Abstract
In a cell culture of Saccharomyces cerevisiae exponentially growing in basal medium, only 0.02% of the cells were osmotolerant, i.e., survived transfer to medium containing 1.4 M NaCl. Short-time conditioning in 0.7 M NaCl medium transformed the whole population into an osmotolerance phenotype. During this conditioning, the rate of formation of glycerol, the main compatible solute in S. cerevisiae, increased threefold and the specific activity of glycerol-3-phosphate dehydrogenase (NAD+) (GPDH) (EC 1.1.1.8) was enhanced sixfold. The apparent flux control coefficient for GPDH in the formation of glycerol was estimated to be 0.6. Glycerol production was also favored by regulated activities of alcohol dehydrogenase (EC 1.1.1.1) and aldehyde dehydrogenase [NAD(P)]+ (EC 1.2.1.5). About 50% of the total glycerol produced during conditioning in 0.7 M NaCl was retained intracellularly, and the increased glycerol accumulation was shown to be not merely a result of enhanced production rate but also of increased retention of glycerol. Washing the cells with solutions of lower salinities resulted in loss of glycerol, with retained levels proportional to the concentration of NaCl in the washing solution. Cycloheximide addition inhibited the development of acquired osmotolerance and conditioned cells washed free of glycerol retained a high degree of osmotolerance, which indicate that protein synthesis was required to establish the osmotolerance state.
Full text
PDF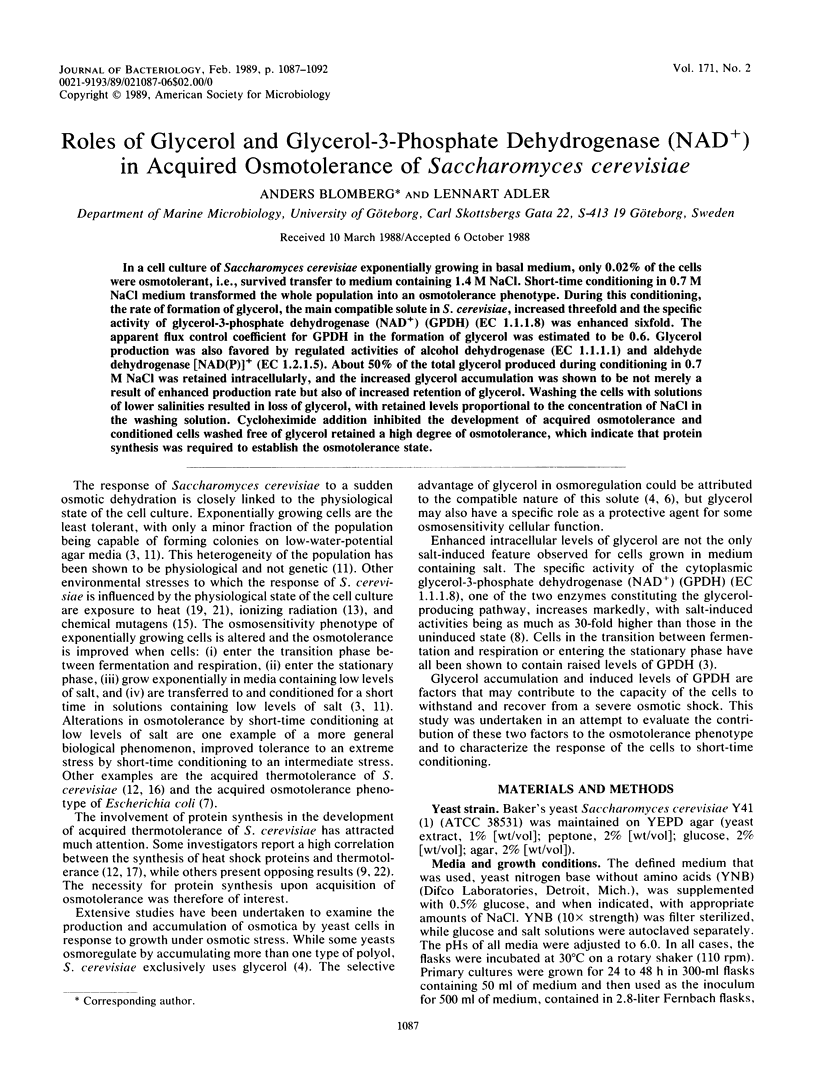
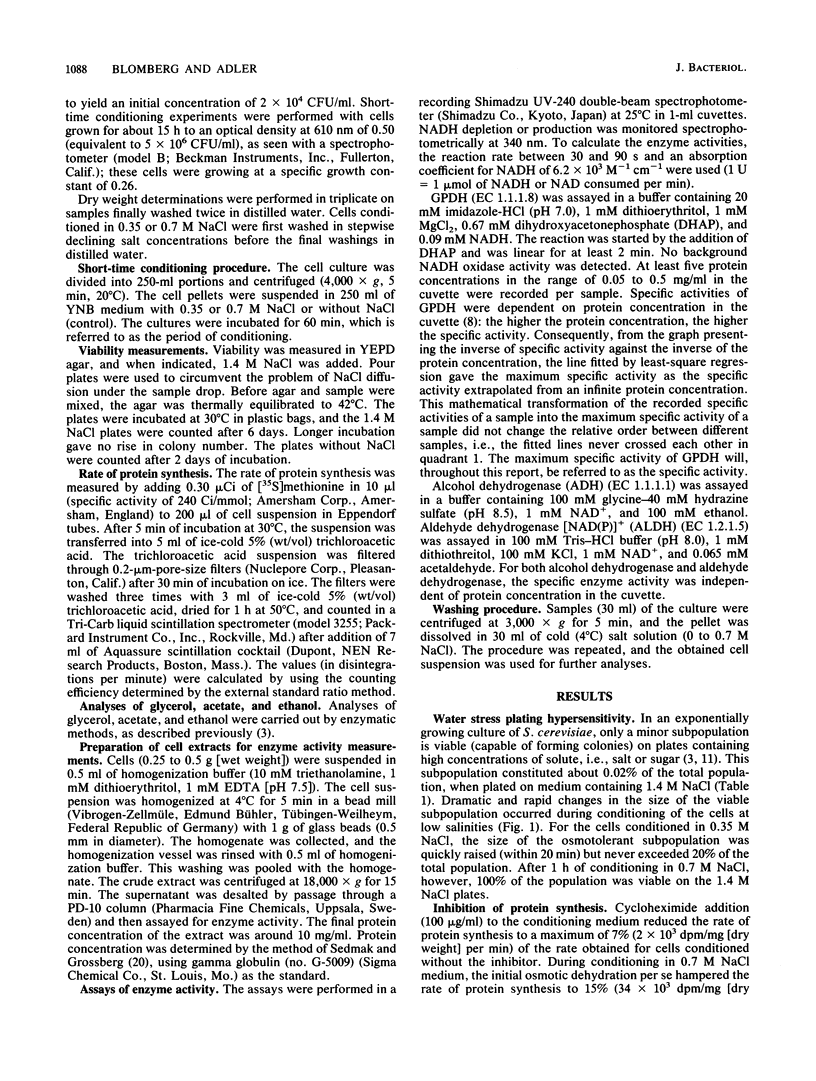
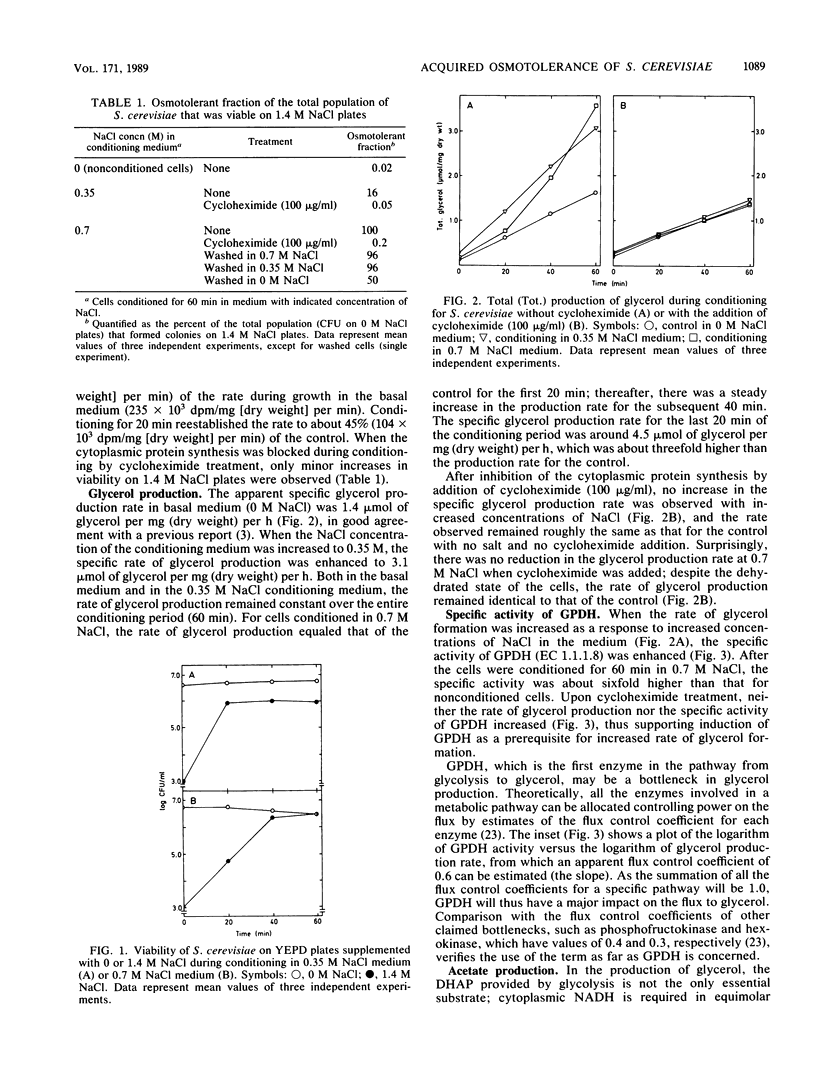
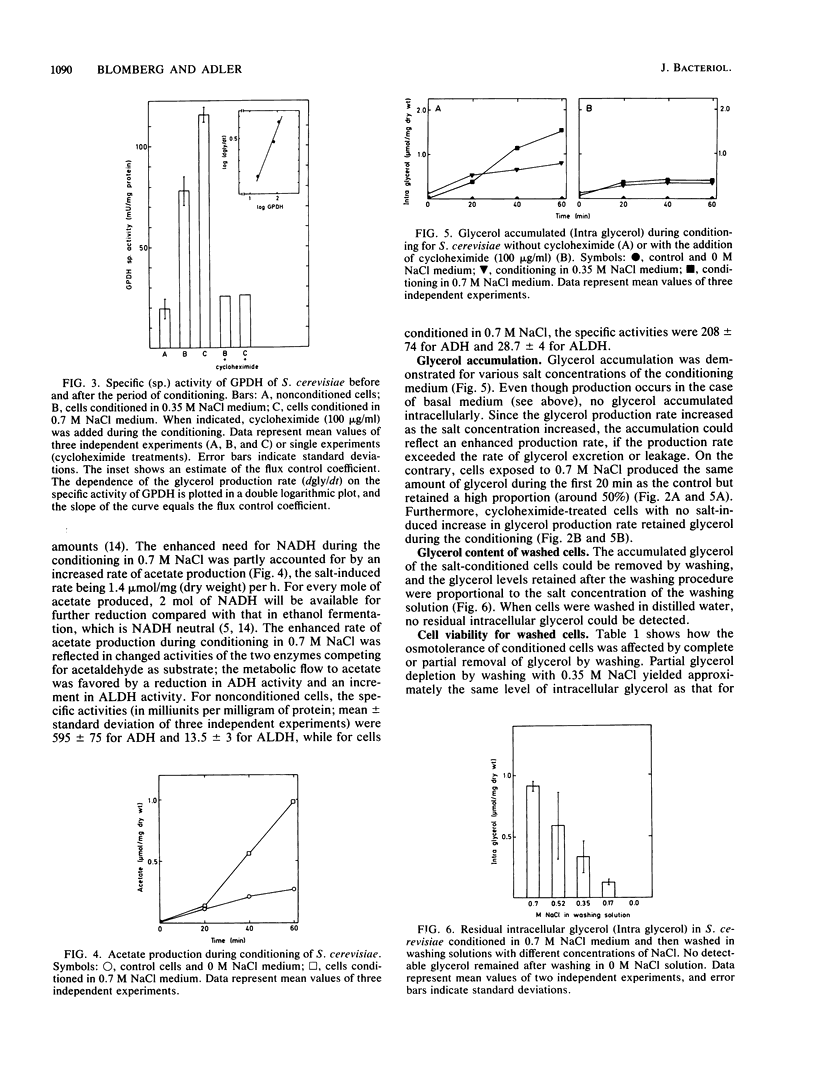
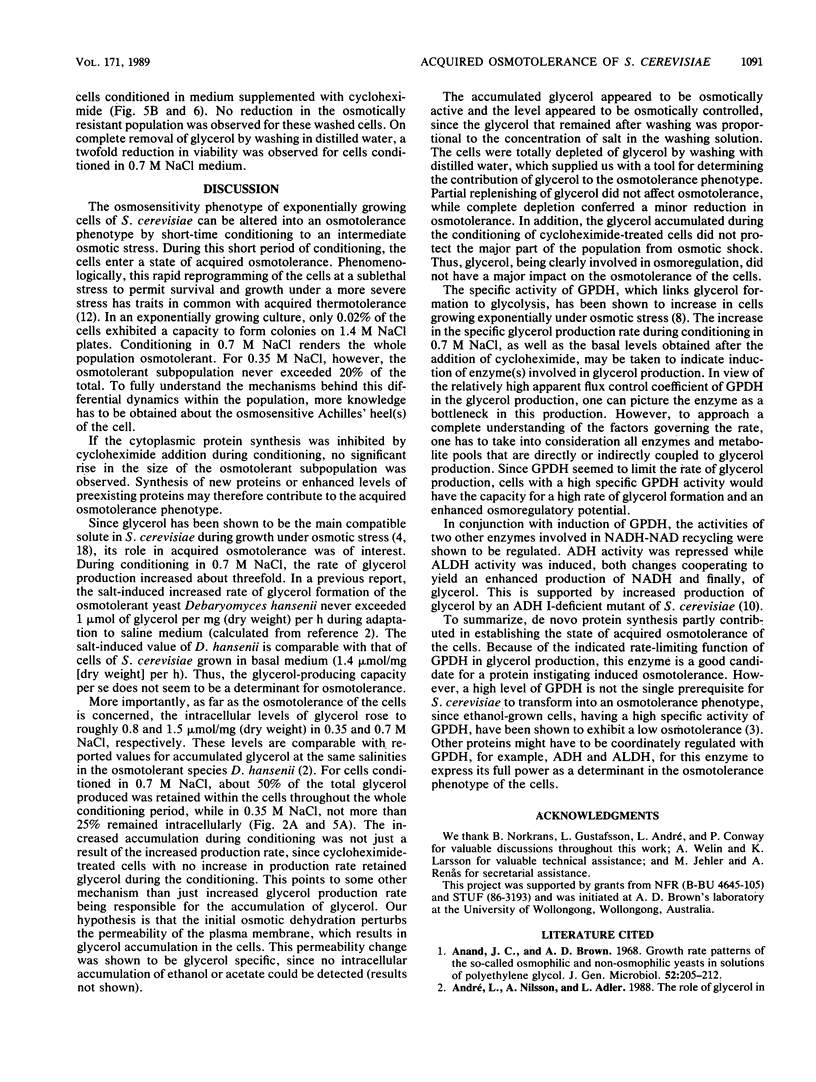
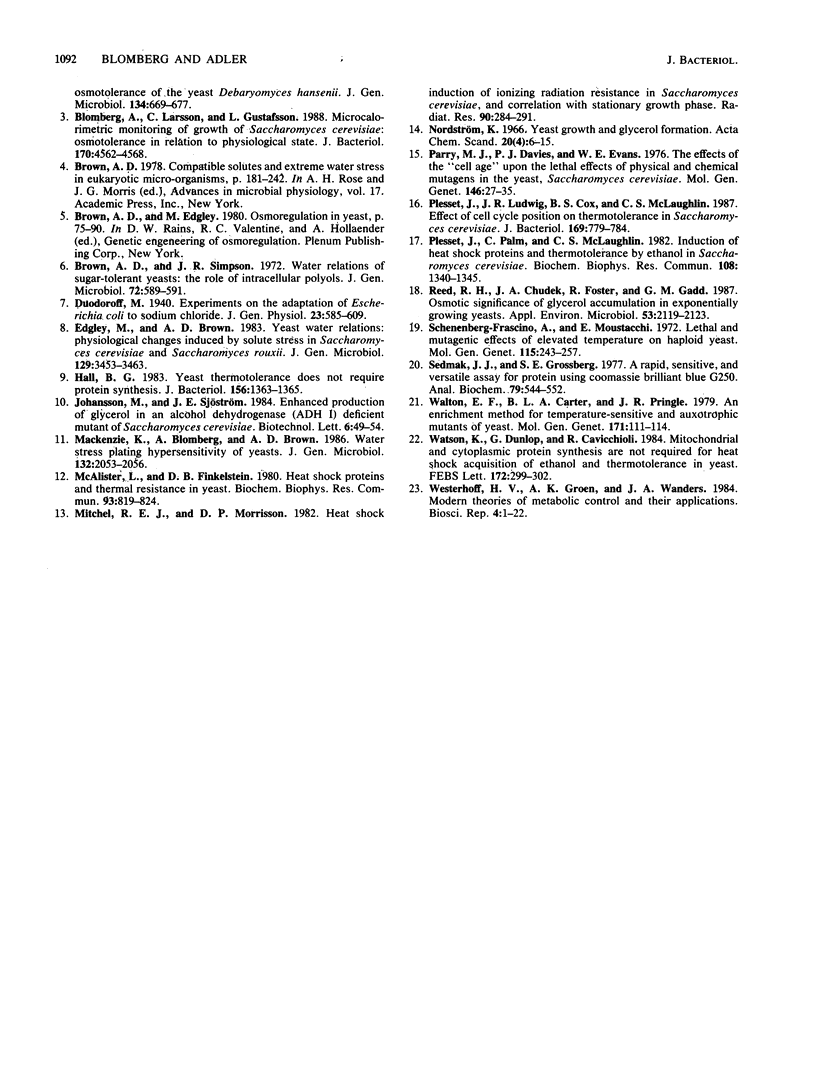
Selected References
These references are in PubMed. This may not be the complete list of references from this article.
- Blomberg A., Larsson C., Gustafsson L. Microcalorimetric monitoring of growth of Saccharomyces cerevisiae: osmotolerance in relation to physiological state. J Bacteriol. 1988 Oct;170(10):4562–4568. doi: 10.1128/jb.170.10.4562-4568.1988. [DOI] [PMC free article] [PubMed] [Google Scholar]
- Brown A. D., Simpson J. R. Water relations of sugar-tolerant yeasts: the role of intracellular polyols. J Gen Microbiol. 1972 Oct;72(3):589–591. doi: 10.1099/00221287-72-3-589. [DOI] [PubMed] [Google Scholar]
- Hall B. G. Yeast thermotolerance does not require protein synthesis. J Bacteriol. 1983 Dec;156(3):1363–1365. doi: 10.1128/jb.156.3.1363-1365.1983. [DOI] [PMC free article] [PubMed] [Google Scholar]
- Mackenzie K. F., Blomberg A., Brown A. D. Water stress plating hypersensitivity of yeasts. J Gen Microbiol. 1986 Jul;132(7):2053–2056. doi: 10.1099/00221287-132-7-2053. [DOI] [PubMed] [Google Scholar]
- McAlister L., Finkelstein D. B. Heat shock proteins and thermal resistance in yeast. Biochem Biophys Res Commun. 1980 Apr 14;93(3):819–824. doi: 10.1016/0006-291x(80)91150-x. [DOI] [PubMed] [Google Scholar]
- Mitchel R. E., Morrison D. P. Heat-shock induction of ionizing radiation resistance in Saccharomyces cerevisiae, and correlation with stationary growth phase. Radiat Res. 1982 May;90(2):284–291. [PubMed] [Google Scholar]
- Parry J. M., Davies P. J., Evans W. E. The effects of "cell age" upon the lethal effects of physical and chemical mutagens in the yeast, Saccharomyces cerevisiae. Mol Gen Genet. 1976 Jul 5;146(1):27–35. doi: 10.1007/BF00267979. [DOI] [PubMed] [Google Scholar]
- Plesset J., Ludwig J. R., Cox B. S., McLaughlin C. S. Effect of cell cycle position on thermotolerance in Saccharomyces cerevisiae. J Bacteriol. 1987 Feb;169(2):779–784. doi: 10.1128/jb.169.2.779-784.1987. [DOI] [PMC free article] [PubMed] [Google Scholar]
- Plesset J., Palm C., McLaughlin C. S. Induction of heat shock proteins and thermotolerance by ethanol in Saccharomyces cerevisiae. Biochem Biophys Res Commun. 1982 Oct 15;108(3):1340–1345. doi: 10.1016/0006-291x(82)92147-7. [DOI] [PubMed] [Google Scholar]
- Reed R. H., Chudek J. A., Foster R., Gadd G. M. Osmotic significance of glycerol accumulation in exponentially growing yeasts. Appl Environ Microbiol. 1987 Sep;53(9):2119–2123. doi: 10.1128/aem.53.9.2119-2123.1987. [DOI] [PMC free article] [PubMed] [Google Scholar]
- Schenberg-Frascino A., Moustacchi E. Lethal and mutagenic effects of elevated temperature on haploid yeast. I. Variations in sensitivity during the cell cycle. Mol Gen Genet. 1972;115(3):243–257. doi: 10.1007/BF00268888. [DOI] [PubMed] [Google Scholar]
- Sedmak J. J., Grossberg S. E. A rapid, sensitive, and versatile assay for protein using Coomassie brilliant blue G250. Anal Biochem. 1977 May 1;79(1-2):544–552. doi: 10.1016/0003-2697(77)90428-6. [DOI] [PubMed] [Google Scholar]
- Watson K., Dunlop G., Cavicchioli R. Mitochondrial and cytoplasmic protein syntheses are not required for heat shock acquisition of ethanol and thermotolerance in yeast. FEBS Lett. 1984 Jul 9;172(2):299–302. doi: 10.1016/0014-5793(84)81145-x. [DOI] [PubMed] [Google Scholar]
- Westerhoff H. V., Groen A. K., Wanders R. J. Modern theories of metabolic control and their applications (review). Biosci Rep. 1984 Jan;4(1):1–22. doi: 10.1007/BF01120819. [DOI] [PubMed] [Google Scholar]