Abstract
The rate at which Z. mobilis (Entner-Doudoroff pathway) converts high concentrations of glucose (20%) into ethanol plus CO2 changes as ethanol accumulates in the surrounding broth. This decline in glycolytic activity (per milligram of cell protein) does not result from inhibitory effects of ethanol, which can be reversed immediately by ethanol removal. The peak of fermentative activity (58 mumol of CO2 evolved per mg of cell protein per h) occurred after the accumulation of 1.1% ethanol (18 h) and declined to one-half this rate after 30 h (6.2% accumulated ethanol), although the cell number continued to increase. These times corresponded to the end of exponential growth and to the onset of the stationary phase (on the basis of measurement of cell protein), respectively. An examination of many of the requirements for fermentation (nucleotides, magnesium, enzyme levels, intracellular pH, delta pH) revealed three possible reasons for this early decline in activity: decreased abundance of nucleotides, a decrease in internal pH from 6.3 to 5.3, and a decrease in the specific activities of two glycolytic enzymes (pyruvate kinase and glyceraldehyde-3-phosphate dehydrogenase). 31P nuclear magnetic resonance spectra of perchlorate extracts from cells fermenting in broth revealed very low levels of glycolytic intermediates (Entner-Doudoroff pathway) in cells examined at the peak of fermentative activity (18-h cells) in comparison with cells examined at a later stage (30-h cells), consistent with limitation of the fermentation rate by glycolytic enzymes near the end of the pathway. It is likely that cell death (loss of colony-forming ability) and the collapse of delta pH also contribute to the further decline in fermentative activity after 30 h.
Full text
PDF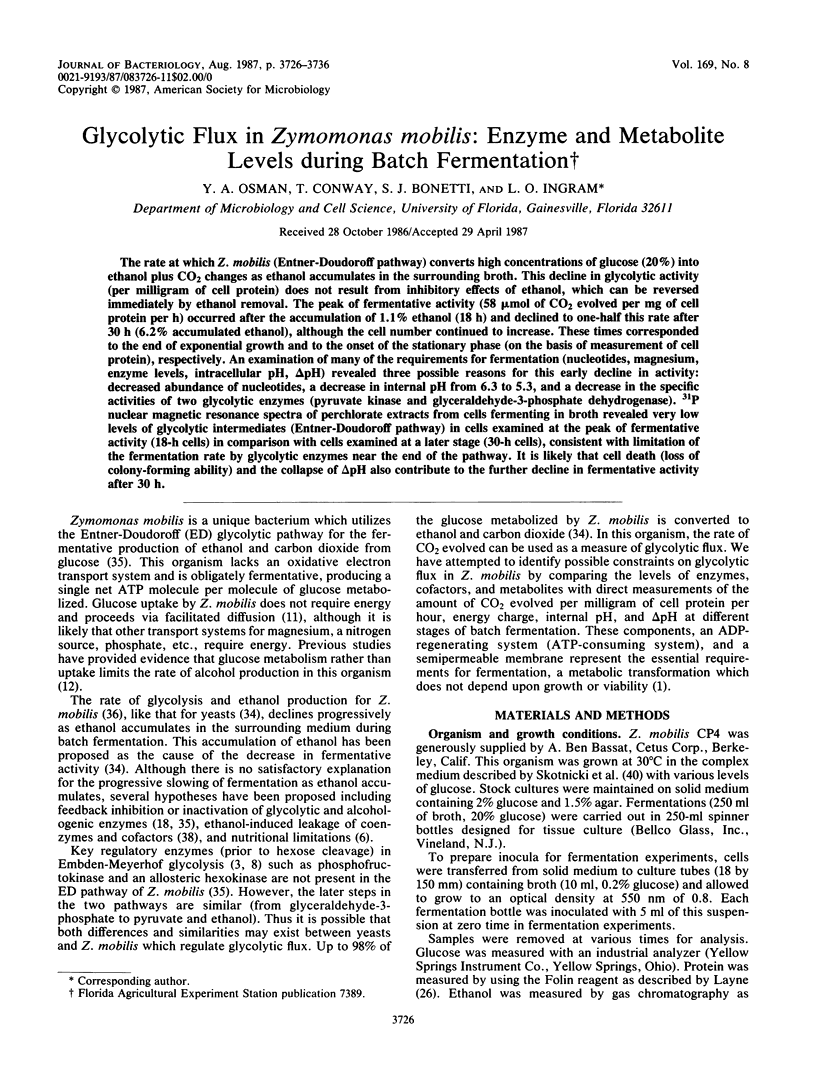
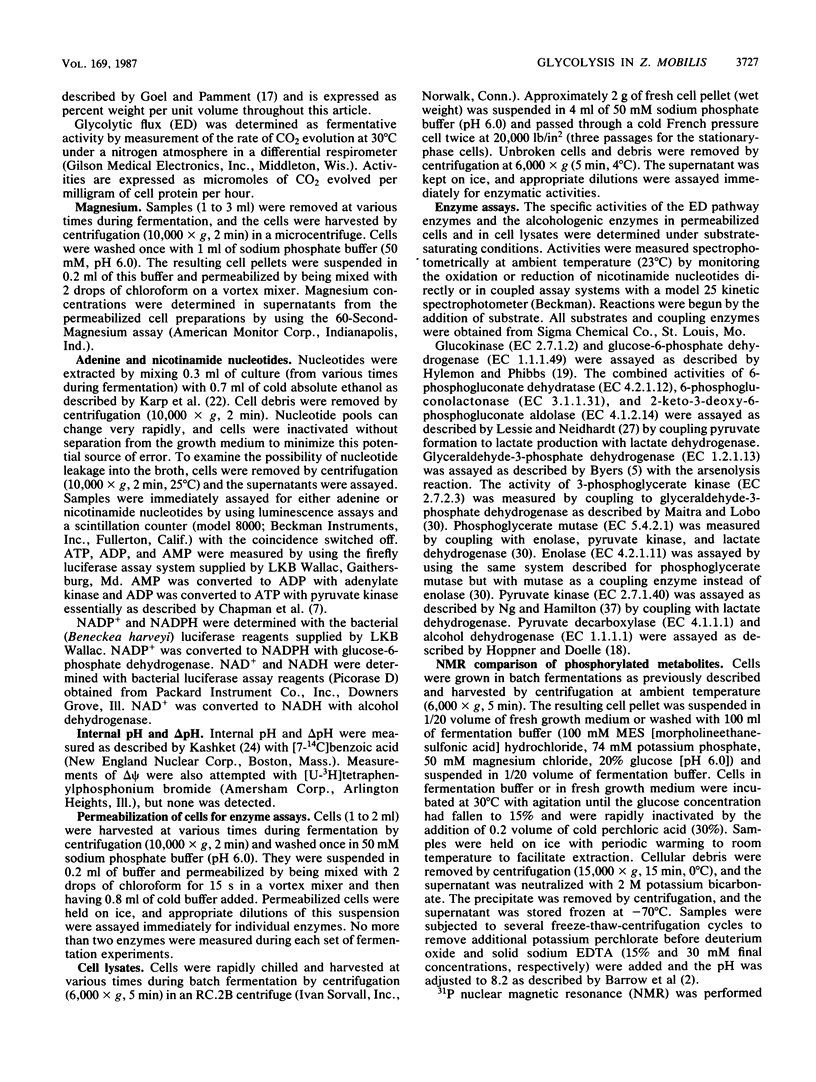
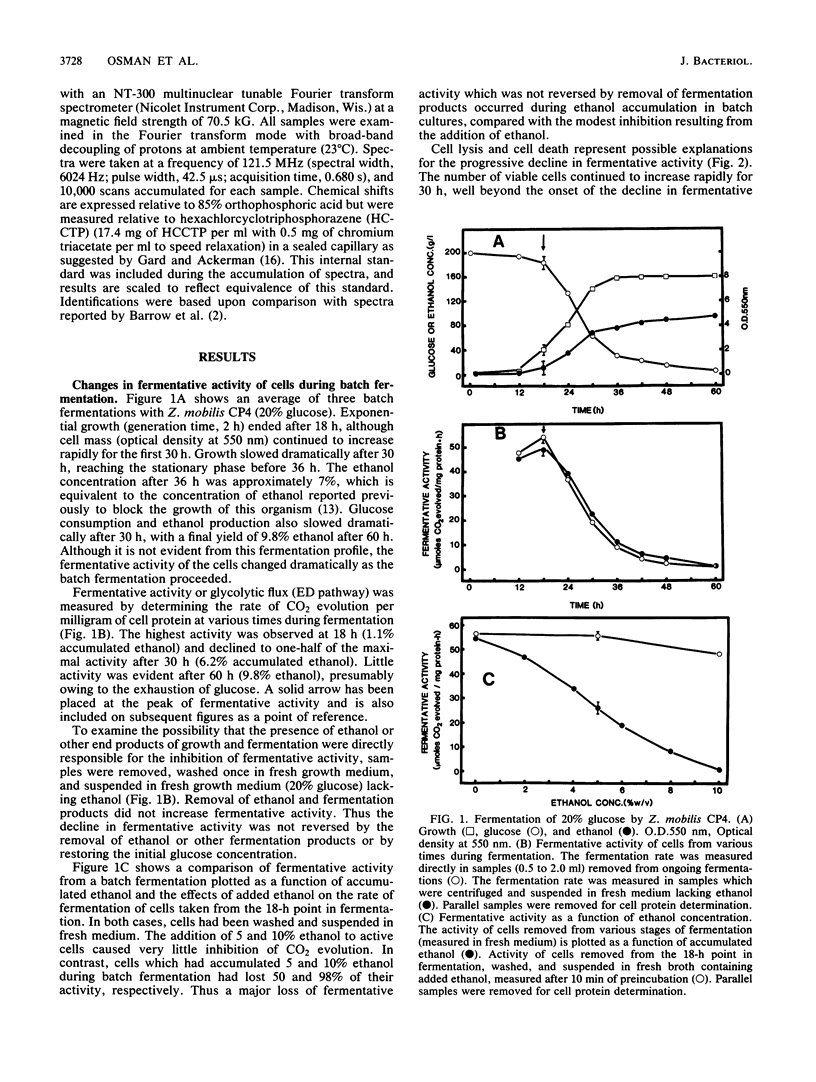
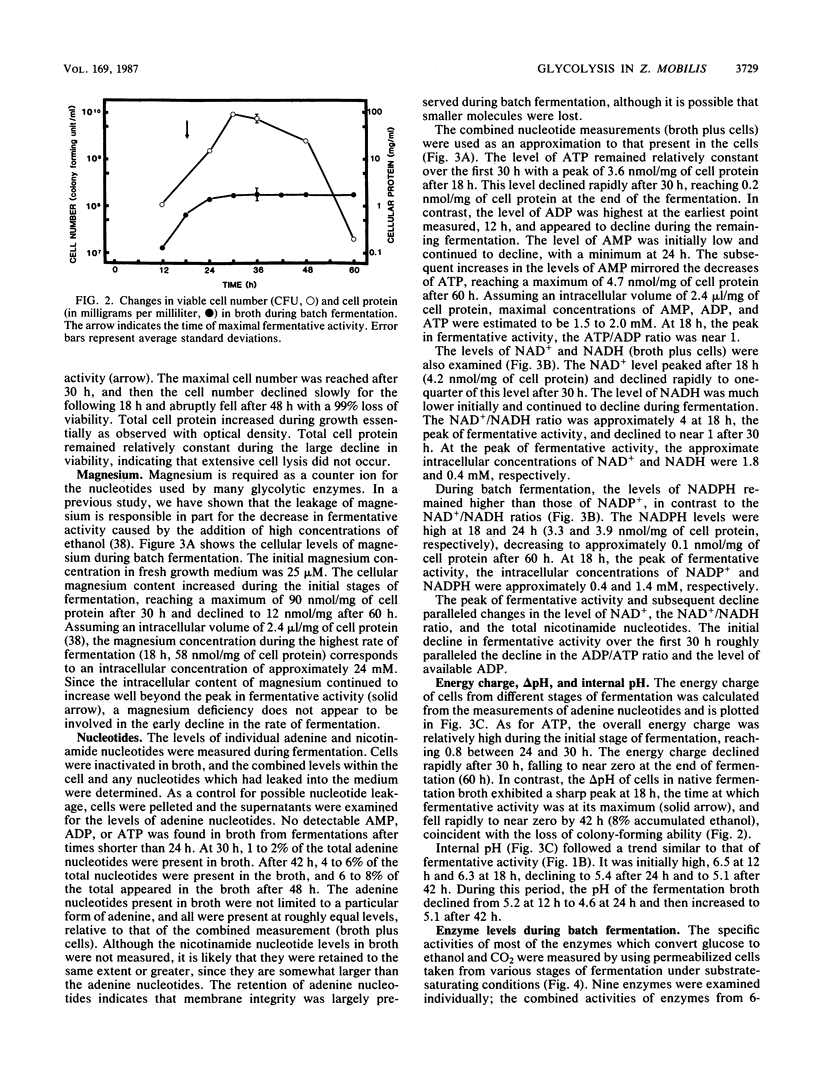
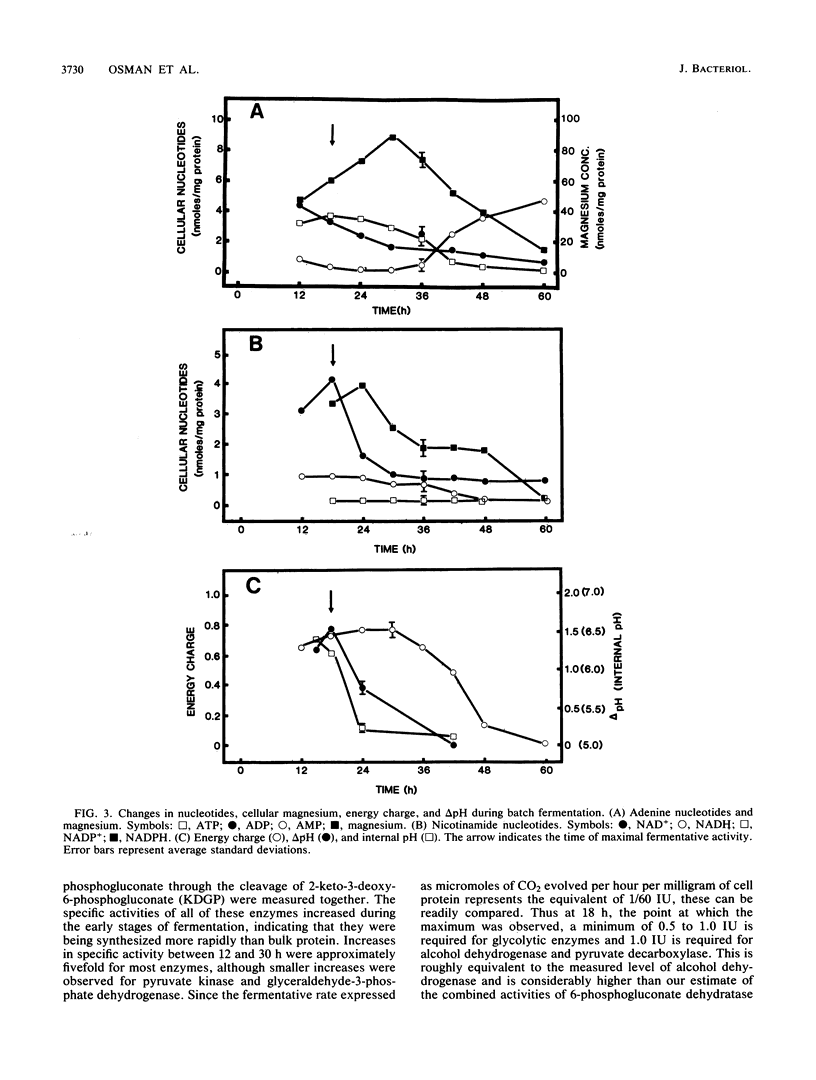
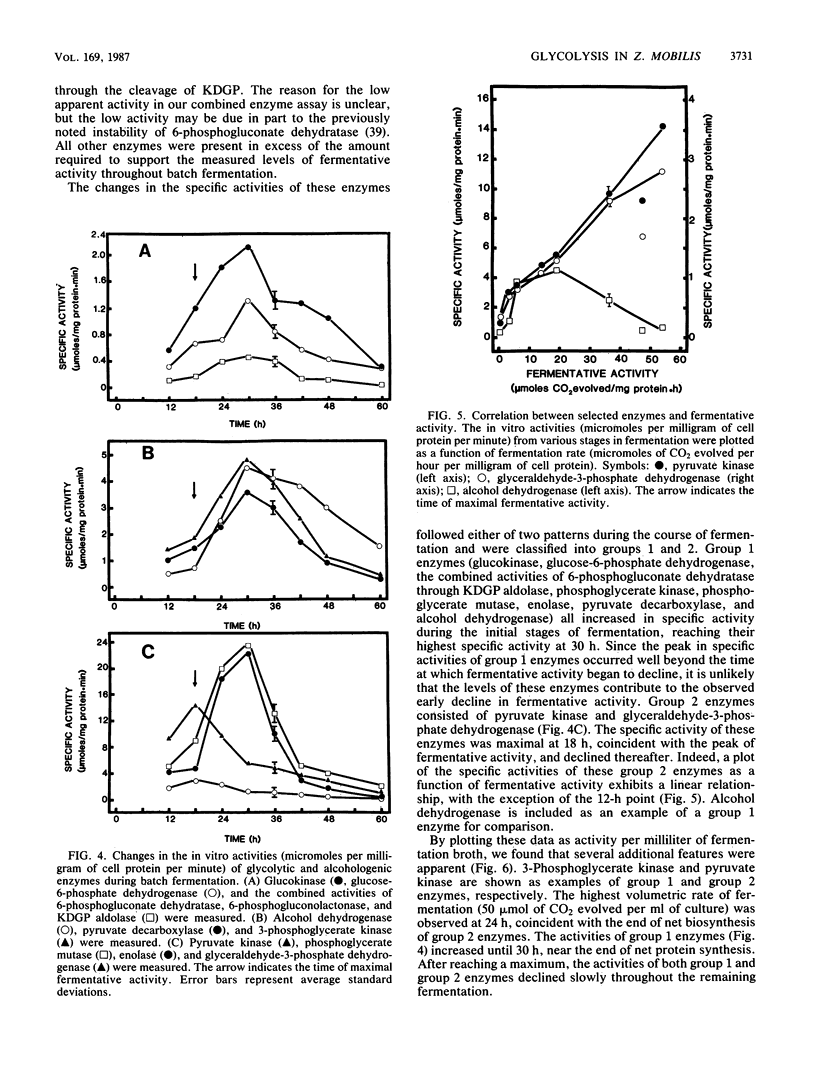
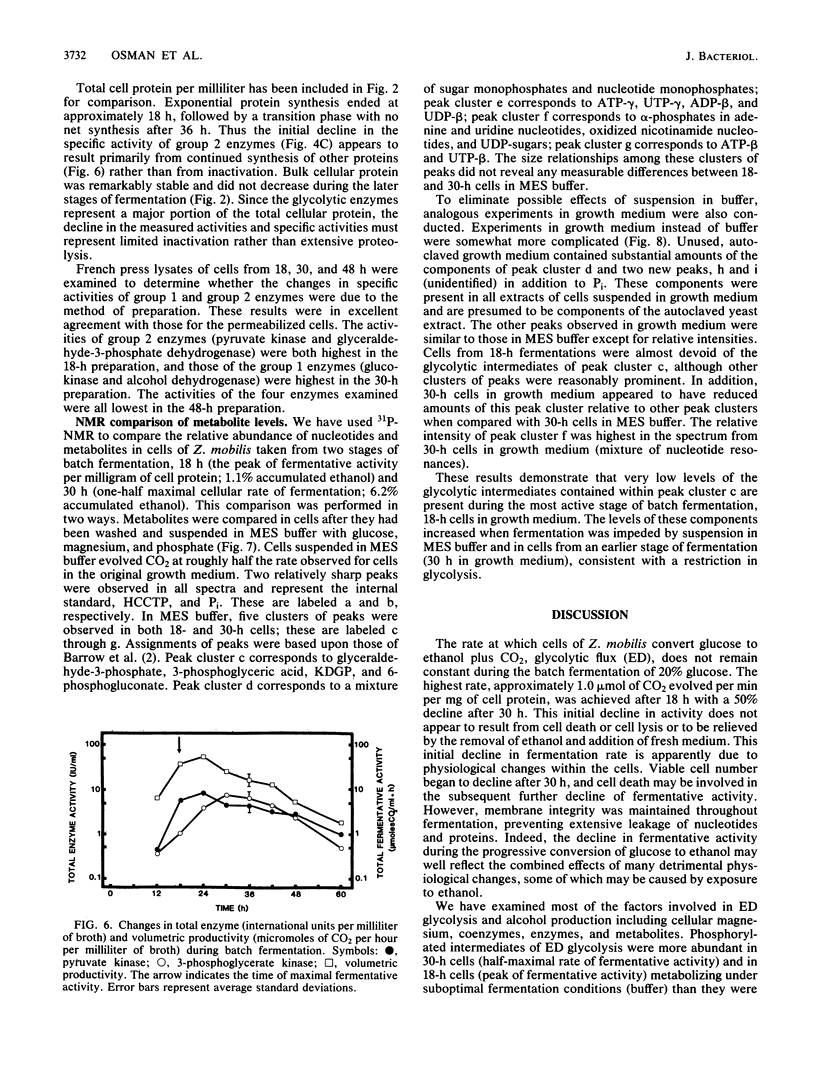
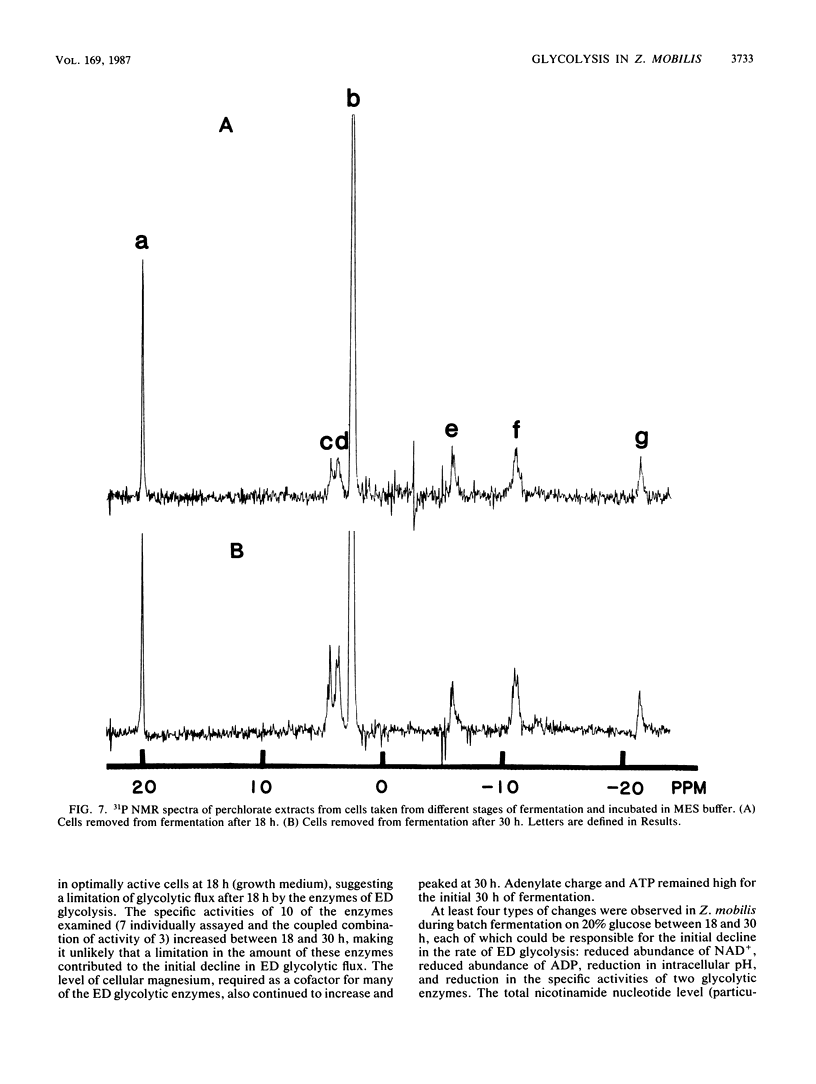
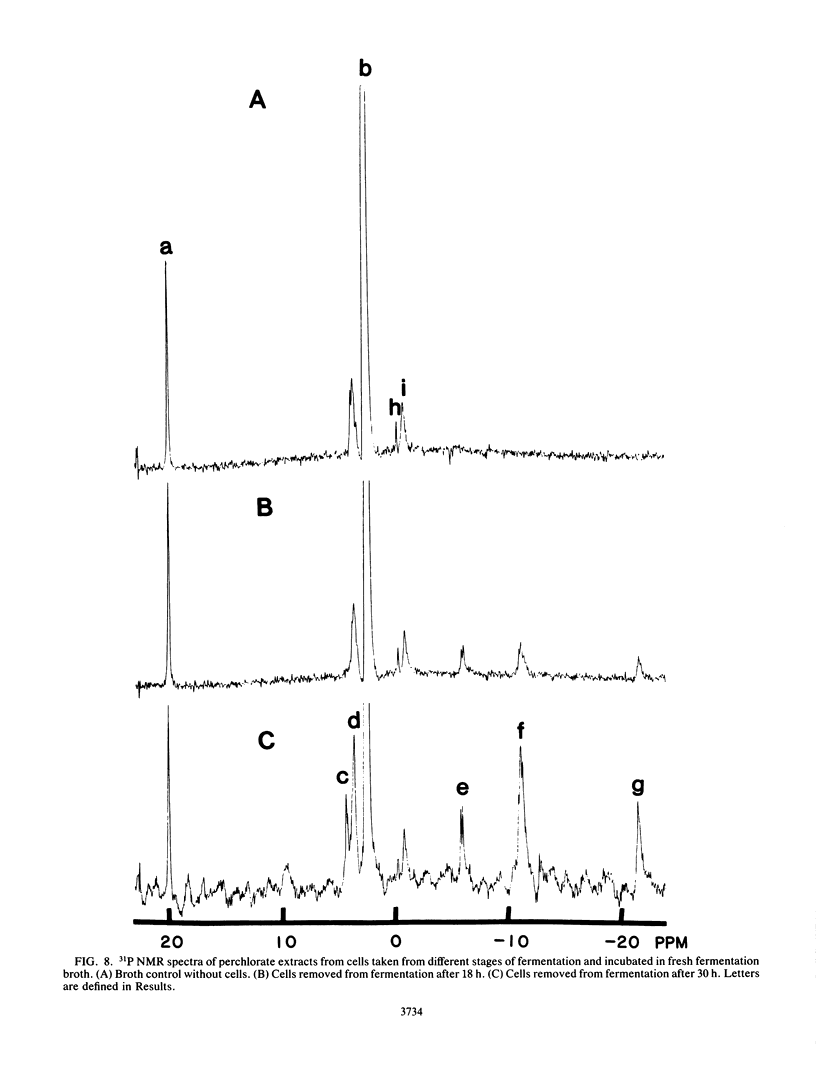
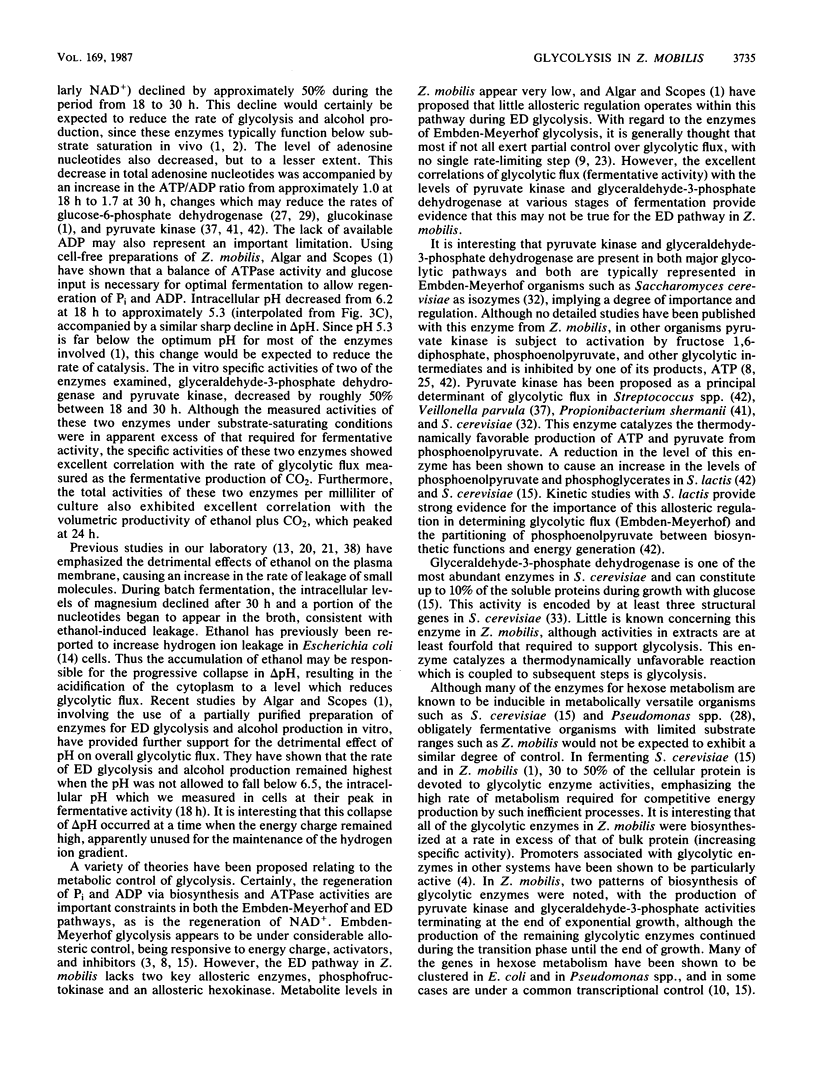
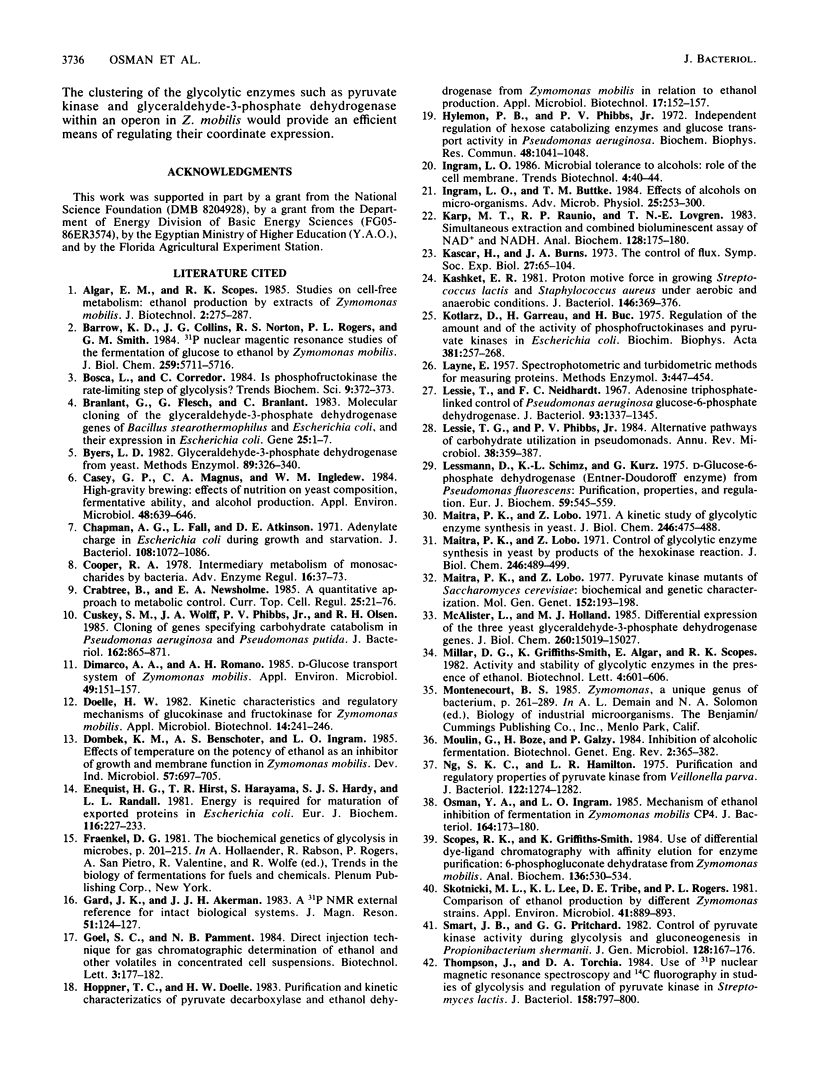
Selected References
These references are in PubMed. This may not be the complete list of references from this article.
- Barrow K. D., Collins J. G., Norton R. S., Rogers P. L., Smith G. M. 31P nuclear magnetic resonance studies of the fermentation of glucose to ethanol by Zymomonas mobilis. J Biol Chem. 1984 May 10;259(9):5711–5716. [PubMed] [Google Scholar]
- Branlant G., Flesch G., Branlant C. Molecular cloning of the glyceraldehyde-3-phosphate dehydrogenase genes of Bacillus stearothermophilus and Escherichia coli, and their expression in Escherichia coli. Gene. 1983 Nov;25(1):1–7. doi: 10.1016/0378-1119(83)90161-0. [DOI] [PubMed] [Google Scholar]
- Byers L. D. Glyceraldehyde-3-phosphate dehydrogenase from yeast. Methods Enzymol. 1982;89(Pt 500):326–335. doi: 10.1016/s0076-6879(82)89059-9. [DOI] [PubMed] [Google Scholar]
- Casey G. P., Magnus C. A., Ingledew W. M. High-gravity brewing: effects of nutrition on yeast composition, fermentative ability, and alcohol production. Appl Environ Microbiol. 1984 Sep;48(3):639–646. doi: 10.1128/aem.48.3.639-646.1984. [DOI] [PMC free article] [PubMed] [Google Scholar]
- Chapman A. G., Fall L., Atkinson D. E. Adenylate energy charge in Escherichia coli during growth and starvation. J Bacteriol. 1971 Dec;108(3):1072–1086. doi: 10.1128/jb.108.3.1072-1086.1971. [DOI] [PMC free article] [PubMed] [Google Scholar]
- Crabtree B., Newsholme E. A. A quantitative approach to metabolic control. Curr Top Cell Regul. 1985;25:21–76. doi: 10.1016/b978-0-12-152825-6.50006-0. [DOI] [PubMed] [Google Scholar]
- Cuskey S. M., Wolff J. A., Phibbs P. V., Jr, Olsen R. H. Cloning of genes specifying carbohydrate catabolism in Pseudomonas aeruginosa and Pseudomonas putida. J Bacteriol. 1985 Jun;162(3):865–871. doi: 10.1128/jb.162.3.865-871.1985. [DOI] [PMC free article] [PubMed] [Google Scholar]
- Dimarco A. A., Romano A. H. d-Glucose Transport System of Zymomonas mobilis. Appl Environ Microbiol. 1985 Jan;49(1):151–157. doi: 10.1128/aem.49.1.151-157.1985. [DOI] [PMC free article] [PubMed] [Google Scholar]
- Enequist H. G., Hirst T. R., Harayama S., Hardy S. J., Randall L. L. Energy is required for maturation of exported proteins in Escherichia coli. Eur J Biochem. 1981 May 15;116(2):227–233. doi: 10.1111/j.1432-1033.1981.tb05323.x. [DOI] [PubMed] [Google Scholar]
- Fraenkel D. G. The biochemical genetics of glycolysis in microbes. Basic Life Sci. 1981;18:201–215. doi: 10.1007/978-1-4684-3980-9_13. [DOI] [PubMed] [Google Scholar]
- Hylemon P. B., Phibbs P. V., Jr Independent regulation of hexose catabolizing enzymes and glucose transport activity in Pseudomonas aeruginosa. Biochem Biophys Res Commun. 1972 Sep 5;48(5):1041–1048. doi: 10.1016/0006-291x(72)90813-3. [DOI] [PubMed] [Google Scholar]
- Ingram L. O., Buttke T. M. Effects of alcohols on micro-organisms. Adv Microb Physiol. 1984;25:253–300. doi: 10.1016/s0065-2911(08)60294-5. [DOI] [PubMed] [Google Scholar]
- Kacser H., Burns J. A. The control of flux. Symp Soc Exp Biol. 1973;27:65–104. [PubMed] [Google Scholar]
- Karp M. T., Raunio R. P., Lövgren T. N. Simultaneous extraction and combined bioluminescent assay of NAD+ and NADH. Anal Biochem. 1983 Jan;128(1):175–180. doi: 10.1016/0003-2697(83)90359-7. [DOI] [PubMed] [Google Scholar]
- Kashket E. R. Proton motive force in growing Streptococcus lactis and Staphylococcus aureus cells under aerobic and anaerobic conditions. J Bacteriol. 1981 Apr;146(1):369–376. doi: 10.1128/jb.146.1.369-376.1981. [DOI] [PMC free article] [PubMed] [Google Scholar]
- Kotlarz D., Garreau H., Buc H. Regulation of the amount and of the activity of phosphofructokinases and pyruvate kinases in Escherichia coli. Biochim Biophys Acta. 1975 Feb 13;381(2):257–268. doi: 10.1016/0304-4165(75)90232-9. [DOI] [PubMed] [Google Scholar]
- Lessie T. G., Phibbs P. V., Jr Alternative pathways of carbohydrate utilization in pseudomonads. Annu Rev Microbiol. 1984;38:359–388. doi: 10.1146/annurev.mi.38.100184.002043. [DOI] [PubMed] [Google Scholar]
- Lessie T., Neidhardt F. C. Adenosine triphosphate-linked control of Pseudomonas aeruginosa glucose-6-phosphate dehydrogenase. J Bacteriol. 1967 Apr;93(4):1337–1345. doi: 10.1128/jb.93.4.1337-1345.1967. [DOI] [PMC free article] [PubMed] [Google Scholar]
- Lessmann D., Schimz K. L., Kurz G. D-glucose-6-phosphate dehydrogenase (Entner-Doudoroff enzyme) from Pseudomonas fluorescens. Purification, properties and regulation. Eur J Biochem. 1975 Nov 15;59(2):545–559. doi: 10.1111/j.1432-1033.1975.tb02481.x. [DOI] [PubMed] [Google Scholar]
- Maitra P. K., Lobo Z. A kinetic study of glycolytic enzyme synthesis in yeast. J Biol Chem. 1971 Jan 25;246(2):475–488. [PubMed] [Google Scholar]
- Maitra P. K., Lobo Z. Control of glycolytic enzyme synthesis in yeast by products of the hexokinase reaction. J Biol Chem. 1971 Jan 25;246(2):489–499. [PubMed] [Google Scholar]
- Maitra P. K., Lobo Z. Pyruvate kinase mutants of Saccharomyces cerevisiae: biochemical and genetic characterisation. Mol Gen Genet. 1977 Apr 29;152(3):193–200. doi: 10.1007/BF00268817. [DOI] [PubMed] [Google Scholar]
- McAlister L., Holland M. J. Differential expression of the three yeast glyceraldehyde-3-phosphate dehydrogenase genes. J Biol Chem. 1985 Dec 5;260(28):15019–15027. [PubMed] [Google Scholar]
- Ng S. K., Hamilton I. R. Purification and regulatory properties of pyruvate kinase from Veillonella parvula. J Bacteriol. 1975 Jun;122(3):1274–1282. doi: 10.1128/jb.122.3.1274-1282.1975. [DOI] [PMC free article] [PubMed] [Google Scholar]
- Osman Y. A., Ingram L. O. Mechanism of ethanol inhibition of fermentation in Zymomonas mobilis CP4. J Bacteriol. 1985 Oct;164(1):173–180. doi: 10.1128/jb.164.1.173-180.1985. [DOI] [PMC free article] [PubMed] [Google Scholar]
- Scopes R. K., Griffiths-Smith K. Use of differential dye-ligand chromatography with affinity elution for enzyme purification: 6-phosphogluconate dehydratase from Zymomonas mobilis. Anal Biochem. 1984 Feb;136(2):530–534. doi: 10.1016/0003-2697(84)90257-4. [DOI] [PubMed] [Google Scholar]
- Skotnicki M. L., Lee K. J., Tribe D. E., Rogers P. L. Comparison of ethanol production by different zymomonas strains. Appl Environ Microbiol. 1981 Apr;41(4):889–893. doi: 10.1128/aem.41.4.889-893.1981. [DOI] [PMC free article] [PubMed] [Google Scholar]
- Smart J. B., Pritchard G. G. Control of pyruvate kinase activity during glycolysis and gluconeogenesis in Propionibacterium shermanii. J Gen Microbiol. 1982 Jan;128(1):167–176. doi: 10.1099/00221287-128-1-167. [DOI] [PubMed] [Google Scholar]
- Thompson J., Torchia D. A. Use of 31P nuclear magnetic resonance spectroscopy and 14C fluorography in studies of glycolysis and regulation of pyruvate kinase in Streptococcus lactis. J Bacteriol. 1984 Jun;158(3):791–800. doi: 10.1128/jb.158.3.791-800.1984. [DOI] [PMC free article] [PubMed] [Google Scholar]