Abstract
When Selenomonas ruminantium HD4 was grown in a chemostat, maximal succinate production and the highest molar growth yield values were both observed at a dilution rate of roughly 0.2 h-1. To determine the possible relationship between succinate efflux and high molar growth yields, the generation of a membrane potential by succinate efflux was studied in whole cells and vesicles (inside-out and right-side-out) prepared from S. ruminantium. Washed whole cells took up succinate in the absence of an exogenous energy supply; uptake was completely abolished by brief treatment with dinitrophenol or with nigericin and valinomycin. High levels of sodium ions (with respect to the intracellular sodium concentration in the assay buffer had a stimulatory effect on succinate uptake. When succinate was added to inside-out vesicles, a membrane potential (inside positive) was generated, as indicated by fluorescence quenching of the anionic lipophilic dye Oxonol V. Fluorescence quenching was sensitive to uncoupling by gramicidin D but only partially sensitive to the uncoupler carbonyl cyanide-p-trifluoromethoxyphenylhydrazone. In right-side-out vesicles, succinate uptake could be driven by an artificially imposed sodium gradient but not by a potassium diffusion potential; imposition of both a sodium gradient and potassium diffusion potential resulted in improved succinate uptake. The generation of a membrane potential (inside negative) upon succinate efflux was demonstrated directly in right-side-out vesicles when succinate-loaded vesicles were diluted into succinate-free buffer, and the lipophilic cationic probe tetraphenylphosphonium accumulated in the vesicles. Results indicate that an electrogenic succinate-sodium symporter is present in S. ruminantium. Transport of succinate out of the cell via the symporter might be responsible for the high molar growth yields obtained by this organism when it is grown at dilution rates where maximal succinate production occurs.
Full text
PDF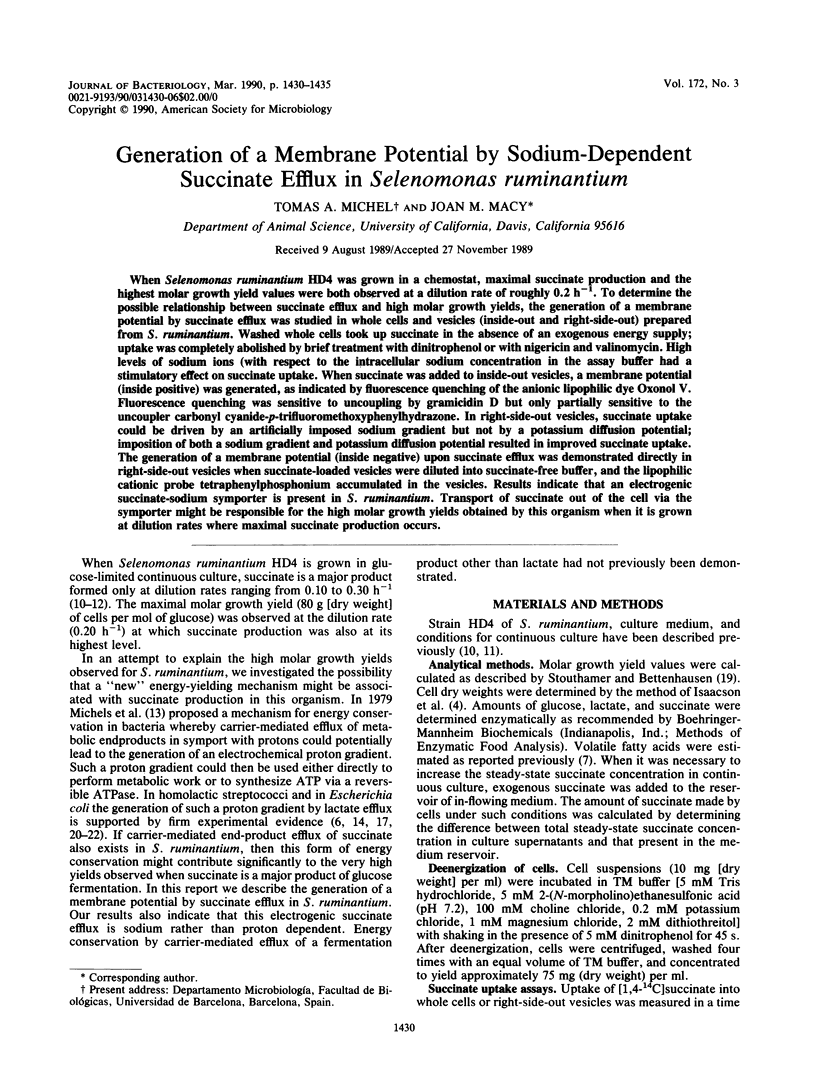
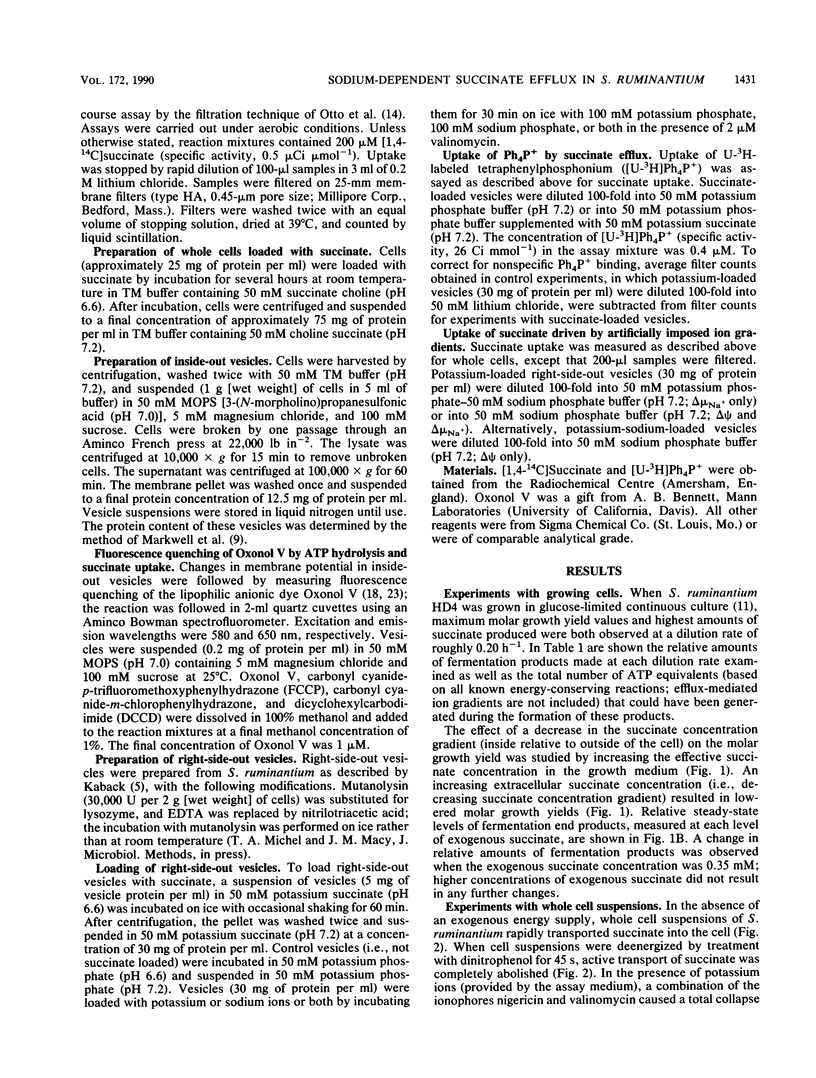
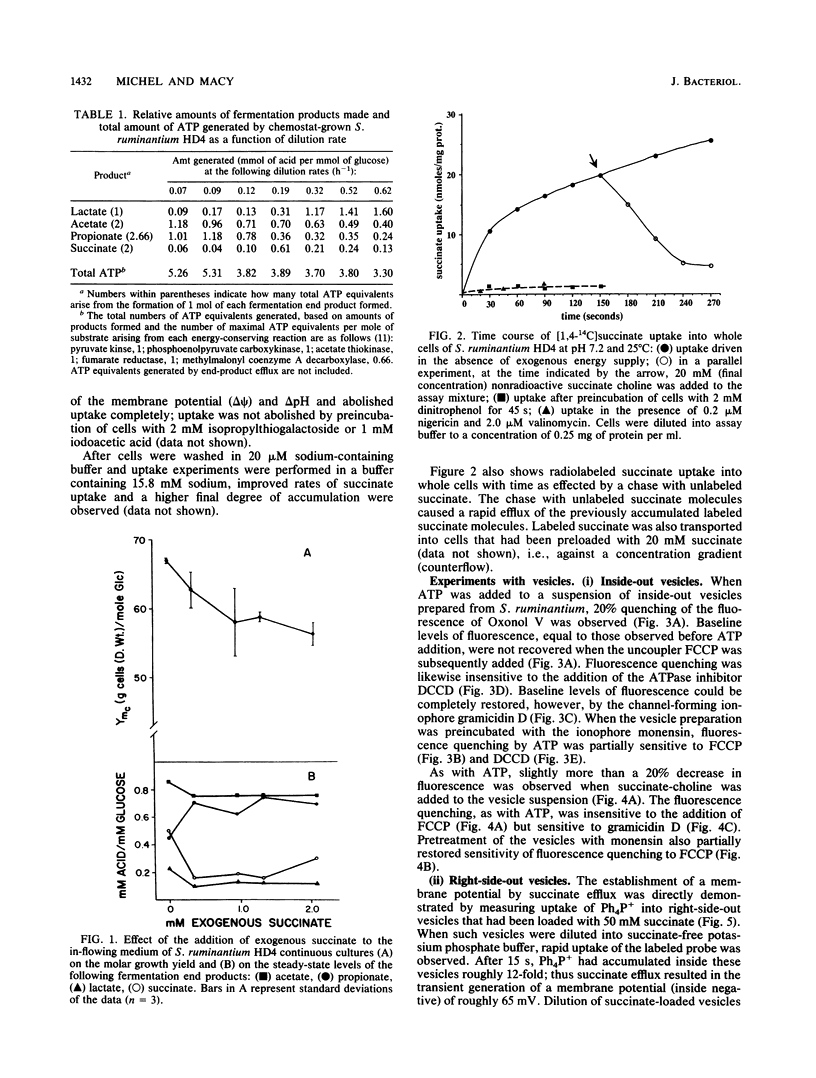
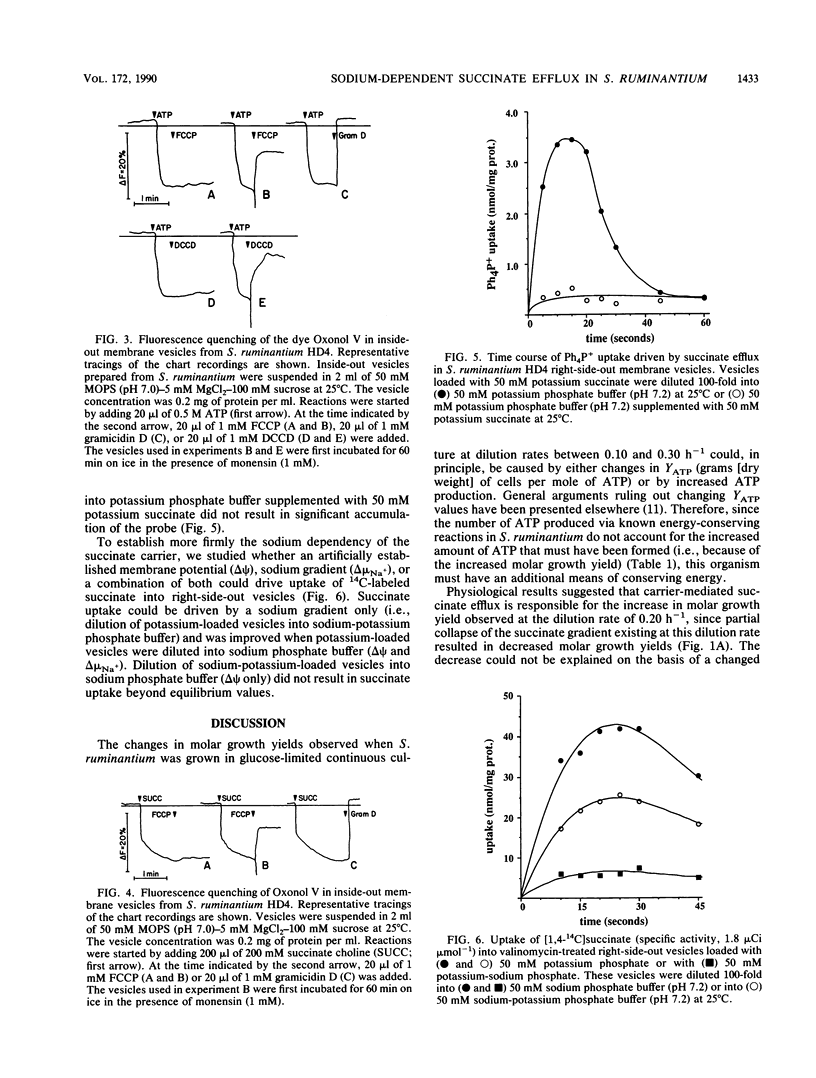
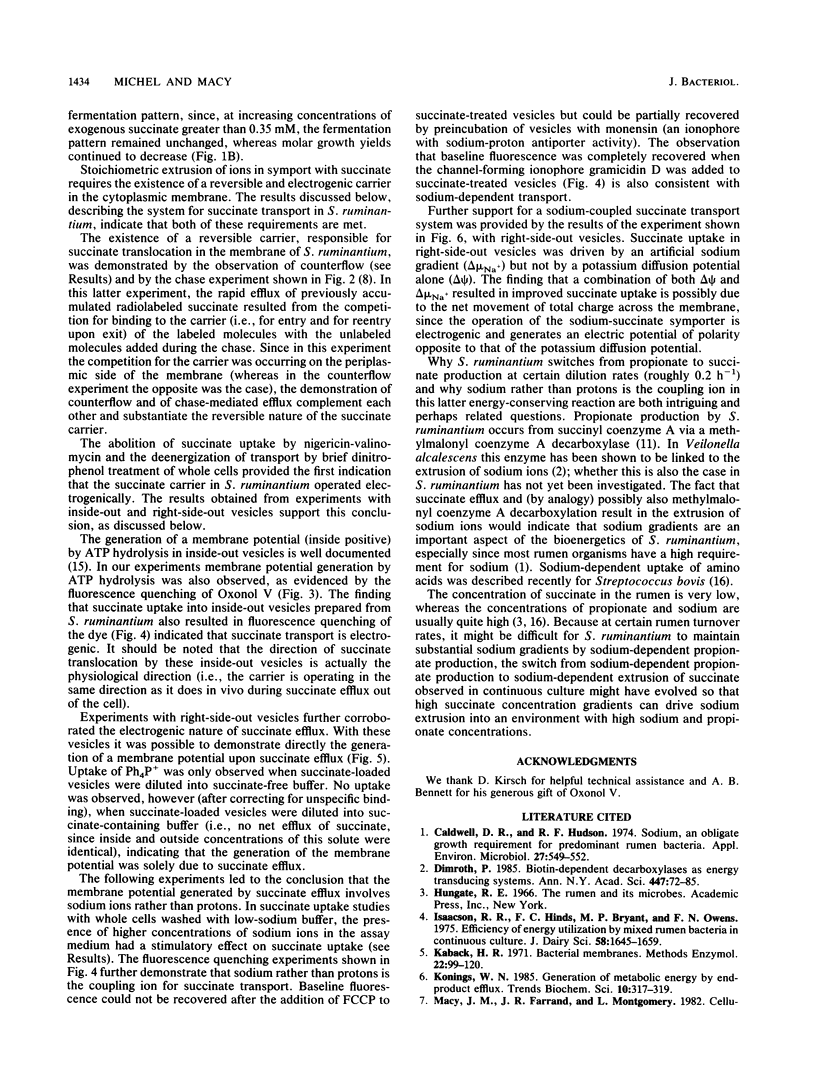
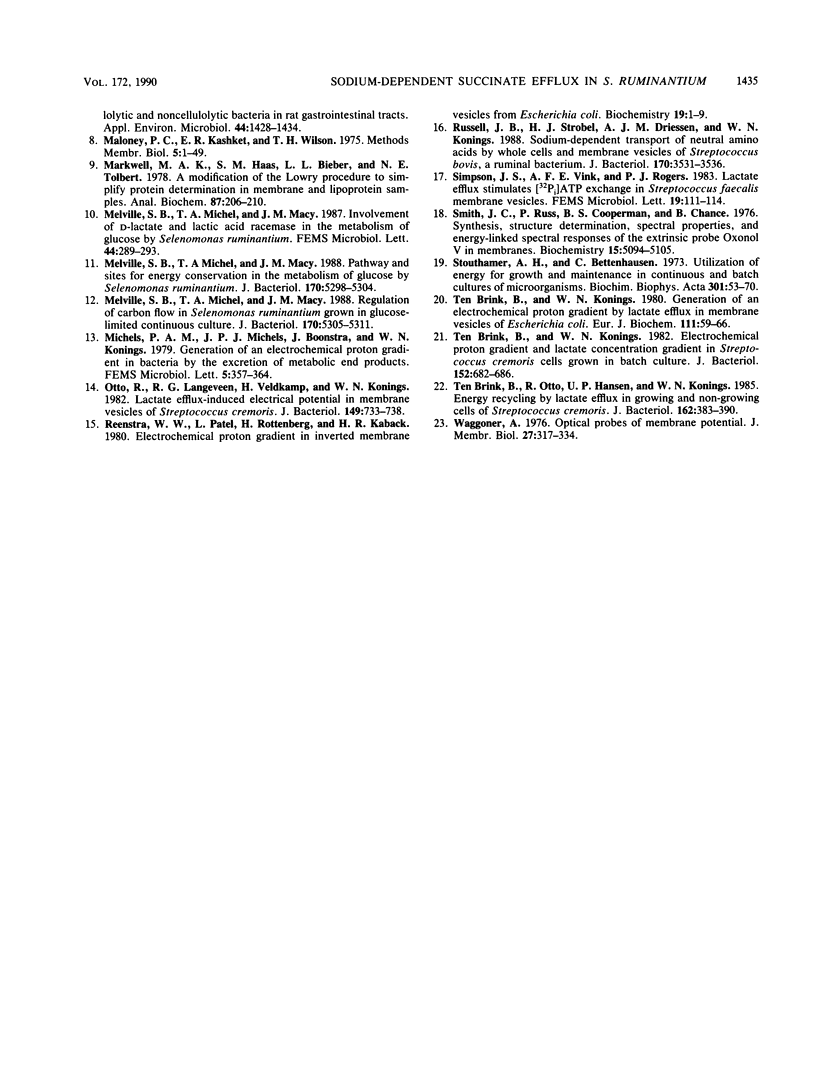
Selected References
These references are in PubMed. This may not be the complete list of references from this article.
- Caldwell D. R., Hudson R. F. Sodium, an obligate growth requirement for predominant rumen bacteria. Appl Microbiol. 1974 Mar;27(3):549–552. doi: 10.1128/am.27.3.549-552.1974. [DOI] [PMC free article] [PubMed] [Google Scholar]
- Dimroth P. Biotin-dependent decarboxylases as energy transducing systems. Ann N Y Acad Sci. 1985;447:72–85. doi: 10.1111/j.1749-6632.1985.tb18426.x. [DOI] [PubMed] [Google Scholar]
- Isaacson H. R., Hinds F. C., Bryant M. P., Owens F. N. Efficiency of energy utilization by mixed rumen bacteria in continuous culture. J Dairy Sci. 1975 Nov;58(11):1645–1659. doi: 10.3168/jds.S0022-0302(75)84763-1. [DOI] [PubMed] [Google Scholar]
- Markwell M. A., Haas S. M., Bieber L. L., Tolbert N. E. A modification of the Lowry procedure to simplify protein determination in membrane and lipoprotein samples. Anal Biochem. 1978 Jun 15;87(1):206–210. doi: 10.1016/0003-2697(78)90586-9. [DOI] [PubMed] [Google Scholar]
- Melville S. B., Michel T. A., Macy J. M. Pathway and sites for energy conservation in the metabolism of glucose by Selenomonas ruminantium. J Bacteriol. 1988 Nov;170(11):5298–5304. doi: 10.1128/jb.170.11.5298-5304.1988. [DOI] [PMC free article] [PubMed] [Google Scholar]
- Melville S. B., Michel T. A., Macy J. M. Regulation of carbon flow in Selenomonas ruminantium grown in glucose-limited continuous culture. J Bacteriol. 1988 Nov;170(11):5305–5311. doi: 10.1128/jb.170.11.5305-5311.1988. [DOI] [PMC free article] [PubMed] [Google Scholar]
- Otto R., Lageveen R. G., Veldkamp H., Konings W. N. Lactate efflux-induced electrical potential in membrane vesicles of Streptococcus cremoris. J Bacteriol. 1982 Feb;149(2):733–738. doi: 10.1128/jb.149.2.733-738.1982. [DOI] [PMC free article] [PubMed] [Google Scholar]
- Reenstra W. W., Patel L., Rottenberg H., Kaback H. R. Electrochemical proton gradient in inverted membrane vesicles from Escherichia coli. Biochemistry. 1980 Jan 8;19(1):1–9. doi: 10.1021/bi00542a001. [DOI] [PubMed] [Google Scholar]
- Russell J. B., Strobel H. J., Driessen A. J., Konings W. N. Sodium-dependent transport of neutral amino acids by whole cells and membrane vesicles of Streptococcus bovis, a ruminal bacterium. J Bacteriol. 1988 Aug;170(8):3531–3536. doi: 10.1128/jb.170.8.3531-3536.1988. [DOI] [PMC free article] [PubMed] [Google Scholar]
- Smith J. C., Russ P., Cooperman B. S., Chance B. Synthesis, structure determination, spectral properties, and energy-linked spectral responses of the extrinsic probe oxonol V in membranes. Biochemistry. 1976 Nov 16;15(23):5094–5105. doi: 10.1021/bi00668a023. [DOI] [PubMed] [Google Scholar]
- Stouthamer A. H., Bettenhaussen C. Utilization of energy for growth and maintenance in continuous and batch cultures of microorganisms. A reevaluation of the method for the determination of ATP production by measuring molar growth yields. Biochim Biophys Acta. 1973 Feb 12;301(1):53–70. doi: 10.1016/0304-4173(73)90012-8. [DOI] [PubMed] [Google Scholar]
- Ten Brink B., Konings W. N. Generation of an electrochemical proton gradient by lactate efflux in membrane vesicles of Escherichia coli. Eur J Biochem. 1980 Oct;111(1):59–66. doi: 10.1111/j.1432-1033.1980.tb06074.x. [DOI] [PubMed] [Google Scholar]
- Waggoner A. Optical probes of membrane potential. J Membr Biol. 1976 Jun 30;27(4):317–334. doi: 10.1007/BF01869143. [DOI] [PubMed] [Google Scholar]
- ten Brink B., Konings W. N. Electrochemical proton gradient and lactate concentration gradient in Streptococcus cremoris cells grown in batch culture. J Bacteriol. 1982 Nov;152(2):682–686. doi: 10.1128/jb.152.2.682-686.1982. [DOI] [PMC free article] [PubMed] [Google Scholar]
- ten Brink B., Otto R., Hansen U. P., Konings W. N. Energy recycling by lactate efflux in growing and nongrowing cells of Streptococcus cremoris. J Bacteriol. 1985 Apr;162(1):383–390. doi: 10.1128/jb.162.1.383-390.1985. [DOI] [PMC free article] [PubMed] [Google Scholar]