Abstract
The properties of enzymes involved in energy transduction from a mesophilic (Bacillus subtilis) and a thermophilic (B. stearothermophilus) bacterium were compared. Membrane preparations of the two organisms contained dehydrogenases for NADH, succinate, L-alpha-glycerophosphate, and L-lactate. Maximum NADH and cytochrome c oxidation rates were obtained at the respective growth temperatures of the two bacteria. The enzymes involved in the oxidation reactions in membranes of the thermophilic species were more thermostable than those of the mesophilic species. The apparent microviscosities of the two membrane preparations were studied at different temperatures. At the respective optimal growth temperatures, the apparent microviscosities of the membranes of the two organisms were remarkably similar. The transition from the gel to the liquid-crystalline state occurred at different temperatures in the two species. In the two species, the oxidation of physiological (NADH) and nonphysiological (N,N,N',N'-tetramethyl-p-phenylenediamine or phenazine methosulfate) electron donors led to generation of a proton motive force which varied strongly with temperature. At increasing temperatures, the efficiency of energy transduction declined because of increasing H+ permeability. At the growth temperature, the efficiency of energy transduction was lower in B. stearothermophilus than in the mesophilic species. Extremely high respiratory activities enabled B. stearothermophilus to maintain a high proton motive force at elevated temperatures. The pH dependence of proton motive force generation appeared to be similar in the two membrane preparations. The highest proton motive forces were generated at low external pH, mainly because of a high pH gradient. At increasing external pH, the proton motive force declined.
Full text
PDF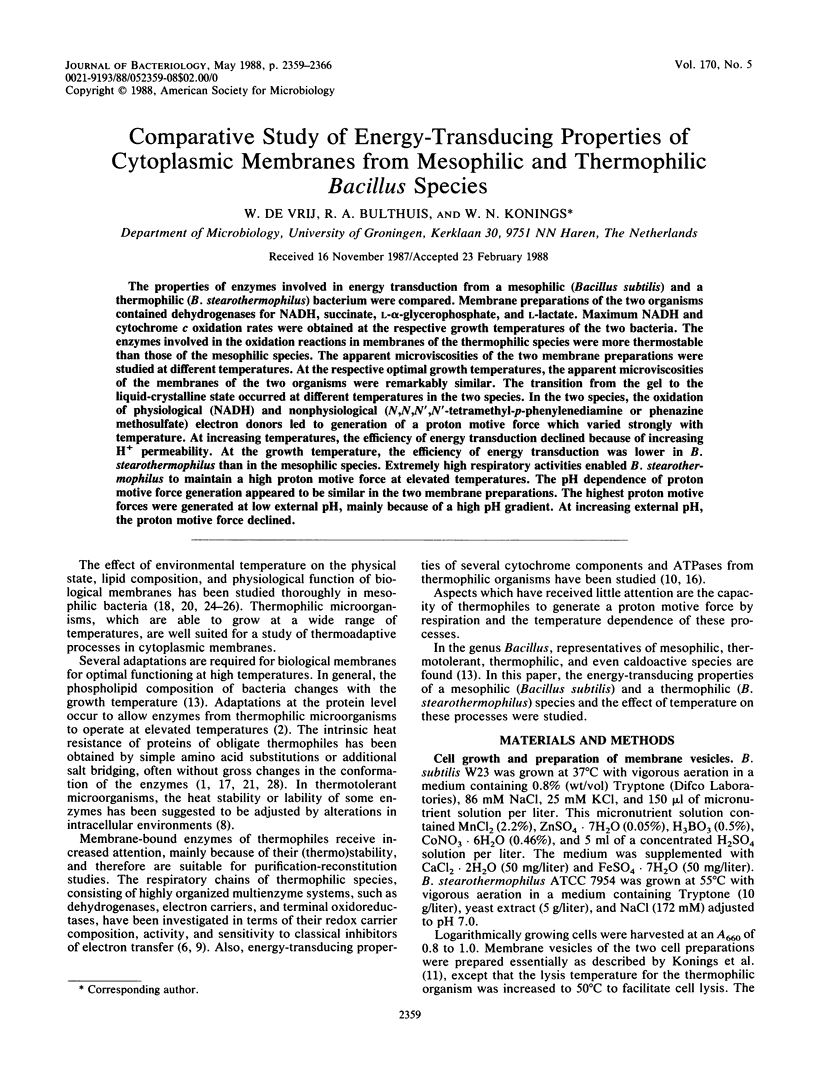
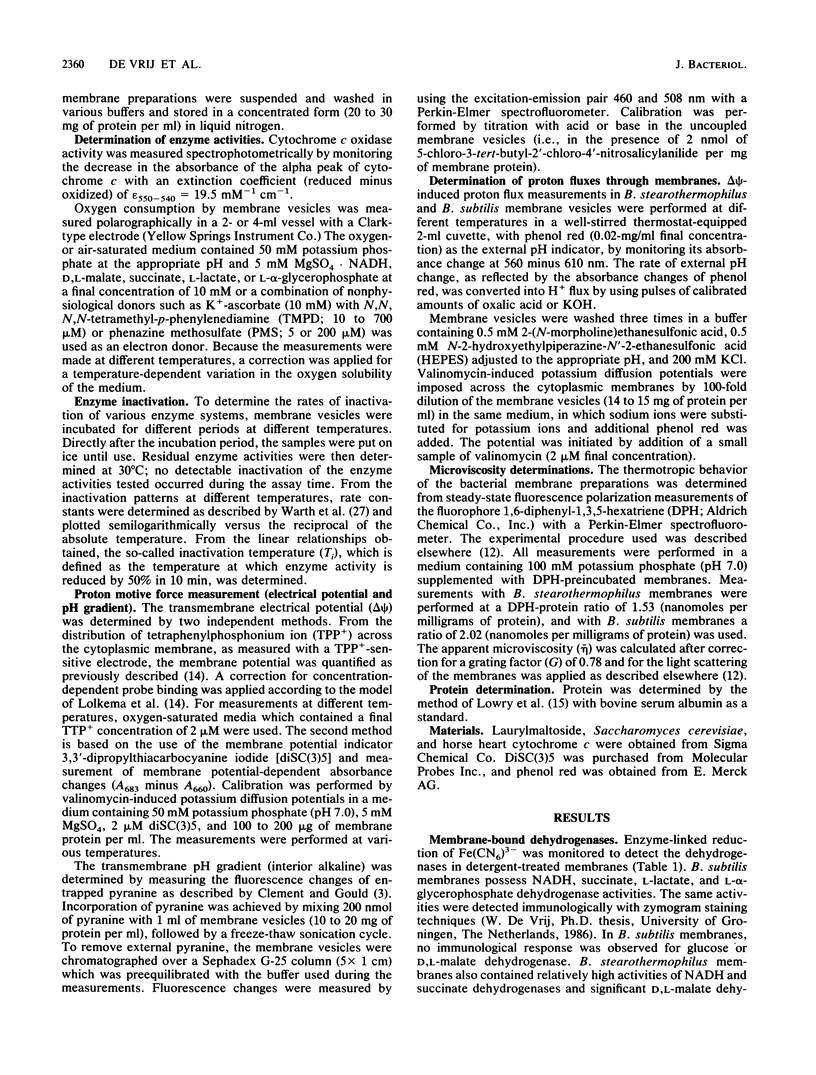
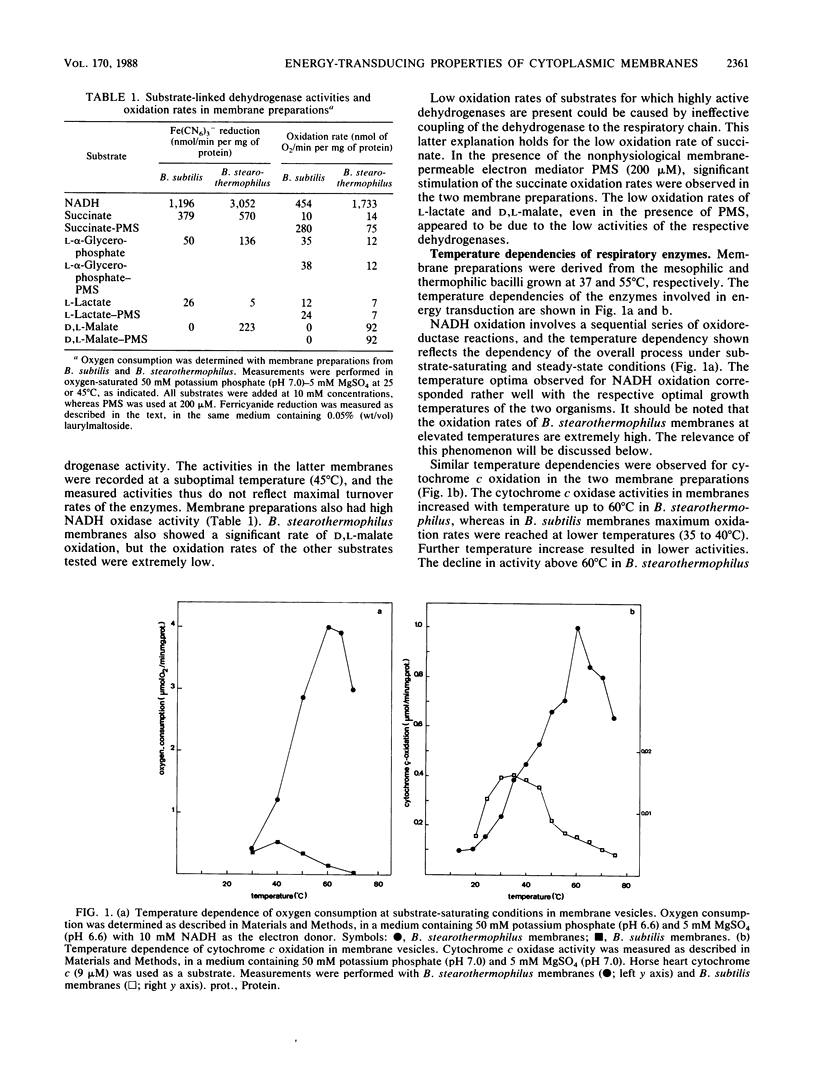
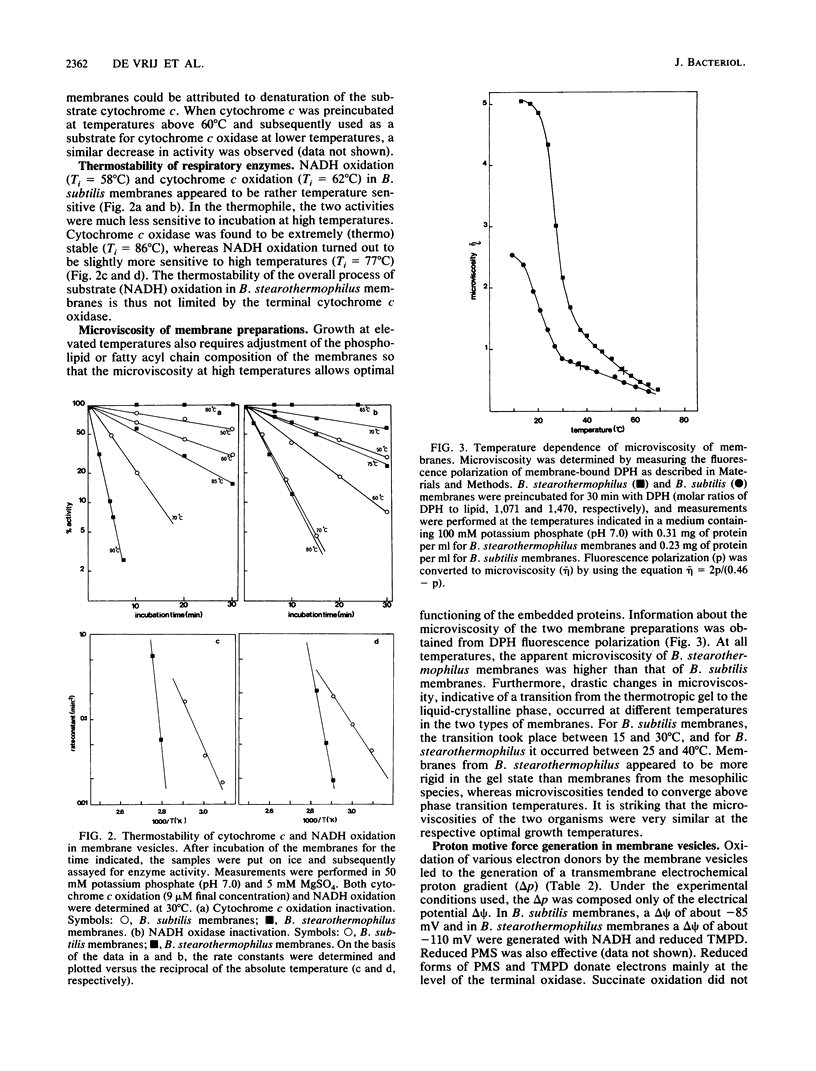
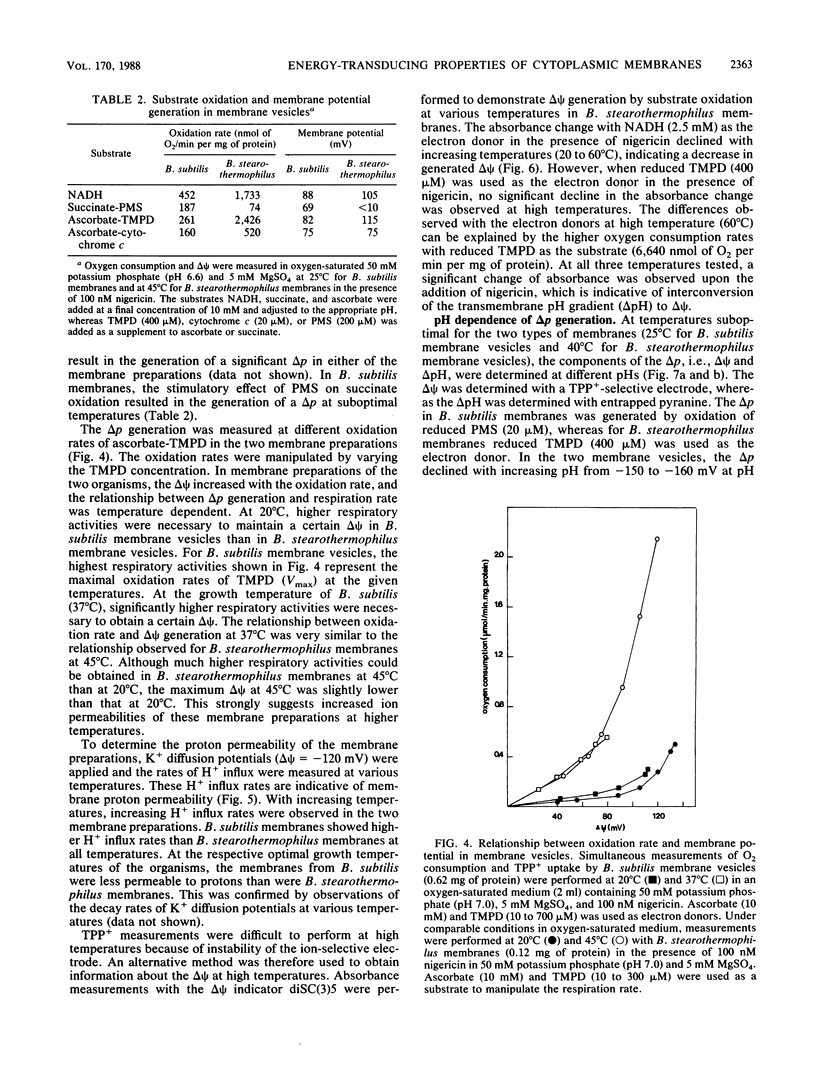
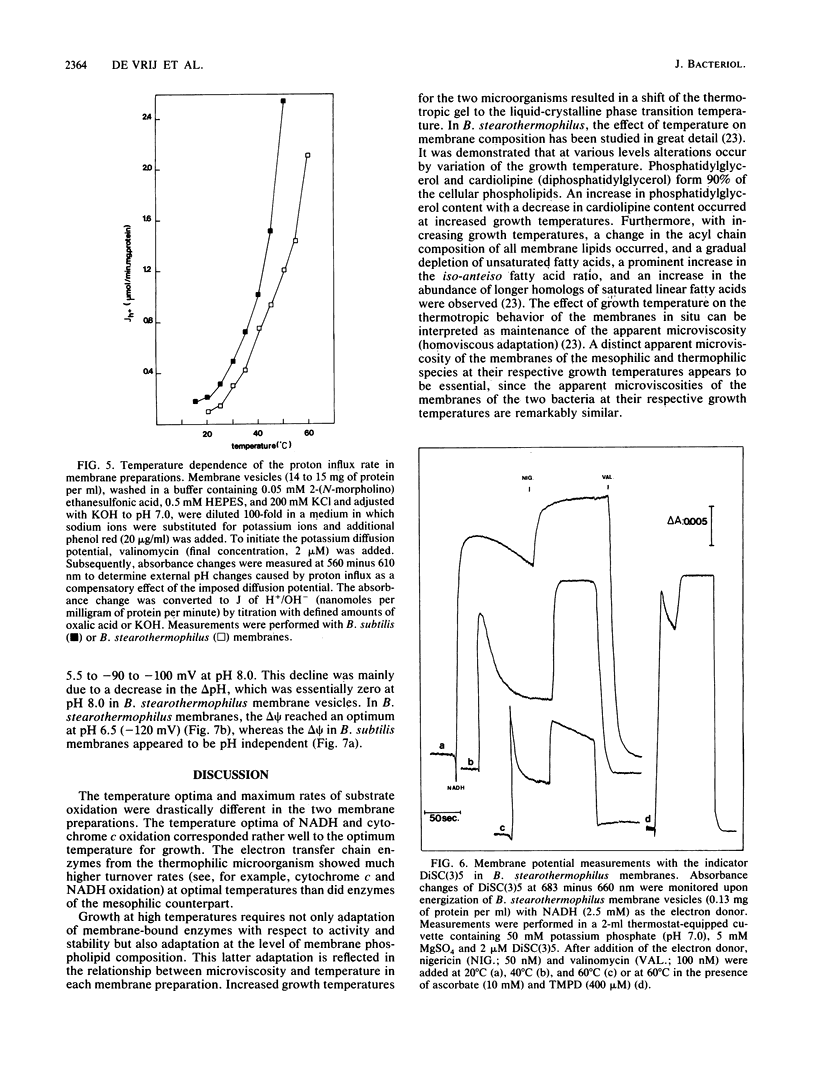
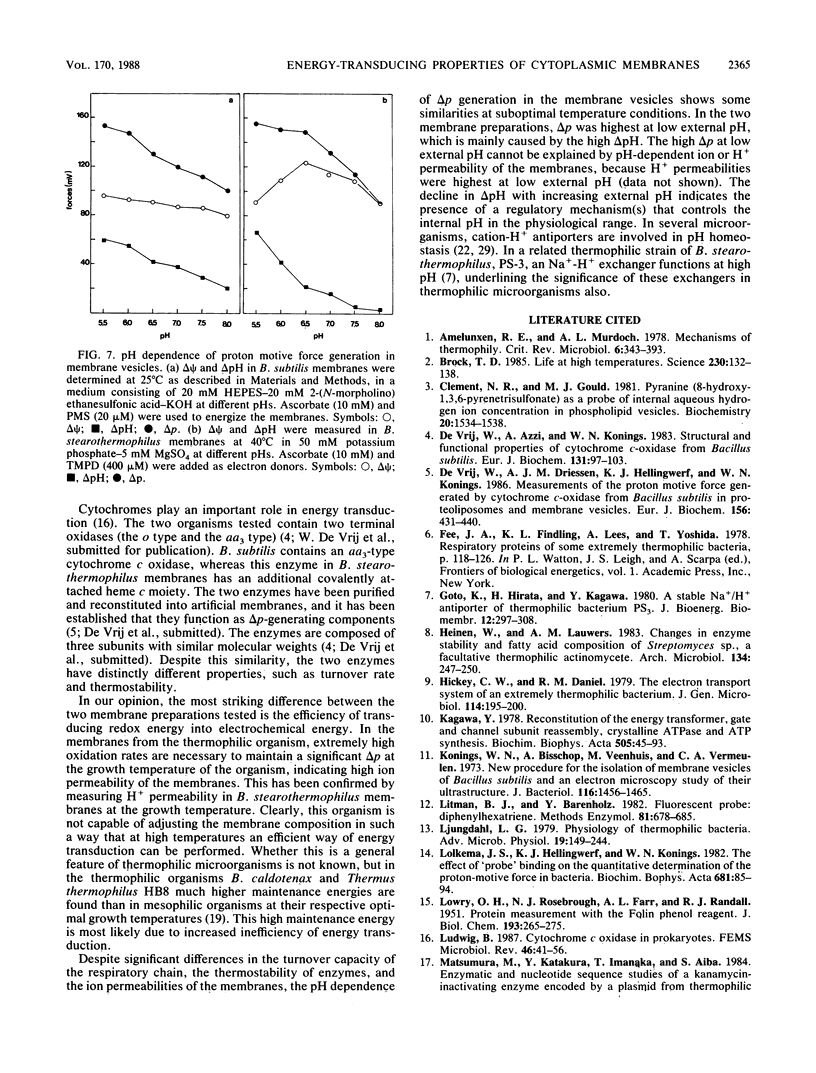
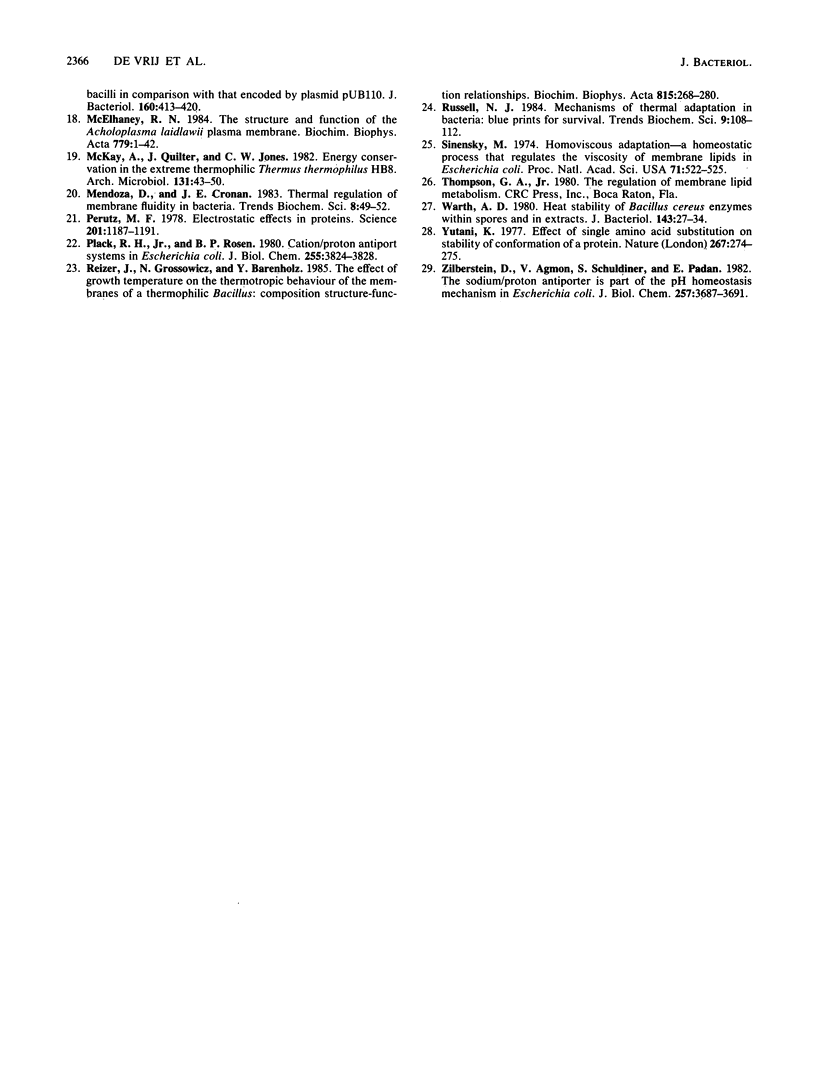
Selected References
These references are in PubMed. This may not be the complete list of references from this article.
- Amelunxen R. E., Murdock A. L. Mechanisms of thermophily. CRC Crit Rev Microbiol. 1978;6(4):343–393. doi: 10.3109/10408417809090626. [DOI] [PubMed] [Google Scholar]
- Brock T. D. Life at high temperatures. Science. 1985 Oct 11;230(4722):132–138. doi: 10.1126/science.230.4722.132. [DOI] [PubMed] [Google Scholar]
- Clement N. R., Gould J. M. Pyranine (8-hydroxy-1,3,6-pyrenetrisulfonate) as a probe of internal aqueous hydrogen ion concentration in phospholipid vesicles. Biochemistry. 1981 Mar 17;20(6):1534–1538. doi: 10.1021/bi00509a019. [DOI] [PubMed] [Google Scholar]
- De Vrij W., Azzi A., Konings W. N. Structural and functional properties of cytochrome c oxidase from Bacillus subtilis W23. Eur J Biochem. 1983 Mar 1;131(1):97–103. doi: 10.1111/j.1432-1033.1983.tb07235.x. [DOI] [PubMed] [Google Scholar]
- Goto K., Hirata H., Kagawa Y. A stable Na+/H+ antiporter of thermophilic bacterium PS3. J Bioenerg Biomembr. 1980 Aug;12(3-4):297–308. doi: 10.1007/BF00744690. [DOI] [PubMed] [Google Scholar]
- Heinen W., Lauwers A. M. Changes in enzyme stability and fatty acid composition of Streptomyces sp., a facultative thermophilic actinomycete. Arch Microbiol. 1983 Jun;134(3):247–250. doi: 10.1007/BF00407767. [DOI] [PubMed] [Google Scholar]
- Kagawa Y. Reconstitution of the energy transformer, gate and channel subunit reassembly, crystalline ATPase and ATP synthesis. Biochim Biophys Acta. 1978 Sep 21;505(1):45–93. doi: 10.1016/0304-4173(78)90008-3. [DOI] [PubMed] [Google Scholar]
- Konings W. N., Bisschop A., Veenhuis M., Vermeulen C. A. New procedure for the isolation of membrane vesicles of Bacillus subtilis and an electron microscopy study of their ultrastructure. J Bacteriol. 1973 Dec;116(3):1456–1465. doi: 10.1128/jb.116.3.1456-1465.1973. [DOI] [PMC free article] [PubMed] [Google Scholar]
- LOWRY O. H., ROSEBROUGH N. J., FARR A. L., RANDALL R. J. Protein measurement with the Folin phenol reagent. J Biol Chem. 1951 Nov;193(1):265–275. [PubMed] [Google Scholar]
- Litman B. J., Barenholz Y. Fluorescent probe: diphenylhexatriene. Methods Enzymol. 1982;81:678–685. doi: 10.1016/s0076-6879(82)81093-8. [DOI] [PubMed] [Google Scholar]
- Ljungdahl L. G. Physiology of thermophilic bacteria. Adv Microb Physiol. 1979;19:149–243. doi: 10.1016/s0065-2911(08)60199-x. [DOI] [PubMed] [Google Scholar]
- McElhaney R. N. The structure and function of the Acholeplasma laidlawii plasma membrane. Biochim Biophys Acta. 1984 Jan 27;779(1):1–42. doi: 10.1016/0304-4157(84)90002-9. [DOI] [PubMed] [Google Scholar]
- Perutz M. F. Electrostatic effects in proteins. Science. 1978 Sep 29;201(4362):1187–1191. doi: 10.1126/science.694508. [DOI] [PubMed] [Google Scholar]
- Plack R. H., Jr, Rosen B. P. Cation/proton antiport systems in Escherichia coli. Absence of potassium/proton antiporter activity in a pH-sensitive mutant. J Biol Chem. 1980 May 10;255(9):3824–3825. [PubMed] [Google Scholar]
- Reizer J., Grossowicz N., Barenholz Y. The effect of growth temperature on the thermotropic behavior of the membranes of a thermophilic Bacillus. Composition-structure-function relationships. Biochim Biophys Acta. 1985 May 14;815(2):268–280. doi: 10.1016/0005-2736(85)90297-4. [DOI] [PubMed] [Google Scholar]
- Sinensky M. Homeoviscous adaptation--a homeostatic process that regulates the viscosity of membrane lipids in Escherichia coli. Proc Natl Acad Sci U S A. 1974 Feb;71(2):522–525. doi: 10.1073/pnas.71.2.522. [DOI] [PMC free article] [PubMed] [Google Scholar]
- Warth A. D. Heat stability of Bacillus cereus enzymes within spores and in extracts. J Bacteriol. 1980 Jul;143(1):27–34. doi: 10.1128/jb.143.1.27-34.1980. [DOI] [PMC free article] [PubMed] [Google Scholar]
- Yutani K., Ogasahara K., Sugino Y., Matsushiro A. Effect of a single amino acid substitution on stability of conformation of a protein. Nature. 1977 May 19;267(5608):274–275. doi: 10.1038/267274a0. [DOI] [PubMed] [Google Scholar]
- Zilberstein D., Agmon V., Schuldiner S., Padan E. The sodium/proton antiporter is part of the pH homeostasis mechanism in Escherichia coli. J Biol Chem. 1982 Apr 10;257(7):3687–3691. [PubMed] [Google Scholar]
- de Vrij W., Driessen A. J., Hellingwerf K. J., Konings W. N. Measurements of the proton motive force generated by cytochrome c oxidase from Bacillus subtilis in proteoliposomes and membrane vesicles. Eur J Biochem. 1986 Apr 15;156(2):431–440. doi: 10.1111/j.1432-1033.1986.tb09600.x. [DOI] [PubMed] [Google Scholar]