Abstract
O-antigen units are nonuniformly distributed among lipid A-core molecules in lipopolysaccharide (LPS) from gram-negative bacteria, as revealed by polyacrylamide gel electrophoresis in sodium dodecyl sulfate; the actual distribution patterns are complex, multimodal, and strain specific. Although the basic biochemical steps involved in synthesis and polymerization of O-antigen monomers and their subsequent attachment to lipid A-core are known, the mechanism by which specific multimodal distribution patterns are attained in mature LPS has not been previously considered theoretically or experimentally. We have developed probability equations which completely describe O-antigen distribution among lipid A-core molecules in terms of the probability of finding a nascent polymer (O antigen linked to carrier lipid) of length k (Tk) and the probability that a nascent polymer of length k will be extended to k + 1 by polymerase (pk) or transferred to lipid A-core by ligase (qk). These equations were used to show that multimodal distribution patterns in mature LPS cannot be produced if all pk are equal to p and all qk are equal to q, conditions which indicate a lack of selectivity of polymerase and ligase, respectively, for nascent O-antigen chain lengths. A completely stochastic model (pk = p, qk = q) of O-antigen polymerization and transfer to lipid A-core was also inconsistent with observed effects of mutations which resulted in partial inhibition of O-antigen monomer synthesis, lipid A-core synthesis, or ligase activity. The simplest explanation compatible with experimental observations is that polymerase or ligase, or perhaps both, have specificity for certain O-antigen chain lengths during biosynthesis of LPS. Our mathematical model indicates selectively probably was associated with the polymerase reaction. Although one may argue for a multimodal distribution pattern based on a kinetic mechanism i.e., varying reaction parameters in space or in time during cell growth, such a model requires complex sensory and regulatory mechanisms to explain the mutant data and mechanisms for sequestering specific components of LPS biosynthesis to explain the distribution pattern in normal cells. We favor the simple alternative of enzyme specificity and present generalized equations which should be useful in analysis of other analogous biochemical systems.
Full text
PDF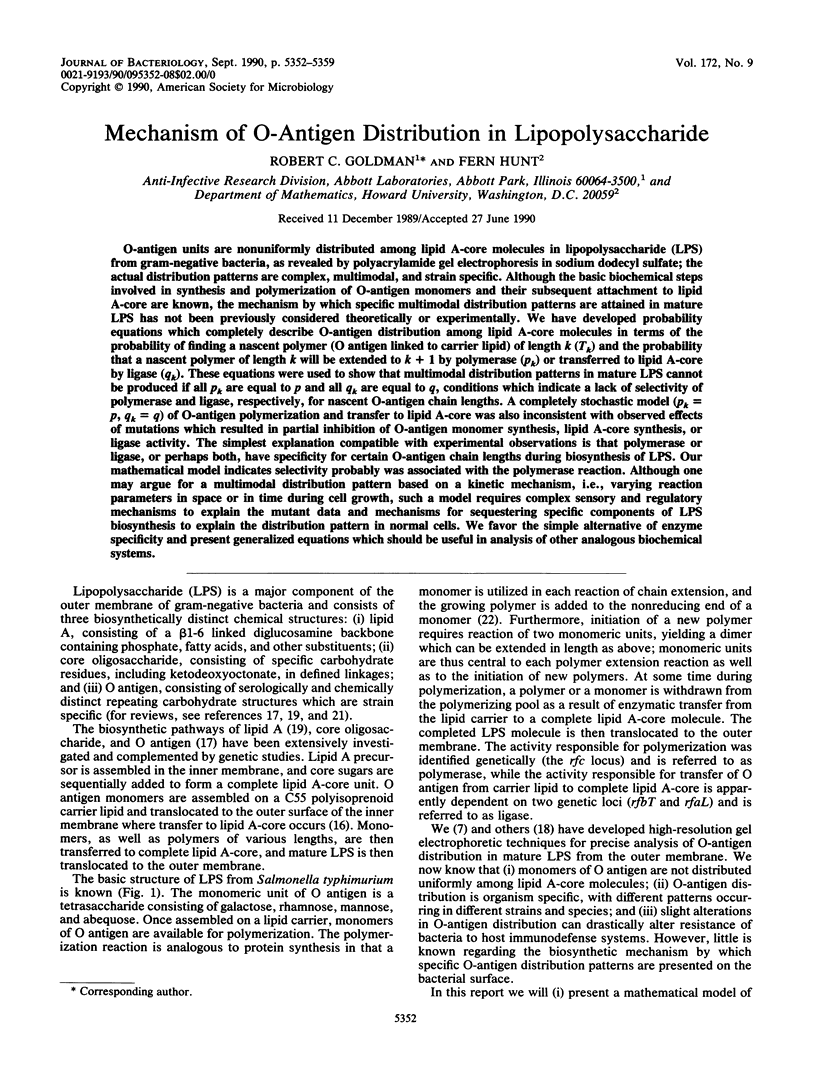
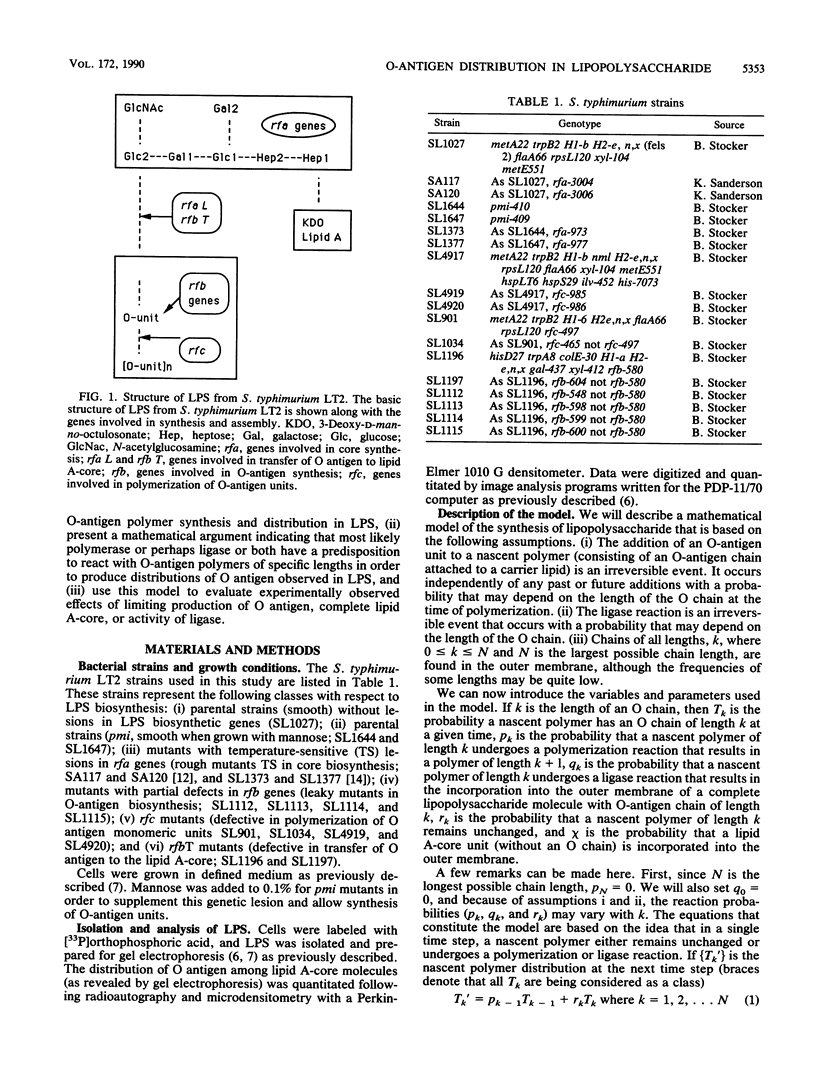
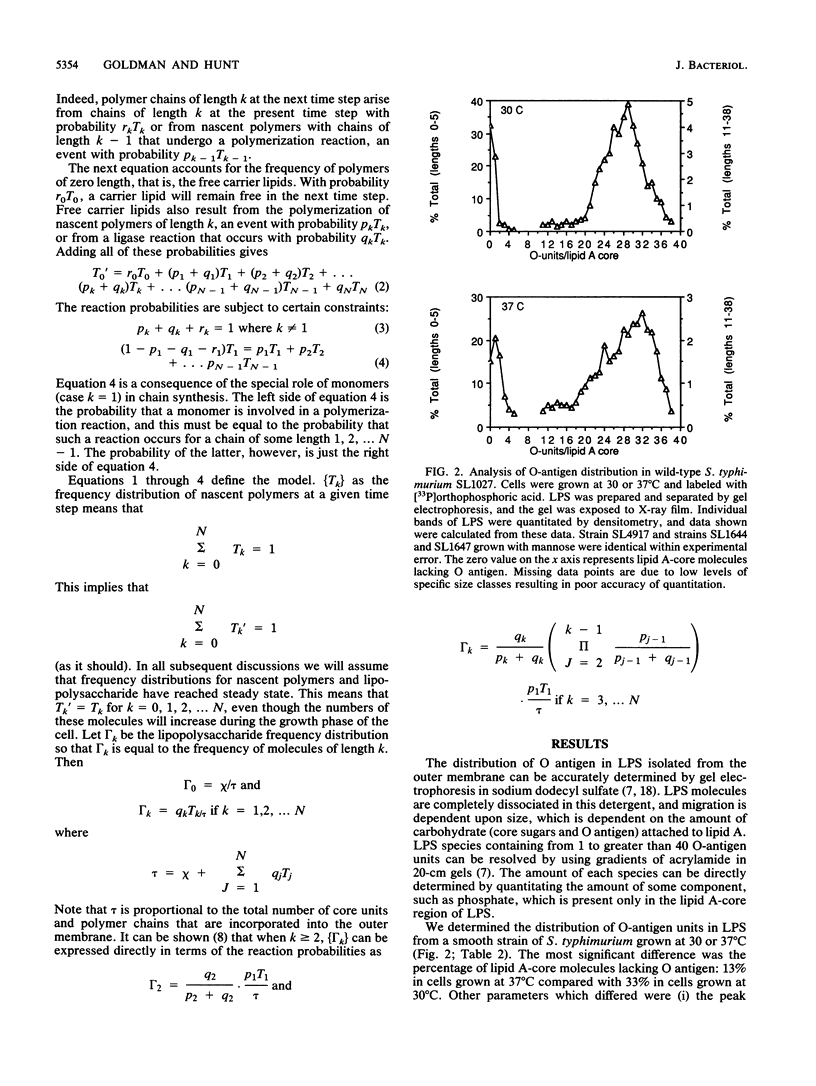
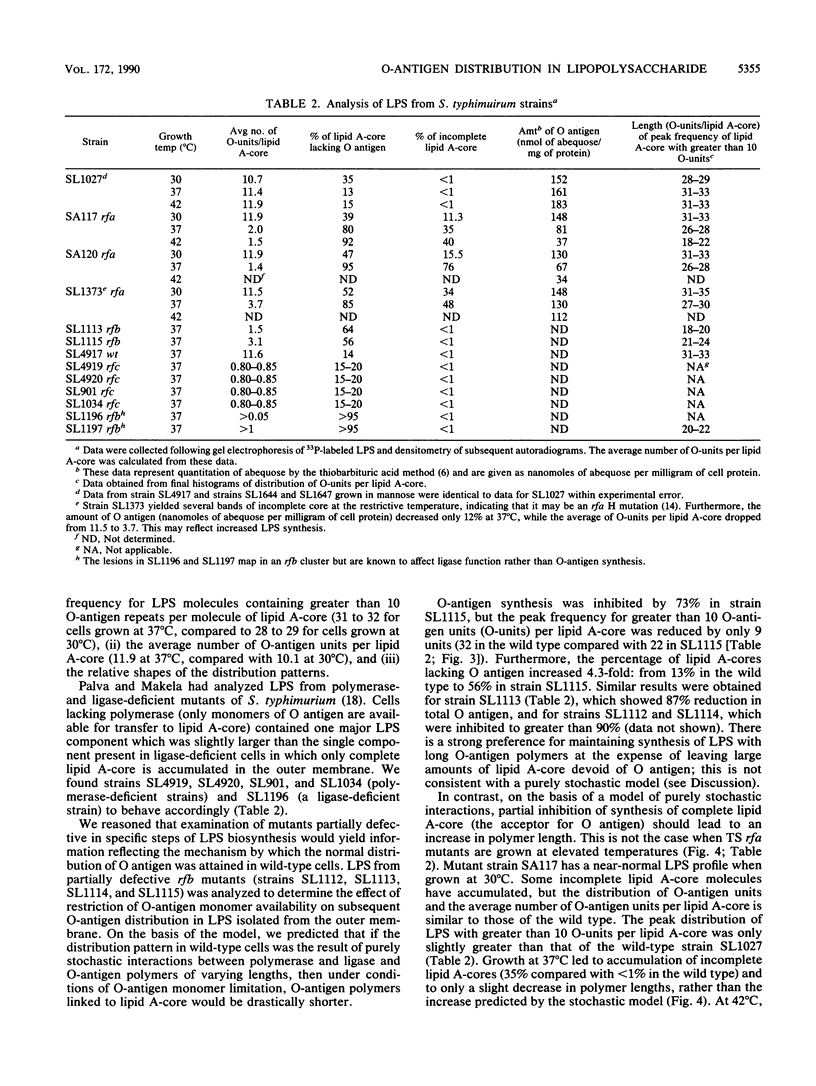
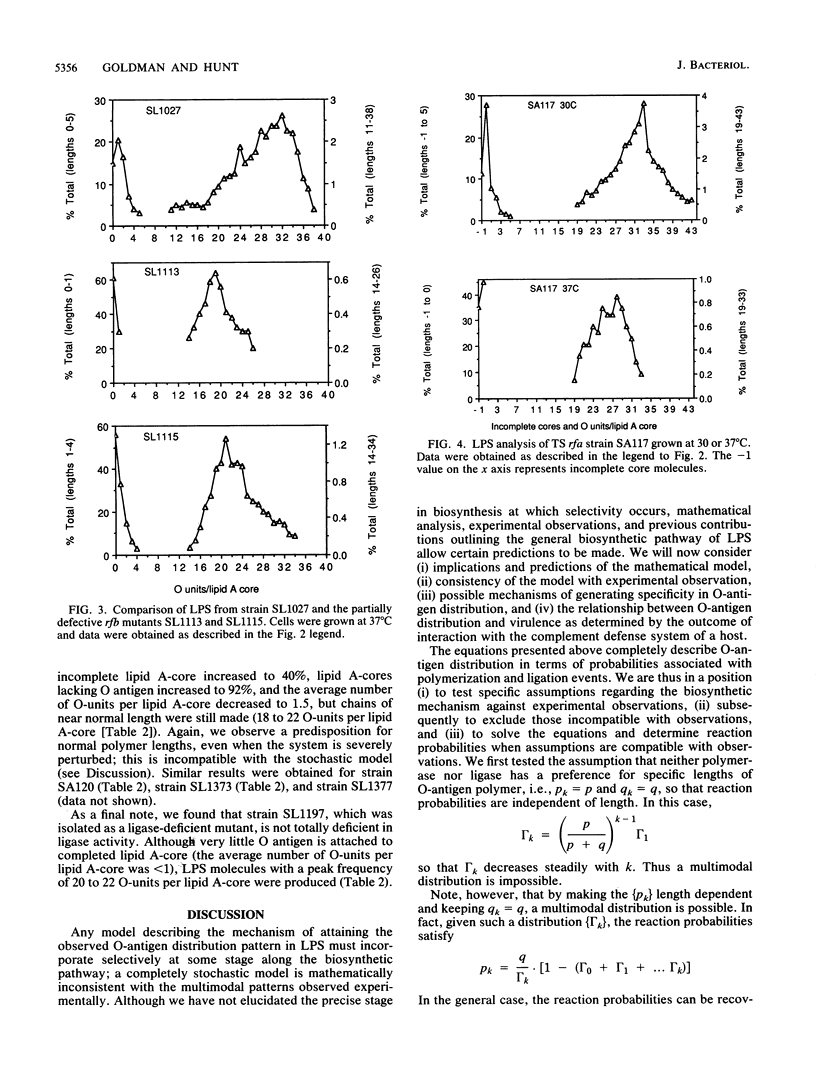
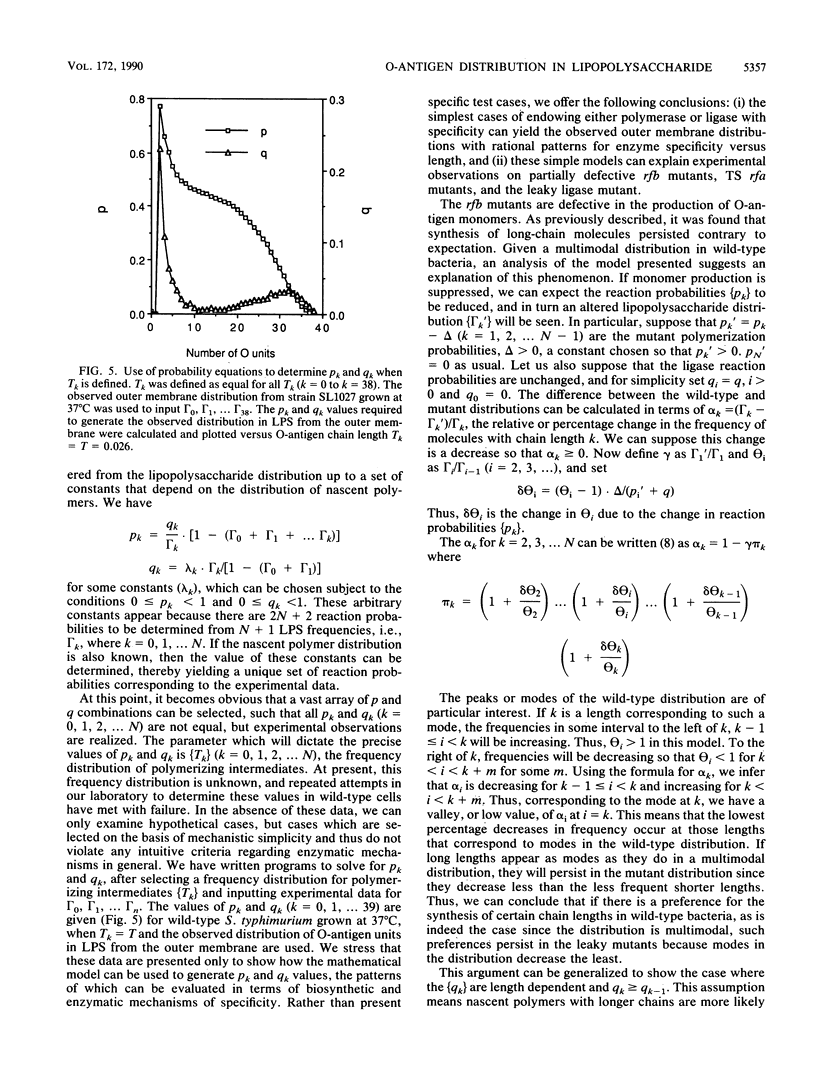
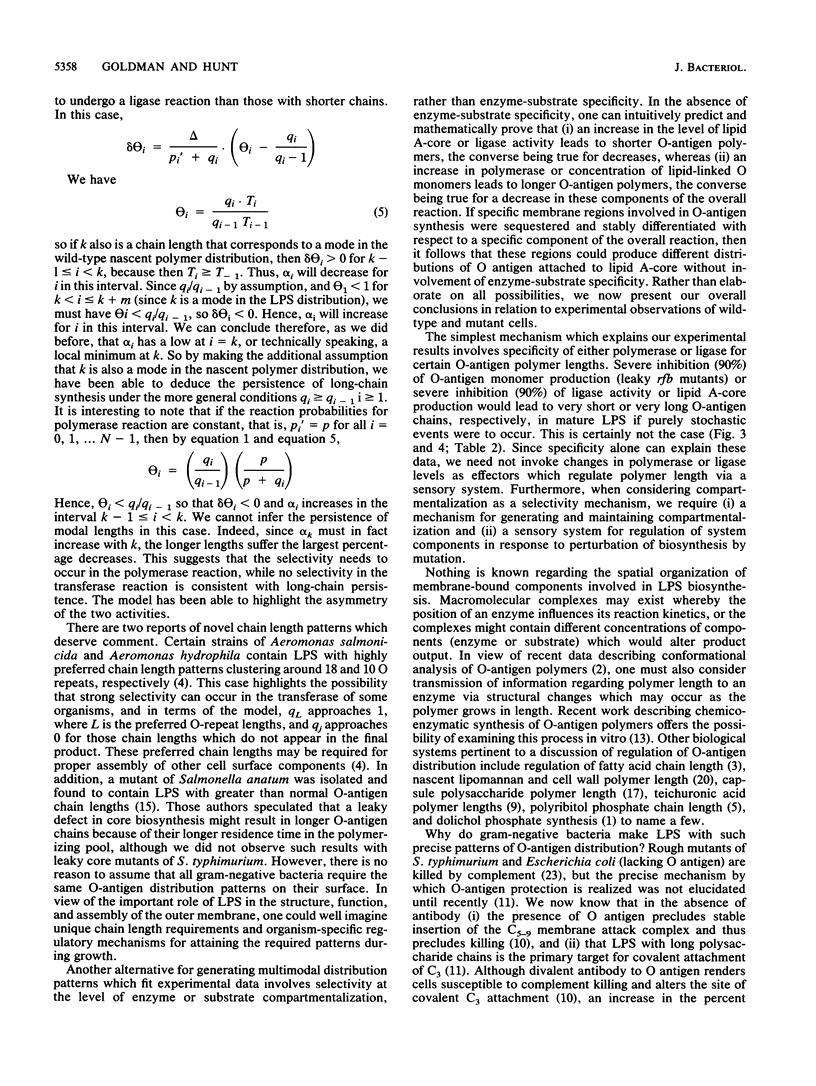
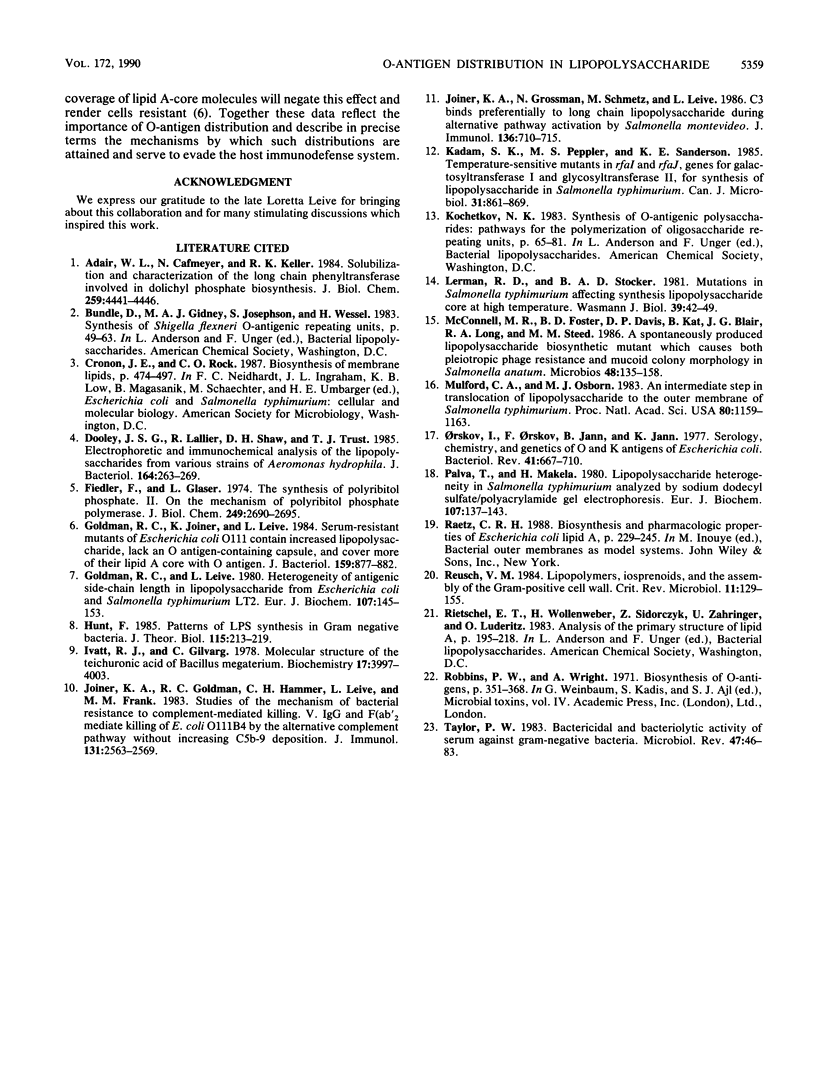
Selected References
These references are in PubMed. This may not be the complete list of references from this article.
- Adair W. L., Jr, Cafmeyer N., Keller R. K. Solubilization and characterization of the long chain prenyltransferase involved in dolichyl phosphate biosynthesis. J Biol Chem. 1984 Apr 10;259(7):4441–4446. [PubMed] [Google Scholar]
- Dooley J. S., Lallier R., Shaw D. H., Trust T. J. Electrophoretic and immunochemical analyses of the lipopolysaccharides from various strains of Aeromonas hydrophila. J Bacteriol. 1985 Oct;164(1):263–269. doi: 10.1128/jb.164.1.263-269.1985. [DOI] [PMC free article] [PubMed] [Google Scholar]
- Fiedler F., Glaser L. The synthesis of polyribitol phosphate. II. On the mechanism of polyribitol phosphate polymerase. J Biol Chem. 1974 May 10;249(9):2690–2695. [PubMed] [Google Scholar]
- Goldman R. C., Joiner K., Leive L. Serum-resistant mutants of Escherichia coli O111 contain increased lipopolysaccharide, lack an O antigen-containing capsule, and cover more of their lipid A core with O antigen. J Bacteriol. 1984 Sep;159(3):877–882. doi: 10.1128/jb.159.3.877-882.1984. [DOI] [PMC free article] [PubMed] [Google Scholar]
- Goldman R. C., Leive L. Heterogeneity of antigenic-side-chain length in lipopolysaccharide from Escherichia coli 0111 and Salmonella typhimurium LT2. Eur J Biochem. 1980;107(1):145–153. doi: 10.1111/j.1432-1033.1980.tb04635.x. [DOI] [PubMed] [Google Scholar]
- Hunt F. Patterns of LPS synthesis in gram negative bacteria. J Theor Biol. 1985 Jul 21;115(2):213–219. doi: 10.1016/s0022-5193(85)80097-7. [DOI] [PubMed] [Google Scholar]
- Ivatt R. J., Gilvarg C. Molecular structure of the teichuronic acid of Bacillus megaterium. Biochemistry. 1978 Sep 19;17(19):3997–4003. doi: 10.1021/bi00612a019. [DOI] [PubMed] [Google Scholar]
- Joiner K. A., Goldman R. C., Hammer C. H., Leive L., Frank M. M. Studies of the mechanism of bacterial resistance to complement-mediated killing. V. IgG and F(ab')2 mediate killing of E. coli 0111B4 by the alternative complement pathway without increasing C5b-9 deposition. J Immunol. 1983 Nov;131(5):2563–2569. [PubMed] [Google Scholar]
- Joiner K. A., Grossman N., Schmetz M., Leive L. C3 binds preferentially to long-chain lipopolysaccharide during alternative pathway activation by Salmonella montevideo. J Immunol. 1986 Jan;136(2):710–715. [PubMed] [Google Scholar]
- Kadam S. K., Peppler M. S., Sanderson K. E. Temperature-sensitive mutants in rfaI and rfaJ, genes for galactosyltransferase I and glucosyltransferase II, for synthesis of lipopolysaccharide in Salmonella typhimurium. Can J Microbiol. 1985 Sep;31(9):861–869. doi: 10.1139/m85-160. [DOI] [PubMed] [Google Scholar]
- McConnell M. R., Foster B. D., Davis D. P., Kat B., Blair J. G., Long R. A., Steed M. M. A spontaneously produced lipopolysaccharide biosynthetic defect which causes both pleiotropic phage resistance and mucoid colony morphology in Salmonella anatum. Microbios. 1986;48(196-197):135–158. [PubMed] [Google Scholar]
- Mulford C. A., Osborn M. J. An intermediate step in translocation of lipopolysaccharide to the outer membrane of Salmonella typhimurium. Proc Natl Acad Sci U S A. 1983 Mar;80(5):1159–1163. doi: 10.1073/pnas.80.5.1159. [DOI] [PMC free article] [PubMed] [Google Scholar]
- Orskov I., Orskov F., Jann B., Jann K. Serology, chemistry, and genetics of O and K antigens of Escherichia coli. Bacteriol Rev. 1977 Sep;41(3):667–710. doi: 10.1128/br.41.3.667-710.1977. [DOI] [PMC free article] [PubMed] [Google Scholar]
- Palva E. T., Mäkelä P. H. Lipopolysaccharide heterogeneity in Salmonella typhimurium analyzed by sodium dodecyl sulfate polyacrylamide gel electrophoresis. Eur J Biochem. 1980;107(1):137–143. doi: 10.1111/j.1432-1033.1980.tb04634.x. [DOI] [PubMed] [Google Scholar]
- Reusch V. M., Jr Lipopolymers, isoprenoids, and the assembly of the gram-positive cell wall. Crit Rev Microbiol. 1984;11(2):129–155. doi: 10.3109/10408418409105475. [DOI] [PubMed] [Google Scholar]
- Taylor P. W. Bactericidal and bacteriolytic activity of serum against gram-negative bacteria. Microbiol Rev. 1983 Mar;47(1):46–83. doi: 10.1128/mr.47.1.46-83.1983. [DOI] [PMC free article] [PubMed] [Google Scholar]