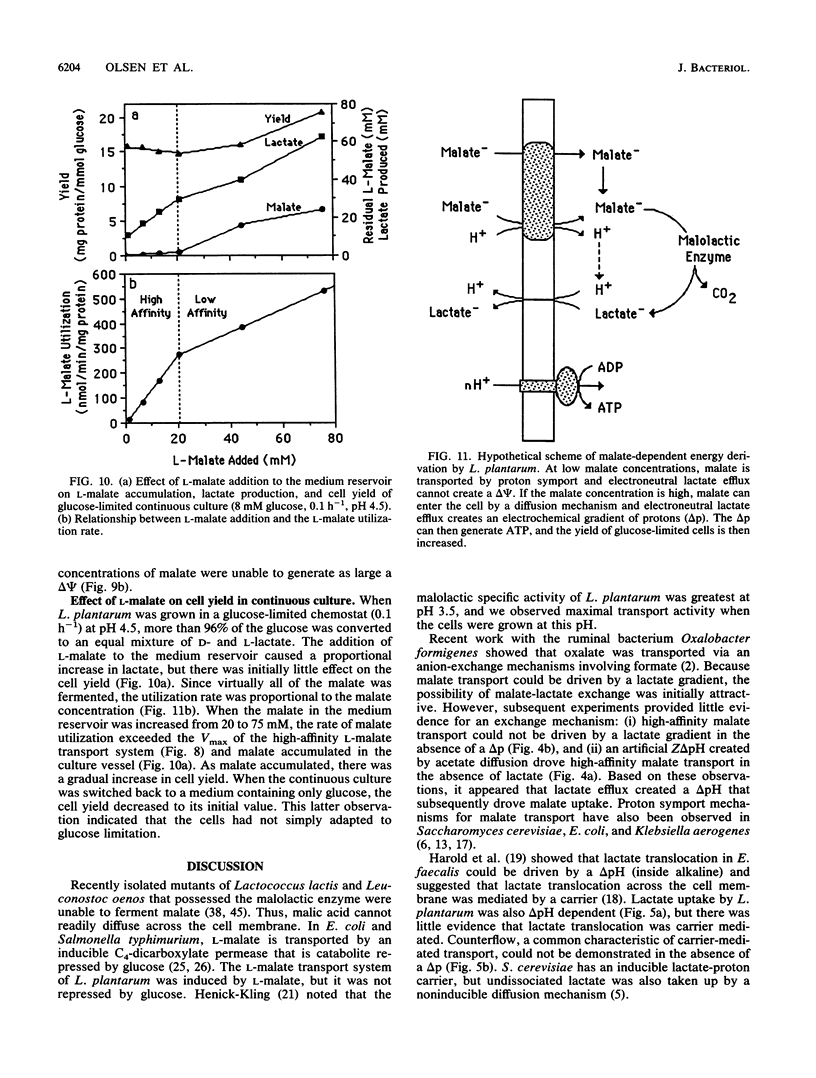
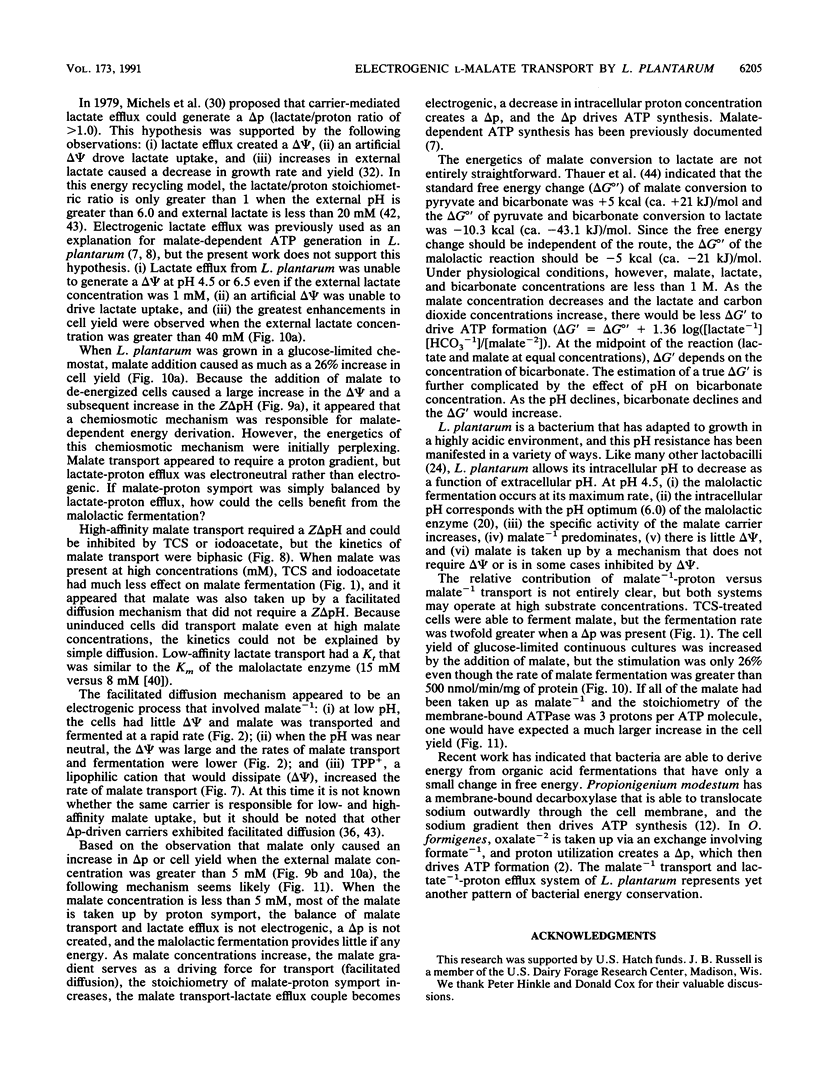
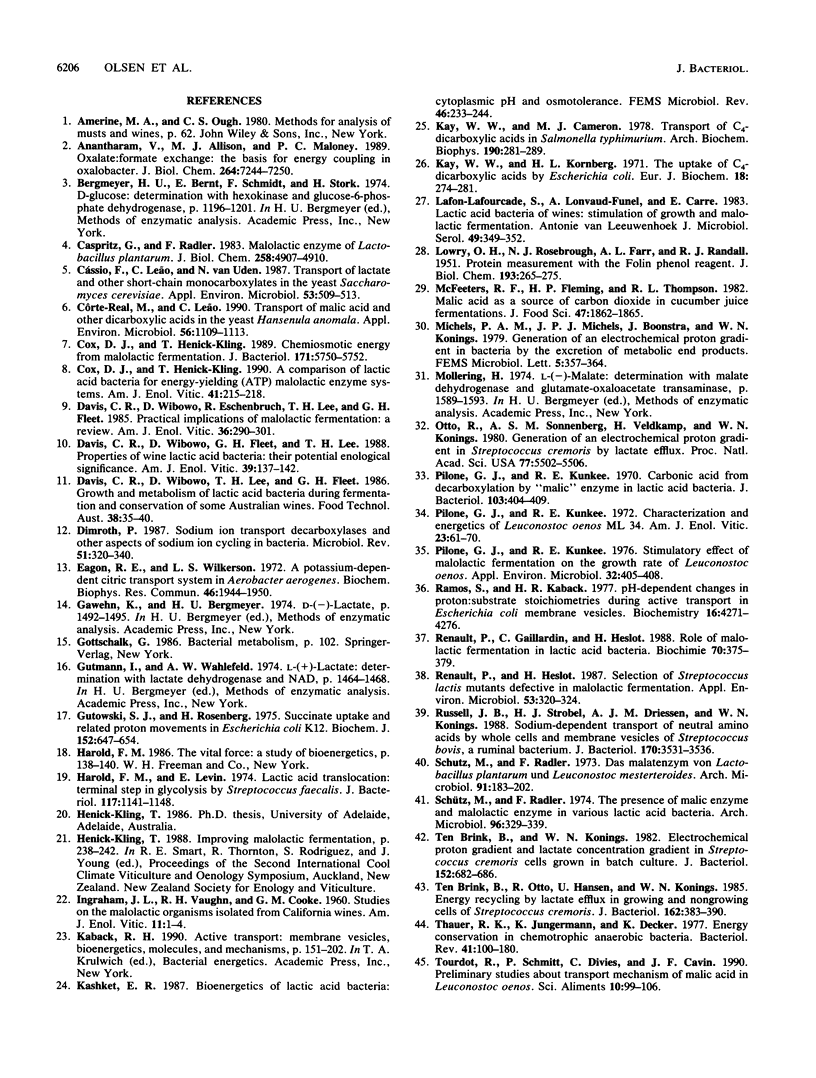
Selected References
These references are in PubMed. This may not be the complete list of references from this article.
- Anantharam V., Allison M. J., Maloney P. C. Oxalate:formate exchange. The basis for energy coupling in Oxalobacter. J Biol Chem. 1989 May 5;264(13):7244–7250. [PubMed] [Google Scholar]
- Caspritz G., Radler F. Malolactic enzyme of Lactobacillus plantarum. Purification, properties, and distribution among bacteria. J Biol Chem. 1983 Apr 25;258(8):4907–4910. [PubMed] [Google Scholar]
- Cox D. J., Henick-Kling T. Chemiosmotic energy from malolactic fermentation. J Bacteriol. 1989 Oct;171(10):5750–5752. doi: 10.1128/jb.171.10.5750-5752.1989. [DOI] [PMC free article] [PubMed] [Google Scholar]
- Cássio F., Leão C., van Uden N. Transport of lactate and other short-chain monocarboxylates in the yeast Saccharomyces cerevisiae. Appl Environ Microbiol. 1987 Mar;53(3):509–513. doi: 10.1128/aem.53.3.509-513.1987. [DOI] [PMC free article] [PubMed] [Google Scholar]
- Côrte-Real M., Leão C. Transport of malic acid and other dicarboxylic acids in the yeast Hansenula anomala. Appl Environ Microbiol. 1990 Apr;56(4):1109–1113. doi: 10.1128/aem.56.4.1109-1113.1990. [DOI] [PMC free article] [PubMed] [Google Scholar]
- Dimroth P. Sodium ion transport decarboxylases and other aspects of sodium ion cycling in bacteria. Microbiol Rev. 1987 Sep;51(3):320–340. doi: 10.1128/mr.51.3.320-340.1987. [DOI] [PMC free article] [PubMed] [Google Scholar]
- Eagon R. G., Wilkerson L. S. A potassium-dependent citric acid transport system in Aerobacter aerogenes. Biochem Biophys Res Commun. 1972 Mar 10;46(5):1944–1950. doi: 10.1016/0006-291x(72)90074-5. [DOI] [PubMed] [Google Scholar]
- Gutowski S. J., Rosenberg H. Succinate uptake and related proton movements in Escherichia coli K12. Biochem J. 1975 Dec;152(3):647–654. doi: 10.1042/bj1520647. [DOI] [PMC free article] [PubMed] [Google Scholar]
- Harold F. M., Levin E. Lactic acid translocation: terminal step in glycolysis by Streptococcus faecalis. J Bacteriol. 1974 Mar;117(3):1141–1148. doi: 10.1128/jb.117.3.1141-1148.1974. [DOI] [PMC free article] [PubMed] [Google Scholar]
- Kay W. W., Cameron M. J. Transport of C4-dicarboxylic acids in salmonella typhimurium. Arch Biochem Biophys. 1978 Sep;190(1):281–289. doi: 10.1016/0003-9861(78)90277-1. [DOI] [PubMed] [Google Scholar]
- Kay W. W., Kornberg H. L. The uptake of C4-dicarboxylic acids by Escherichia coli. Eur J Biochem. 1971 Jan;18(2):274–281. doi: 10.1111/j.1432-1033.1971.tb01240.x. [DOI] [PubMed] [Google Scholar]
- LOWRY O. H., ROSEBROUGH N. J., FARR A. L., RANDALL R. J. Protein measurement with the Folin phenol reagent. J Biol Chem. 1951 Nov;193(1):265–275. [PubMed] [Google Scholar]
- Lafon-Lafourcade S., Lonvaud-Funel A., Carre E. Lactic acid bacteria of wines: stimulation of growth and malolactic fermentation. Antonie Van Leeuwenhoek. 1983 Sep;49(3):349–352. doi: 10.1007/BF00399509. [DOI] [PubMed] [Google Scholar]
- Otto R., Sonnenberg A. S., Veldkamp H., Konings W. N. Generation of an electrochemical proton gradient in Streptococcus cremoris by lactate efflux. Proc Natl Acad Sci U S A. 1980 Sep;77(9):5502–5506. doi: 10.1073/pnas.77.9.5502. [DOI] [PMC free article] [PubMed] [Google Scholar]
- Pilone G. J., Kunkee R. E. Carbonic acid from decarboxylation by "malic" enzyme in lactic acid bacteria. J Bacteriol. 1970 Aug;103(2):404–409. doi: 10.1128/jb.103.2.404-409.1970. [DOI] [PMC free article] [PubMed] [Google Scholar]
- Pilone G. J., Kunkee R. E. Stimulatory Effect of Malo-Lactic Fermentation on the Growth Rate of Leuconostoc oenos. Appl Environ Microbiol. 1976 Sep;32(3):405–408. doi: 10.1128/aem.32.3.405-408.1976. [DOI] [PMC free article] [PubMed] [Google Scholar]
- Renault P. P., Heslot H. Selection of Streptococcus lactis Mutants Defective in Malolactic Fermentation. Appl Environ Microbiol. 1987 Feb;53(2):320–324. doi: 10.1128/aem.53.2.320-324.1987. [DOI] [PMC free article] [PubMed] [Google Scholar]
- Renault P., Gaillardin C., Heslot H. Role of malolactic fermentation in lactic acid bacteria. Biochimie. 1988 Mar;70(3):375–379. doi: 10.1016/0300-9084(88)90210-6. [DOI] [PubMed] [Google Scholar]
- Russell J. B., Strobel H. J., Driessen A. J., Konings W. N. Sodium-dependent transport of neutral amino acids by whole cells and membrane vesicles of Streptococcus bovis, a ruminal bacterium. J Bacteriol. 1988 Aug;170(8):3531–3536. doi: 10.1128/jb.170.8.3531-3536.1988. [DOI] [PMC free article] [PubMed] [Google Scholar]
- Schütz M., Radler F. Das "Malatenzym" von Lactobacillus plantarum und Leuconostoc mesenteroides. Arch Mikrobiol. 1973 Jun 6;91(3):183–202. doi: 10.1007/BF00408907. [DOI] [PubMed] [Google Scholar]
- Schütz M., Radler F. Das Vorkommen von Malatenzym und Malo-Lactat-Enzym bei verschiedenen Milchsäurebakterien. Arch Mikrobiol. 1974 Mar 28;96(4):329–339. [PubMed] [Google Scholar]
- Thauer R. K., Jungermann K., Decker K. Energy conservation in chemotrophic anaerobic bacteria. Bacteriol Rev. 1977 Mar;41(1):100–180. doi: 10.1128/br.41.1.100-180.1977. [DOI] [PMC free article] [PubMed] [Google Scholar]
- ten Brink B., Konings W. N. Electrochemical proton gradient and lactate concentration gradient in Streptococcus cremoris cells grown in batch culture. J Bacteriol. 1982 Nov;152(2):682–686. doi: 10.1128/jb.152.2.682-686.1982. [DOI] [PMC free article] [PubMed] [Google Scholar]
- ten Brink B., Otto R., Hansen U. P., Konings W. N. Energy recycling by lactate efflux in growing and nongrowing cells of Streptococcus cremoris. J Bacteriol. 1985 Apr;162(1):383–390. doi: 10.1128/jb.162.1.383-390.1985. [DOI] [PMC free article] [PubMed] [Google Scholar]