Abstract
The tricarboxylate transport operon (tctI) was cloned in Escherichia coli as a 12-kilobase (kb) fragment from an EcoRI library of the Salmonella typhimurium chromosome in lambda gtWES. It was further subcloned as a 12-kb fragment into pACYC184 and as an 8-kb fragment into pBR322. By insertional mutagenesis mediated by lambda Tn5, restriction mapping, and phenotypic testing, the tctI operon was localized to a 4.5-kb region. The tctC gene which encodes a periplasmic binding protein (C protein) was located near the center of the insert. E. coli/tctI clones on either multicopy or single-copy vectors grew on the same tricarboxylates as S. typhimurium, although unusually long growth lags were observed. E. coli/tctI clones exhibited similar [14C]fluorocitrate transport kinetics to those of S. typhimurium, whereas E. coli alone was virtually impermeable to [14C]fluorocitrate. The periplasmic C proteins (C1 and C2 isoelectric forms) were produced in prodigious quantities from the cloned strains. Motile E. coli/tctI clones were not chemotactic toward citrate, whereas tctI deletion mutants of S. typhimurium were. Taken together, these observations indicate that tctI is not an operon involved in chemotaxis.
Full text
PDF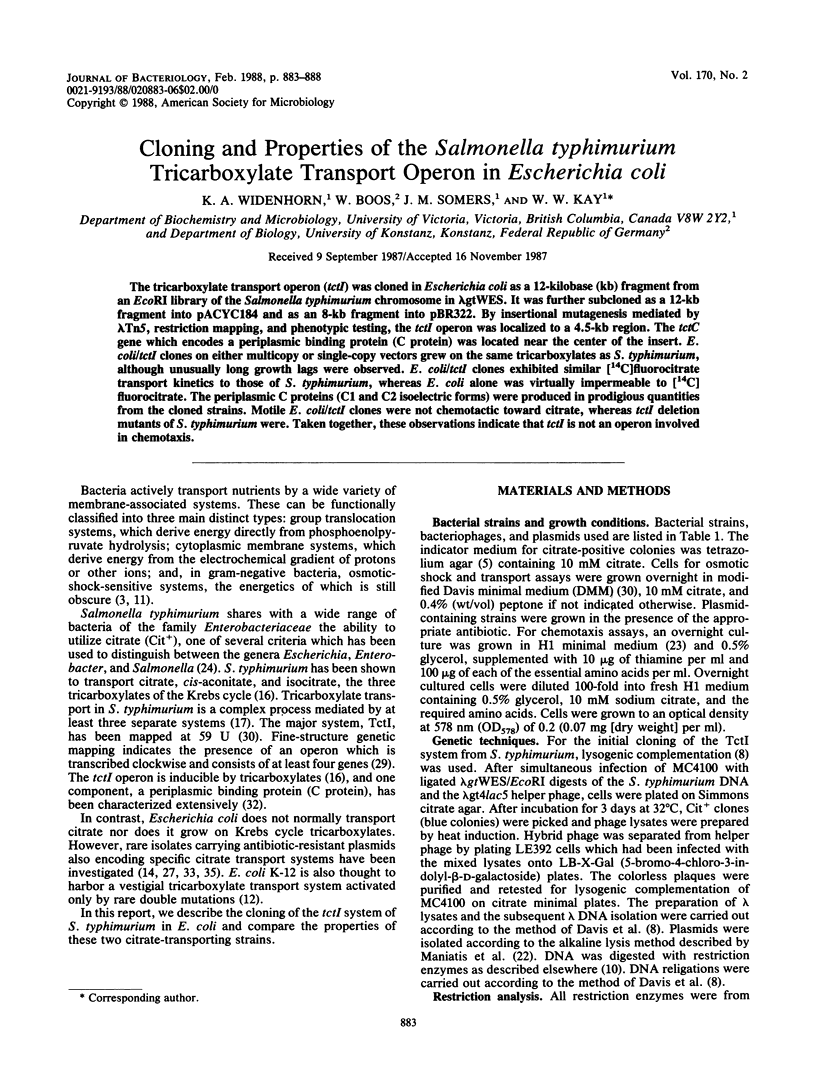
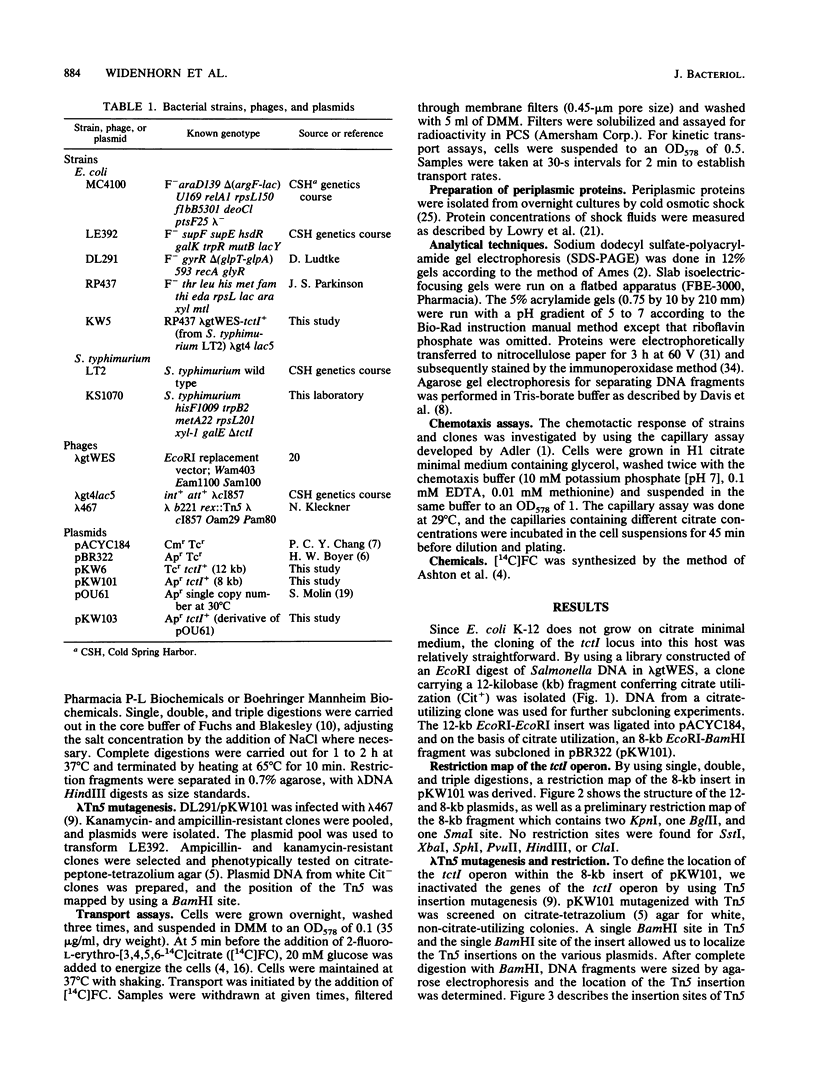
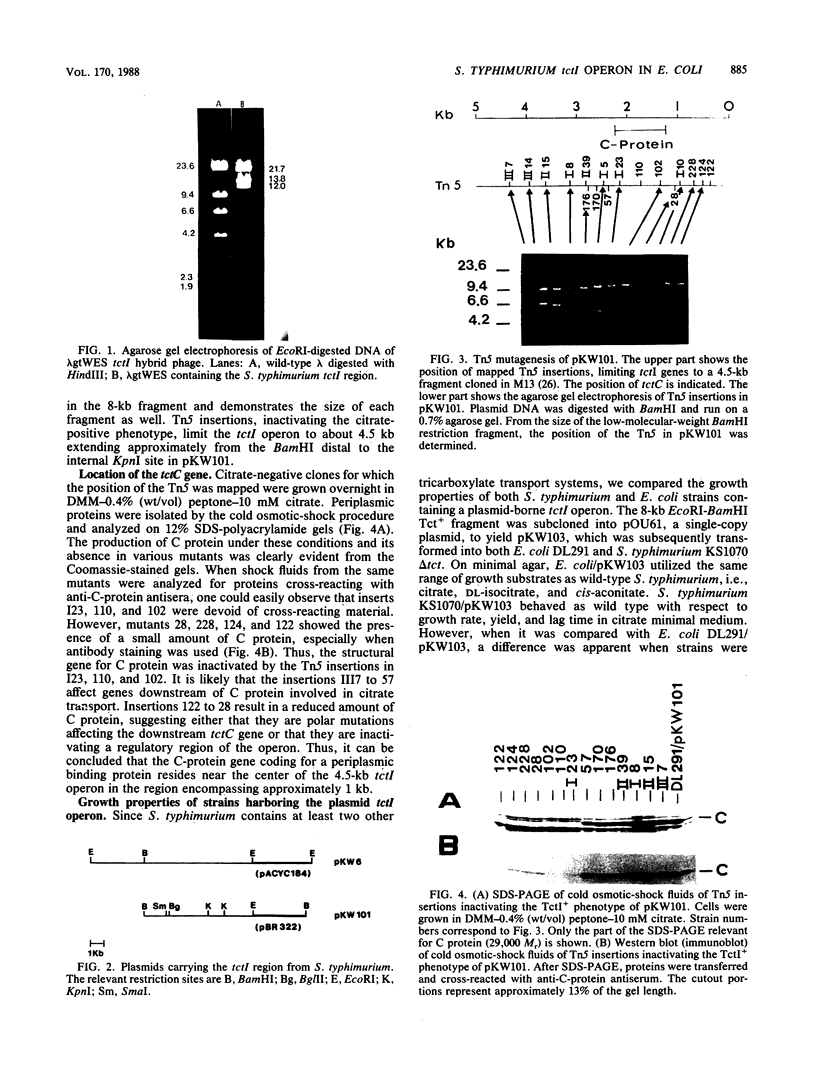
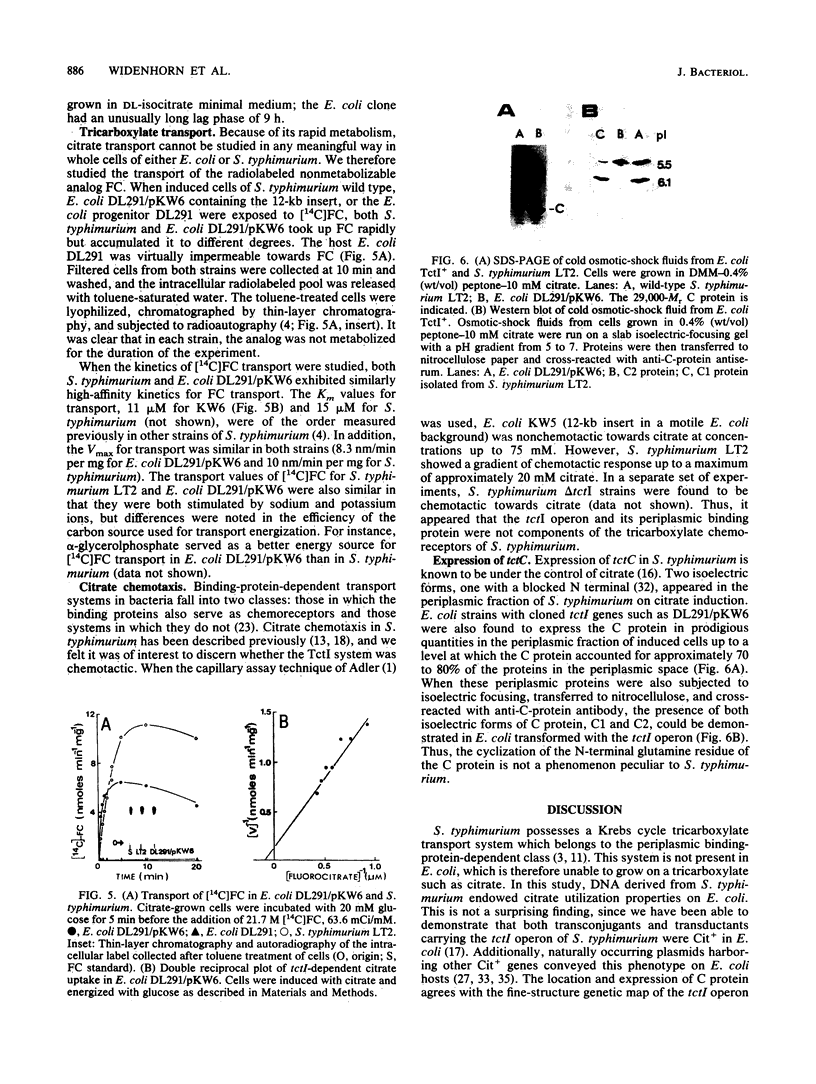
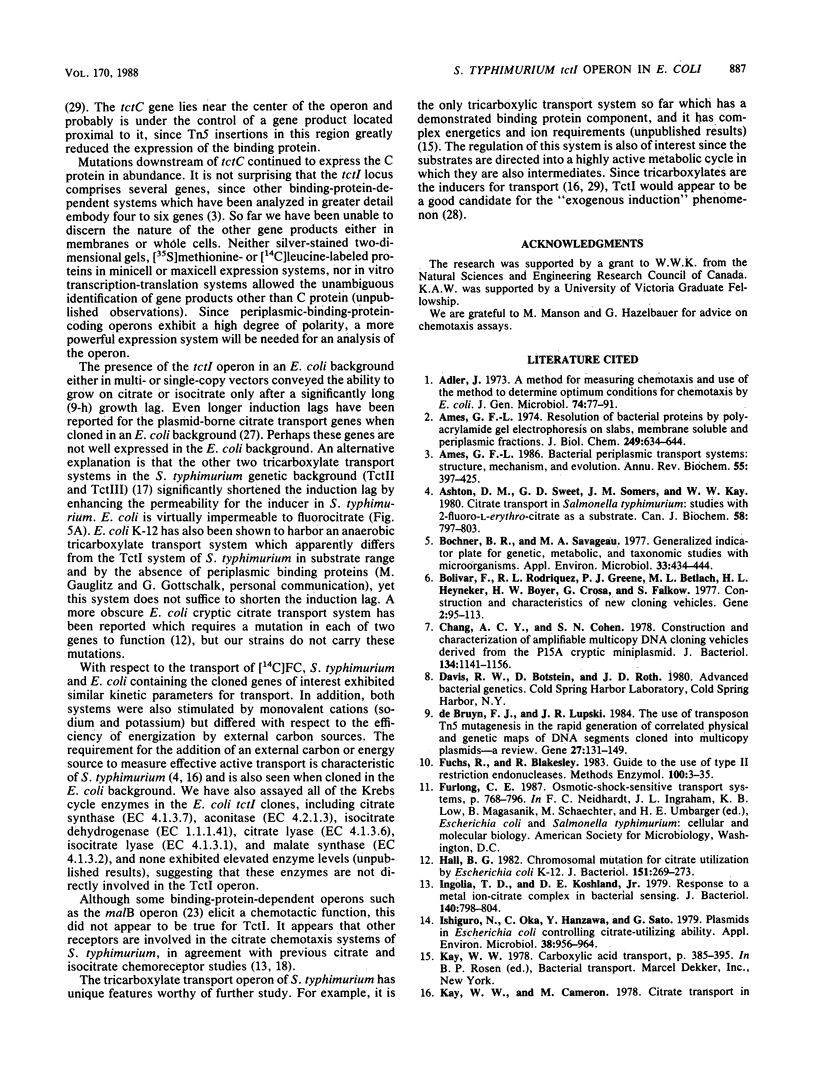
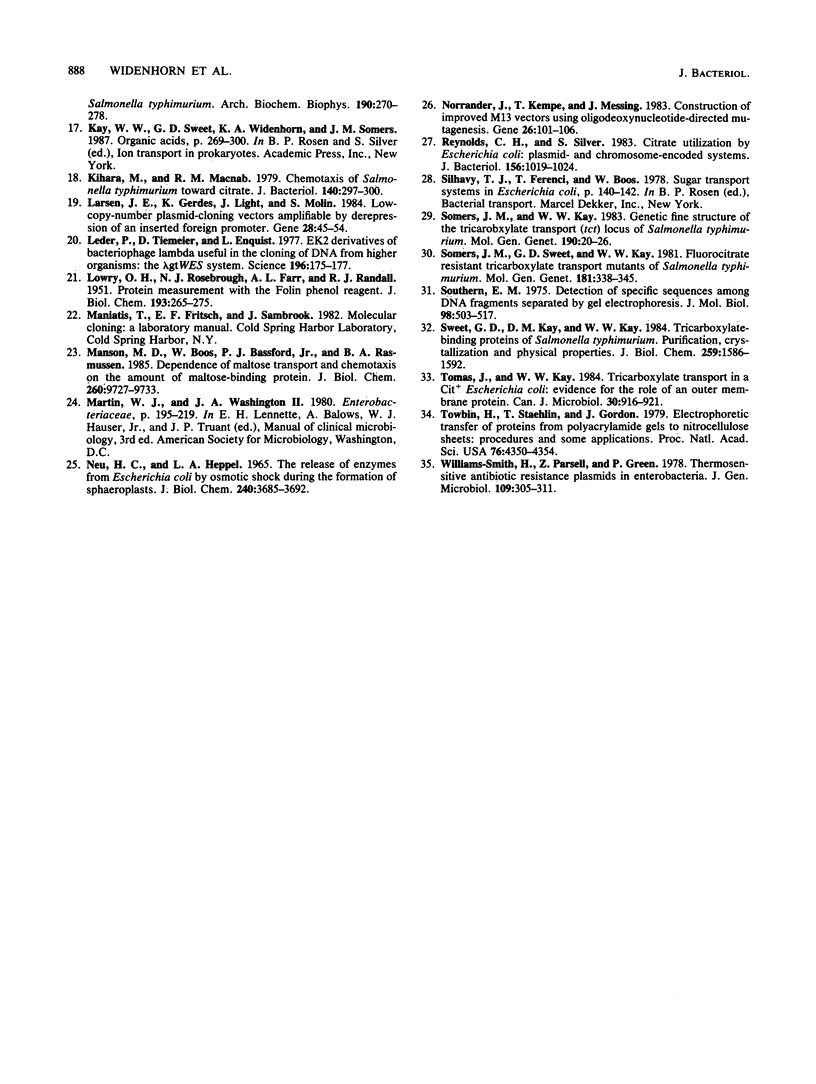
Images in this article
Selected References
These references are in PubMed. This may not be the complete list of references from this article.
- Adler J. A method for measuring chemotaxis and use of the method to determine optimum conditions for chemotaxis by Escherichia coli. J Gen Microbiol. 1973 Jan;74(1):77–91. doi: 10.1099/00221287-74-1-77. [DOI] [PubMed] [Google Scholar]
- Ames G. F. Bacterial periplasmic transport systems: structure, mechanism, and evolution. Annu Rev Biochem. 1986;55:397–425. doi: 10.1146/annurev.bi.55.070186.002145. [DOI] [PubMed] [Google Scholar]
- Ames G. F. Resolution of bacterial proteins by polyacrylamide gel electrophoresis on slabs. Membrane, soluble, and periplasmic fractions. J Biol Chem. 1974 Jan 25;249(2):634–644. [PubMed] [Google Scholar]
- Ashton D. M., Sweet G. D., Somers J. M., Kay W. W. Citrate transport in Salmonella typhimurium: studies with 2-fluoro-L-erythro-citrate as a substrate. Can J Biochem. 1980 Oct;58(10):797–803. doi: 10.1139/o80-111. [DOI] [PubMed] [Google Scholar]
- Bochner B. R., Savageau M. A. Generalized indicator plate for genetic, metabolic, and taxonomic studies with microorganisms. Appl Environ Microbiol. 1977 Feb;33(2):434–444. doi: 10.1128/aem.33.2.434-444.1977. [DOI] [PMC free article] [PubMed] [Google Scholar]
- Bolivar F., Rodriguez R. L., Greene P. J., Betlach M. C., Heyneker H. L., Boyer H. W., Crosa J. H., Falkow S. Construction and characterization of new cloning vehicles. II. A multipurpose cloning system. Gene. 1977;2(2):95–113. [PubMed] [Google Scholar]
- Chang A. C., Cohen S. N. Construction and characterization of amplifiable multicopy DNA cloning vehicles derived from the P15A cryptic miniplasmid. J Bacteriol. 1978 Jun;134(3):1141–1156. doi: 10.1128/jb.134.3.1141-1156.1978. [DOI] [PMC free article] [PubMed] [Google Scholar]
- Hall B. G. Chromosomal mutation for citrate utilization by Escherichia coli K-12. J Bacteriol. 1982 Jul;151(1):269–273. doi: 10.1128/jb.151.1.269-273.1982. [DOI] [PMC free article] [PubMed] [Google Scholar]
- Ingolia T. D., Koshland D. E., Jr Response to a metal ion-citrate complex in bacterial sensing. J Bacteriol. 1979 Dec;140(3):798–804. doi: 10.1128/jb.140.3.798-804.1979. [DOI] [PMC free article] [PubMed] [Google Scholar]
- Ishiguro N., Oka C., Hanzawa Y., Sato G. Plasmids in Escherichia coli controlling citrate-utilizing ability. Appl Environ Microbiol. 1979 Nov;38(5):956–964. doi: 10.1128/aem.38.5.956-964.1979. [DOI] [PMC free article] [PubMed] [Google Scholar]
- Kihara M., Macnab R. M. Chemotaxis of Salmonella typhimurium toward citrate. J Bacteriol. 1979 Oct;140(1):297–300. doi: 10.1128/jb.140.1.297-300.1979. [DOI] [PMC free article] [PubMed] [Google Scholar]
- LOWRY O. H., ROSEBROUGH N. J., FARR A. L., RANDALL R. J. Protein measurement with the Folin phenol reagent. J Biol Chem. 1951 Nov;193(1):265–275. [PubMed] [Google Scholar]
- Larsen J. E., Gerdes K., Light J., Molin S. Low-copy-number plasmid-cloning vectors amplifiable by derepression of an inserted foreign promoter. Gene. 1984 Apr;28(1):45–54. doi: 10.1016/0378-1119(84)90086-6. [DOI] [PubMed] [Google Scholar]
- Leder P., Tiemeier D., Enquist L. EK2 derivatives of bacteriophage lambda useful in the cloning of DNA from higher organisms: the lambdagtWES system. Science. 1977 Apr 8;196(4286):175–177. doi: 10.1126/science.322278. [DOI] [PubMed] [Google Scholar]
- Manson M. D., Boos W., Bassford P. J., Jr, Rasmussen B. A. Dependence of maltose transport and chemotaxis on the amount of maltose-binding protein. J Biol Chem. 1985 Aug 15;260(17):9727–9733. [PubMed] [Google Scholar]
- Neu H. C., Heppel L. A. The release of enzymes from Escherichia coli by osmotic shock and during the formation of spheroplasts. J Biol Chem. 1965 Sep;240(9):3685–3692. [PubMed] [Google Scholar]
- Norrander J., Kempe T., Messing J. Construction of improved M13 vectors using oligodeoxynucleotide-directed mutagenesis. Gene. 1983 Dec;26(1):101–106. doi: 10.1016/0378-1119(83)90040-9. [DOI] [PubMed] [Google Scholar]
- Reynolds C. H., Silver S. Citrate utilization by Escherichia coli: plasmid- and chromosome-encoded systems. J Bacteriol. 1983 Dec;156(3):1019–1024. doi: 10.1128/jb.156.3.1019-1024.1983. [DOI] [PMC free article] [PubMed] [Google Scholar]
- Smith H. W., Parsell Z., Green P. Thermosensitive H1 plasmids determining citrate utilization. J Gen Microbiol. 1978 Dec;109(2):305–311. doi: 10.1099/00221287-109-2-305. [DOI] [PubMed] [Google Scholar]
- Somers J. M., Kay W. W. Genetic fine structure of the tricarboxylate transport (tct) locus of Salmonella typhimurium. Mol Gen Genet. 1983;190(1):20–26. doi: 10.1007/BF00330319. [DOI] [PubMed] [Google Scholar]
- Somers J. M., Sweet G. D., Kay W. W. Flurorcitrate resistant tricarboxylate transport mutants of Salmonella typhimurium. Mol Gen Genet. 1981;181(3):338–345. doi: 10.1007/BF00425608. [DOI] [PubMed] [Google Scholar]
- Southern E. M. Detection of specific sequences among DNA fragments separated by gel electrophoresis. J Mol Biol. 1975 Nov 5;98(3):503–517. doi: 10.1016/s0022-2836(75)80083-0. [DOI] [PubMed] [Google Scholar]
- Sweet G. D., Kay C. M., Kay W. W. Tricarboxylate-binding proteins of Salmonella typhimurium. Purification, crystallization, and physical properties. J Biol Chem. 1984 Feb 10;259(3):1586–1592. [PubMed] [Google Scholar]
- Tomás J. M., Kay W. W. Tricarboxylate transport in a Cit+ Escherichia coli: evidence for the role of an outer membrane protein. Can J Microbiol. 1984 Jul;30(7):916–921. doi: 10.1139/m84-143. [DOI] [PubMed] [Google Scholar]
- Towbin H., Staehelin T., Gordon J. Electrophoretic transfer of proteins from polyacrylamide gels to nitrocellulose sheets: procedure and some applications. Proc Natl Acad Sci U S A. 1979 Sep;76(9):4350–4354. doi: 10.1073/pnas.76.9.4350. [DOI] [PMC free article] [PubMed] [Google Scholar]
- de Bruijn F. J., Lupski J. R. The use of transposon Tn5 mutagenesis in the rapid generation of correlated physical and genetic maps of DNA segments cloned into multicopy plasmids--a review. Gene. 1984 Feb;27(2):131–149. doi: 10.1016/0378-1119(84)90135-5. [DOI] [PubMed] [Google Scholar]