Abstract
The phosphoenolpyruvate-D-glucose phosphotransferase system of Enterobacteriaceae is thought to regulate the synthesis and activity of a number of catabolite uptake systems, including those for maltose, lactose, and glycerol, via the phosphorylation state of one of its components, IIIGlc. We have investigated the proposal by Kornberg and co-workers (FEBS Lett. 117(Suppl.):K28-K36, 1980) that not IIIGlc, but an unknown protein, the product of the iex gene, is responsible for the exclusion of the above-mentioned compounds from the cell. The iex mutant HK738 of Escherichia coli contains normal amounts of IIIGlc as measured by specific antibodies, in contrast to crr mutants that lack IIIGlc. The IIIGlc of the iex strain functions normally in glucose and methyl alpha-glucoside transport, and the specific activity in in vitro phosphorylation is approximately 60% of that of the parent. The IIIGlc activity of the iex strain is, however, heat labile, in contrast to the parental IIIGlc, suggesting that the mutant contains an altered IIIGlc. This is supported by the observation that IIIGlc from the iex strain cannot bind to the lactose carrier. Thus it cannot inhibit the carrier, and this explains why the uptake of non-phosphotransferase system compounds in an iex strain is resistant to phosphotransferase system sugars. The introduction of a plasmid containing a wild-type crr+ allele into the iex strain restores the iex phenotype to that of the iex+ parent. The IIIGlc produced from the plasmid in the iex strain is heat stable and binds normally to the lactose carrier. These results lead to the conclusion that the iex mutation is most likely allelic with crr and results in an altered, temperature-sensitive IIIGlc that is still able to function D-glucose and methyl alpha-glucoside uptake and phosphorylation and in the activation of adenylate cyclase, but is unable to bind to and inhibit the lactose carrier.
Full text
PDF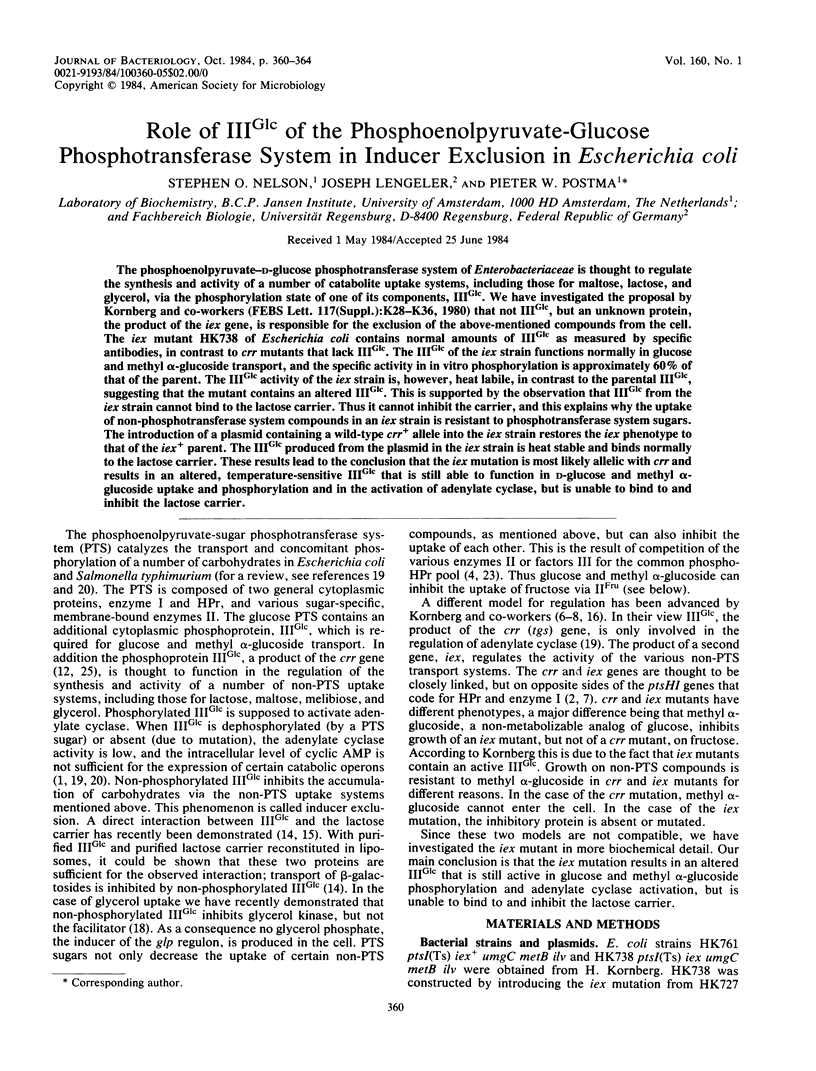
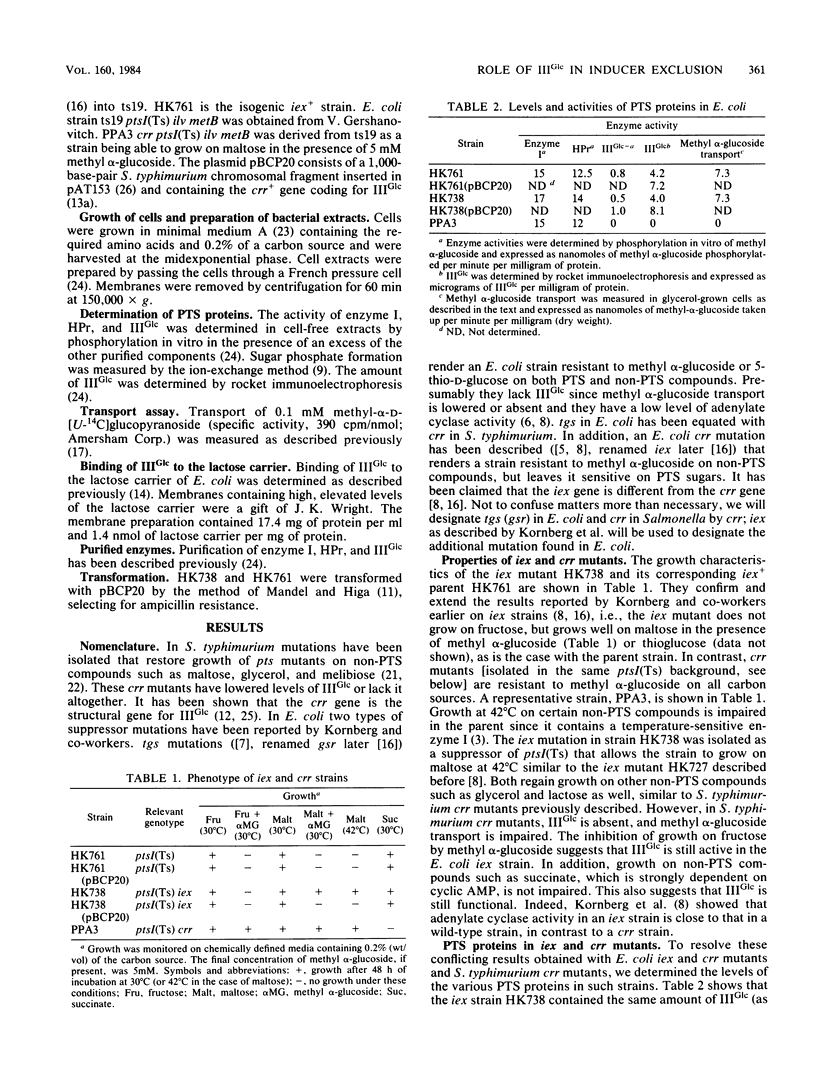
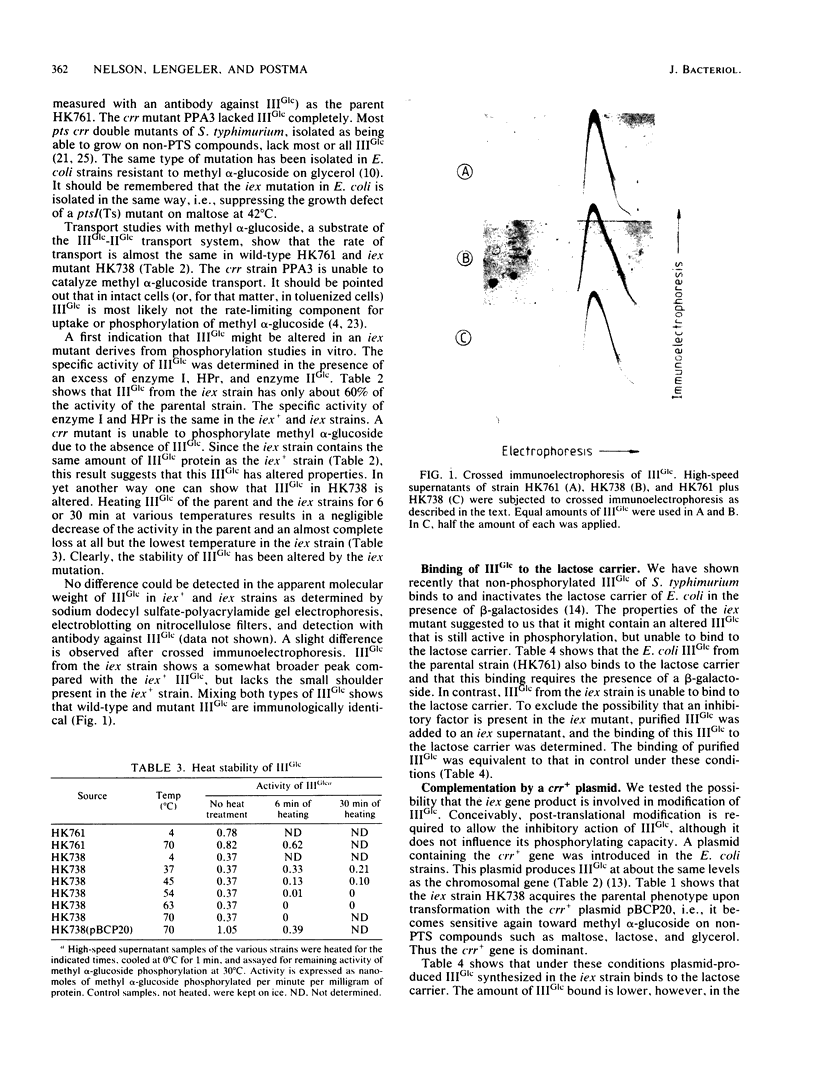
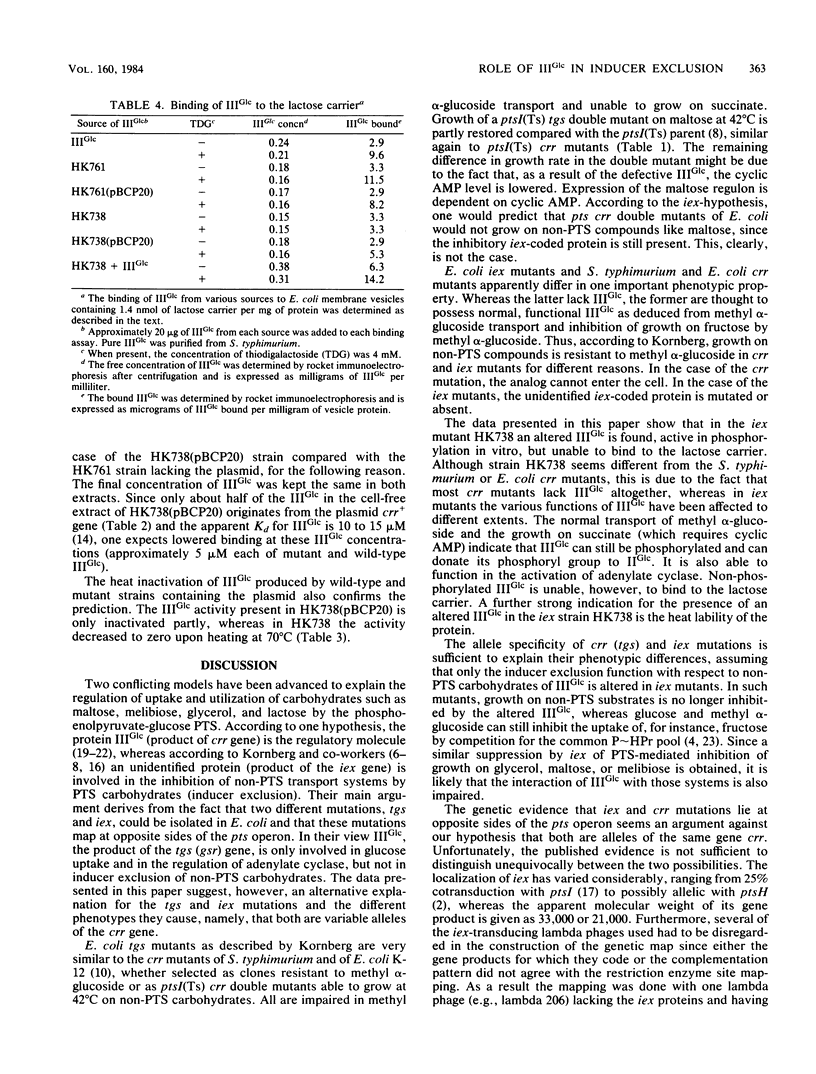
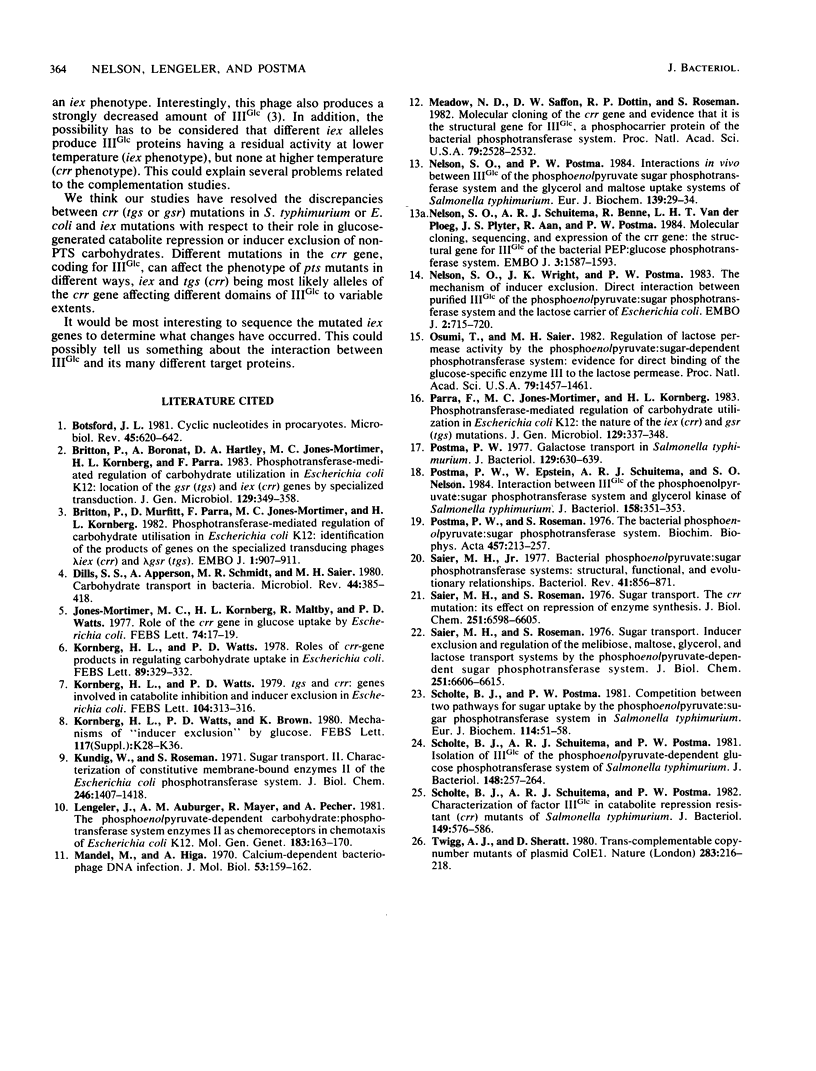
Images in this article
Selected References
These references are in PubMed. This may not be the complete list of references from this article.
- Botsford J. L. Cyclic nucleotides in procaryotes. Microbiol Rev. 1981 Dec;45(4):620–642. doi: 10.1128/mr.45.4.620-642.1981. [DOI] [PMC free article] [PubMed] [Google Scholar]
- Britton P., Boronat A., Hartley D. A., Jones-Mortimer M. C., Kornberg H. L., Parra F. Phosphotransferase-mediated regulation of carbohydrate utilization in Escherichia coli K12: location of the gsr (tgs) and iex (crr) genes by specialized transduction. J Gen Microbiol. 1983 Feb;129(2):349–356. doi: 10.1099/00221287-129-2-349. [DOI] [PubMed] [Google Scholar]
- Britton P., Murfitt D., Parra F., Jones-Mortimer M. C., Kornberg H. L. Phosphotransferase-mediated regulation of carbohydrate utilisation in Escherichia coli K12: identification of the products of genes on the specialised transducing phages lambda iex (crr) and lambda gsr (tgs). EMBO J. 1982;1(8):907–911. doi: 10.1002/j.1460-2075.1982.tb01270.x. [DOI] [PMC free article] [PubMed] [Google Scholar]
- Dills S. S., Apperson A., Schmidt M. R., Saier M. H., Jr Carbohydrate transport in bacteria. Microbiol Rev. 1980 Sep;44(3):385–418. doi: 10.1128/mr.44.3.385-418.1980. [DOI] [PMC free article] [PubMed] [Google Scholar]
- Jones-Mortimer M. C., Kornberg H. L., Maltby R., Watts P. D. Role of the crr-gene in glucose uptake by Escherichia coli. FEBS Lett. 1977 Feb 15;74(1):17–19. doi: 10.1016/0014-5793(77)80742-4. [DOI] [PubMed] [Google Scholar]
- Kornberg H. L., Watts P. D. tgs and crr: Genes involved in catabolite inhibition and inducer exclusion in Escherichia coli. FEBS Lett. 1979 Aug 15;104(2):313–316. doi: 10.1016/0014-5793(79)80841-8. [DOI] [PubMed] [Google Scholar]
- Kornberg H., Watts P. D., Brown K. Mechanisms of 'inducer exclusion' by glucose. FEBS Lett. 1980 Aug 25;117 (Suppl):K28–K36. doi: 10.1016/0014-5793(80)80567-9. [DOI] [PubMed] [Google Scholar]
- Kundig W., Roseman S. Sugar transport. II. Characterization of constitutive membrane-bound enzymes II of the Escherichia coli phosphotransferase system. J Biol Chem. 1971 Mar 10;246(5):1407–1418. [PubMed] [Google Scholar]
- Lengeler J., Auburger A. M., Mayer R., Pecher A. The phosphoenolpyruvate-dependent carbohydrate: phosphotransferase system enzymes II as chemoreceptors in chemotaxis of Escherichia coli K 12. Mol Gen Genet. 1981;183(1):163–170. doi: 10.1007/BF00270156. [DOI] [PubMed] [Google Scholar]
- Mandel M., Higa A. Calcium-dependent bacteriophage DNA infection. J Mol Biol. 1970 Oct 14;53(1):159–162. doi: 10.1016/0022-2836(70)90051-3. [DOI] [PubMed] [Google Scholar]
- Meadow N. D., Saffen D. W., Dottin R. P., Roseman S. Molecular cloning of the crr gene and evidence that it is the structural gene for IIIGlc, a phosphocarrier protein of the bacterial phosphotransferase system. Proc Natl Acad Sci U S A. 1982 Apr;79(8):2528–2532. doi: 10.1073/pnas.79.8.2528. [DOI] [PMC free article] [PubMed] [Google Scholar]
- Nelson S. O., Postma P. W. Interactions in vivo between IIIGlc of the phosphoenolpyruvate:sugar phosphotransferase system and the glycerol and maltose uptake systems of Salmonella typhimurium. Eur J Biochem. 1984 Feb 15;139(1):29–34. doi: 10.1111/j.1432-1033.1984.tb07971.x. [DOI] [PubMed] [Google Scholar]
- Nelson S. O., Schuitema A. R., Benne R., van der Ploeg L. H., Plijter J. S., Aan F., Postma P. W. Molecular cloning, sequencing, and expression of the crr gene: the structural gene for IIIGlc of the bacterial PEP:glucose phosphotransferase system. EMBO J. 1984 Jul;3(7):1587–1593. doi: 10.1002/j.1460-2075.1984.tb02015.x. [DOI] [PMC free article] [PubMed] [Google Scholar]
- Nelson S. O., Wright J. K., Postma P. W. The mechanism of inducer exclusion. Direct interaction between purified III of the phosphoenolpyruvate:sugar phosphotransferase system and the lactose carrier of Escherichia coli. EMBO J. 1983;2(5):715–720. doi: 10.1002/j.1460-2075.1983.tb01490.x. [DOI] [PMC free article] [PubMed] [Google Scholar]
- Osumi T., Saier M. H., Jr Regulation of lactose permease activity by the phosphoenolpyruvate:sugar phosphotransferase system: evidence for direct binding of the glucose-specific enzyme III to the lactose permease. Proc Natl Acad Sci U S A. 1982 Mar;79(5):1457–1461. doi: 10.1073/pnas.79.5.1457. [DOI] [PMC free article] [PubMed] [Google Scholar]
- Parra F., Jones-Mortimer M. C., Kornberg H. L. Phosphotransferase-mediated regulation of carbohydrate utilization in Escherichia coli K12: the nature of the iex (crr) and gsr (tgs) mutations. J Gen Microbiol. 1983 Feb;129(2):337–348. doi: 10.1099/00221287-129-2-337. [DOI] [PubMed] [Google Scholar]
- Postma P. W., Epstein W., Schuitema A. R., Nelson S. O. Interaction between IIIGlc of the phosphoenolpyruvate:sugar phosphotransferase system and glycerol kinase of Salmonella typhimurium. J Bacteriol. 1984 Apr;158(1):351–353. doi: 10.1128/jb.158.1.351-353.1984. [DOI] [PMC free article] [PubMed] [Google Scholar]
- Postma P. W. Galactose transport in Salmonella typhimurium. J Bacteriol. 1977 Feb;129(2):630–639. doi: 10.1128/jb.129.2.630-639.1977. [DOI] [PMC free article] [PubMed] [Google Scholar]
- Postma P. W., Roseman S. The bacterial phosphoenolpyruvate: sugar phosphotransferase system. Biochim Biophys Acta. 1976 Dec 14;457(3-4):213–257. doi: 10.1016/0304-4157(76)90001-0. [DOI] [PubMed] [Google Scholar]
- Saier M. H., Jr Bacterial phosphoenolpyruvate: sugar phosphotransferase systems: structural, functional, and evolutionary interrelationships. Bacteriol Rev. 1977 Dec;41(4):856–871. doi: 10.1128/br.41.4.856-871.1977. [DOI] [PMC free article] [PubMed] [Google Scholar]
- Saier M. H., Jr, Roseman S. Sugar transport. 2nducer exclusion and regulation of the melibiose, maltose, glycerol, and lactose transport systems by the phosphoenolpyruvate:sugar phosphotransferase system. J Biol Chem. 1976 Nov 10;251(21):6606–6615. [PubMed] [Google Scholar]
- Saier M. H., Jr, Roseman S. Sugar transport. The crr mutation: its effect on repression of enzyme synthesis. J Biol Chem. 1976 Nov 10;251(21):6598–6605. [PubMed] [Google Scholar]
- Scholte B. J., Postma P. W. Competition between two pathways for sugar uptake by the phosphoenolpyruvate-dependent sugar phosphotransferase system in Salmonella typhimurium. Eur J Biochem. 1981;114(1):51–58. doi: 10.1111/j.1432-1033.1981.tb06171.x. [DOI] [PubMed] [Google Scholar]
- Scholte B. J., Schuitema A. R., Postma P. W. Characterization of factor IIIGLc in catabolite repression-resistant (crr) mutants of Salmonella typhimurium. J Bacteriol. 1982 Feb;149(2):576–586. doi: 10.1128/jb.149.2.576-586.1982. [DOI] [PMC free article] [PubMed] [Google Scholar]
- Scholte B. J., Schuitema A. R., Postma P. W. Isolation of IIIGlc of the phosphoenolpyruvate-dependent glucose phosphotransferase system of Salmonella typhimurium. J Bacteriol. 1981 Oct;148(1):257–264. doi: 10.1128/jb.148.1.257-264.1981. [DOI] [PMC free article] [PubMed] [Google Scholar]
- Twigg A. J., Sherratt D. Trans-complementable copy-number mutants of plasmid ColE1. Nature. 1980 Jan 10;283(5743):216–218. doi: 10.1038/283216a0. [DOI] [PubMed] [Google Scholar]