Abstract
The inducible nonenzymatic chloramphenicol resistance (Cmr) determinant of the IncP plasmid R26 was cloned on a 1,900-base-pair restriction endonuclease HindIII fragment. Transposon Tn5 mutagenesis revealed that at least 1,400 base pairs is required for expression of Cmr. There was no increase in the level of Cmr when the copy number of the determinant was raised by cloning in pBR322 or pUB5572. Expression of Cmr by cells carrying a lower-copy-number pUB5572cml+ plasmid was inducible and thus indistinguishable from those with R26 itself. However, pBR322cml+-carrying cells expressed Cmr constitutively, possibly due to the activity of vector promoters or an elevated copy number. Transcriptional and translational cml-lac fusions were constructed. The operon (transcriptional) cml-lac fusion carried by the low-copy-number plasmid pUB5572 caused a low level of constitutive beta-galactosidase activity, which could not be elevated by induction with chloramphenicol and was not affected by a coresident R26cml+ element. In contrast, the gene (translational) cml-lac fusion expressed low-level beta-galactosidase activity, which was elevated fivefold by prior exposure to chloramphenicol. We conclude that the regulation of Cmr occurs posttranscriptionally.
Full text
PDF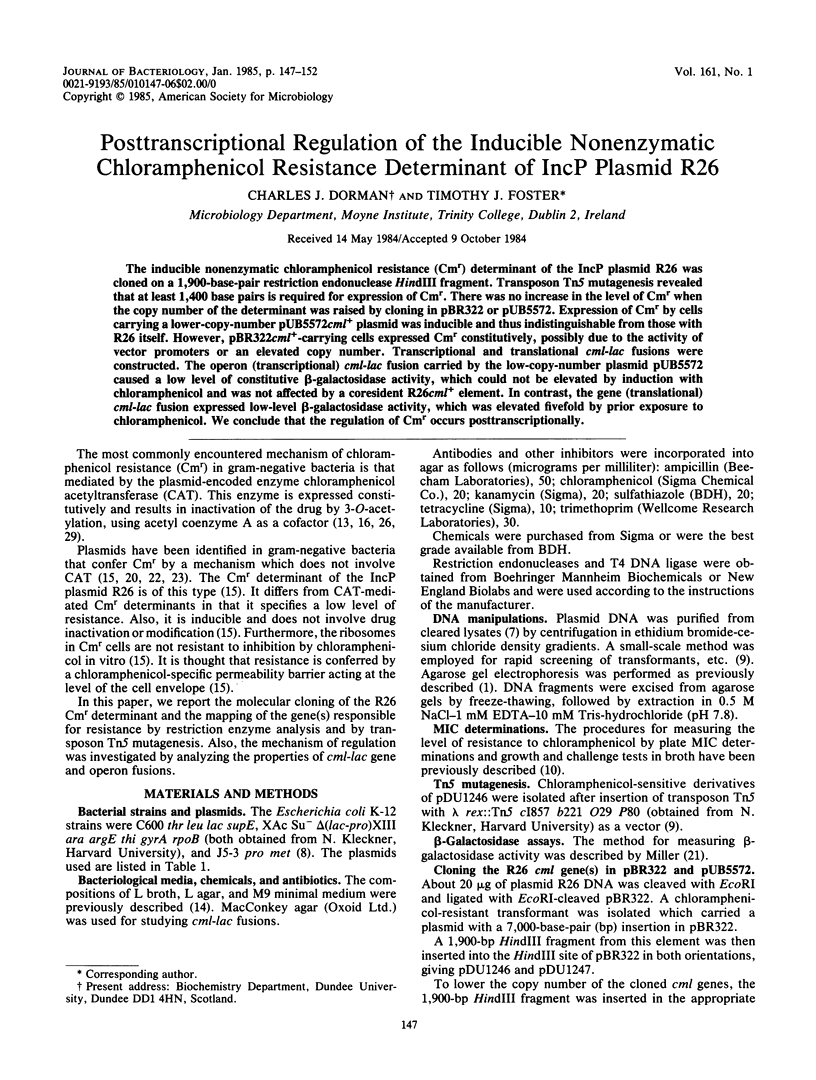
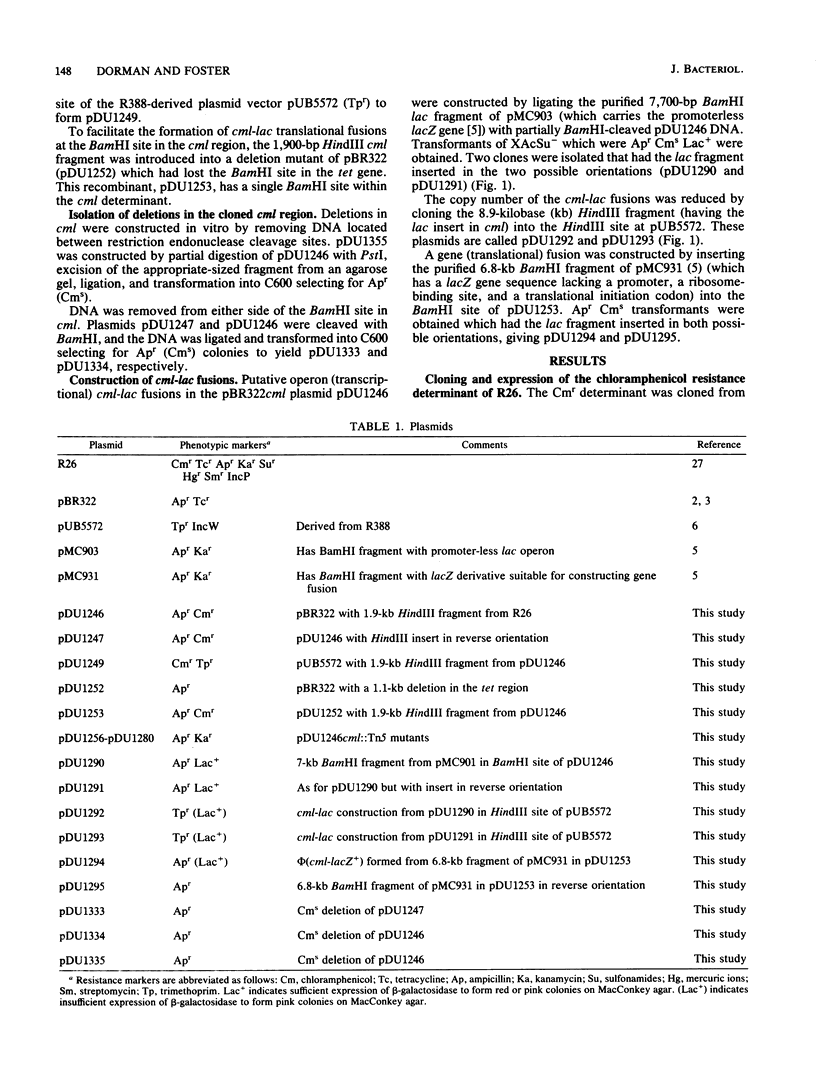
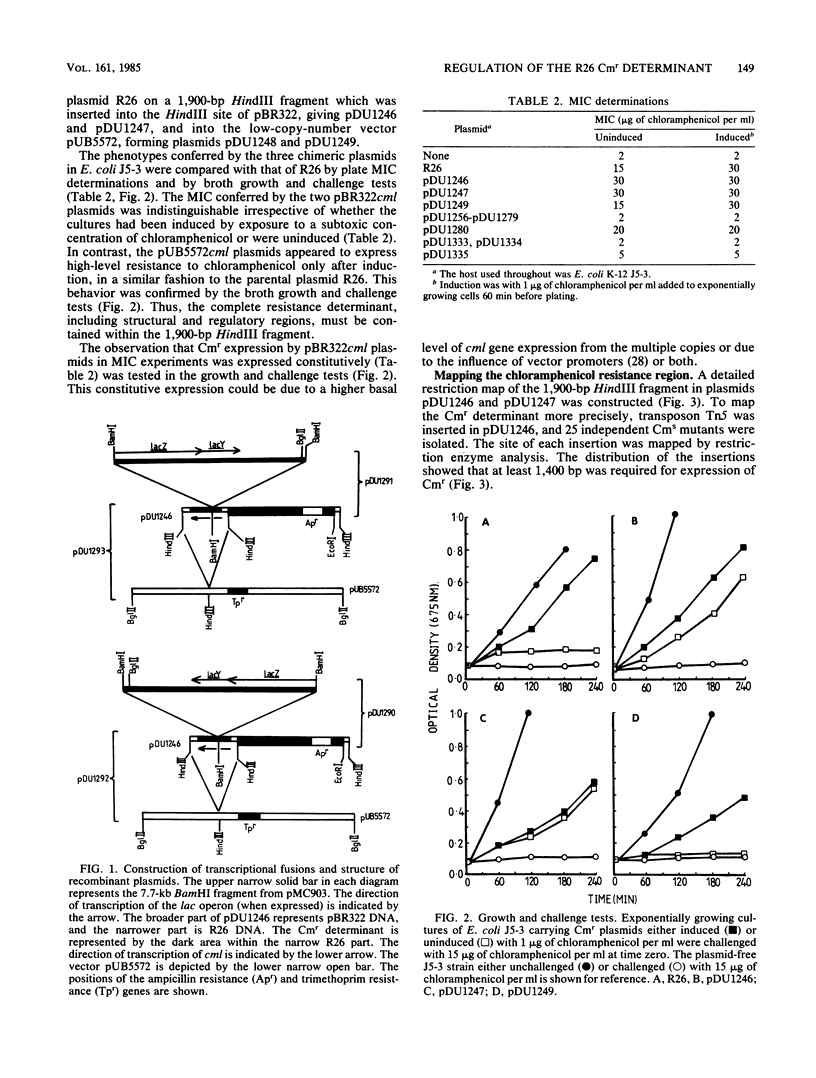
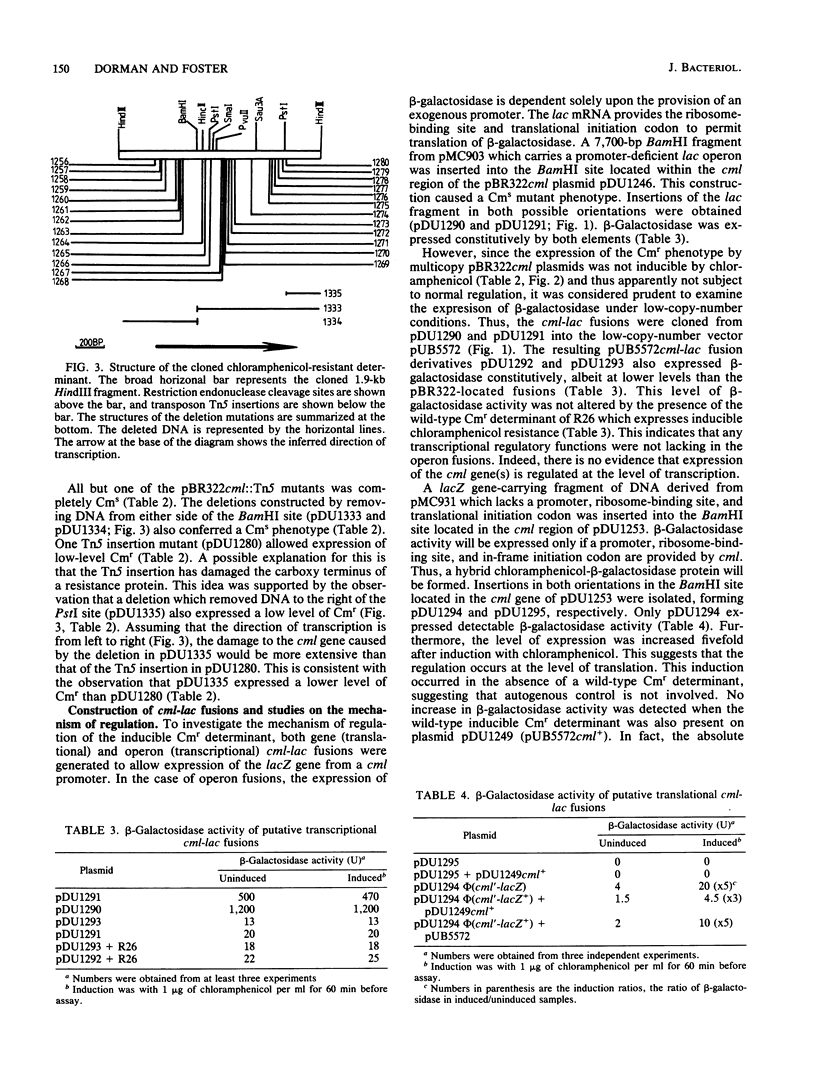
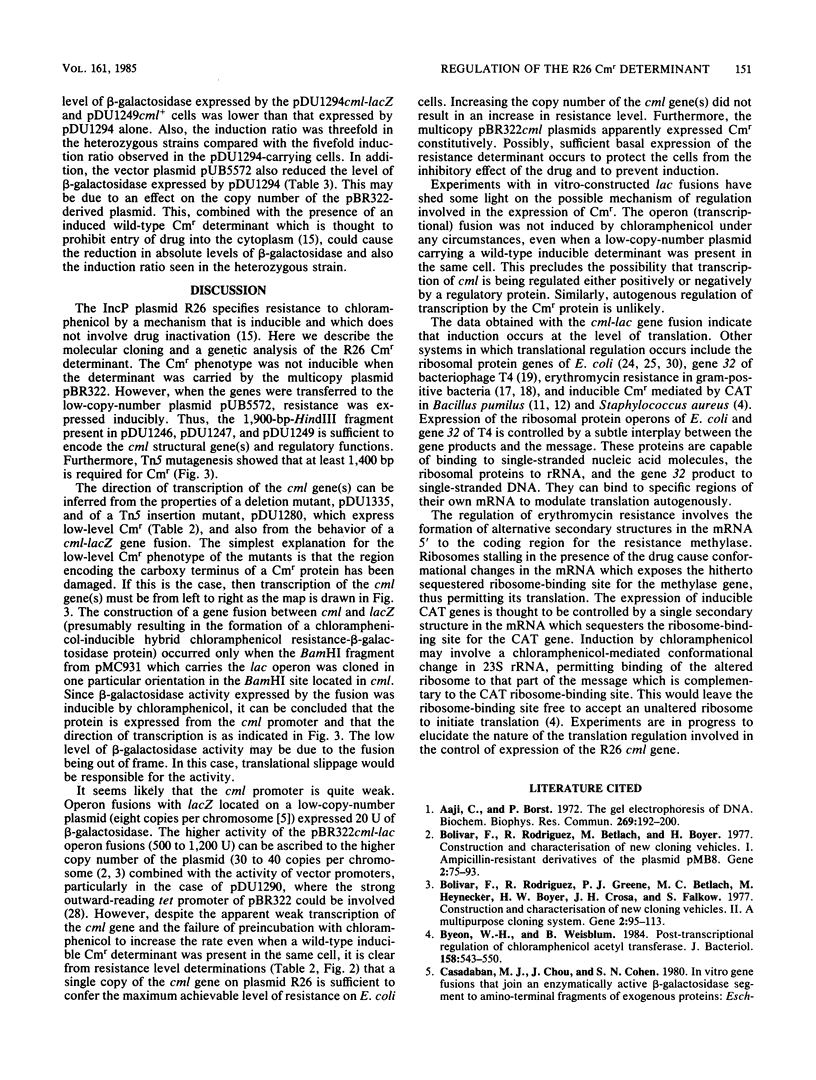
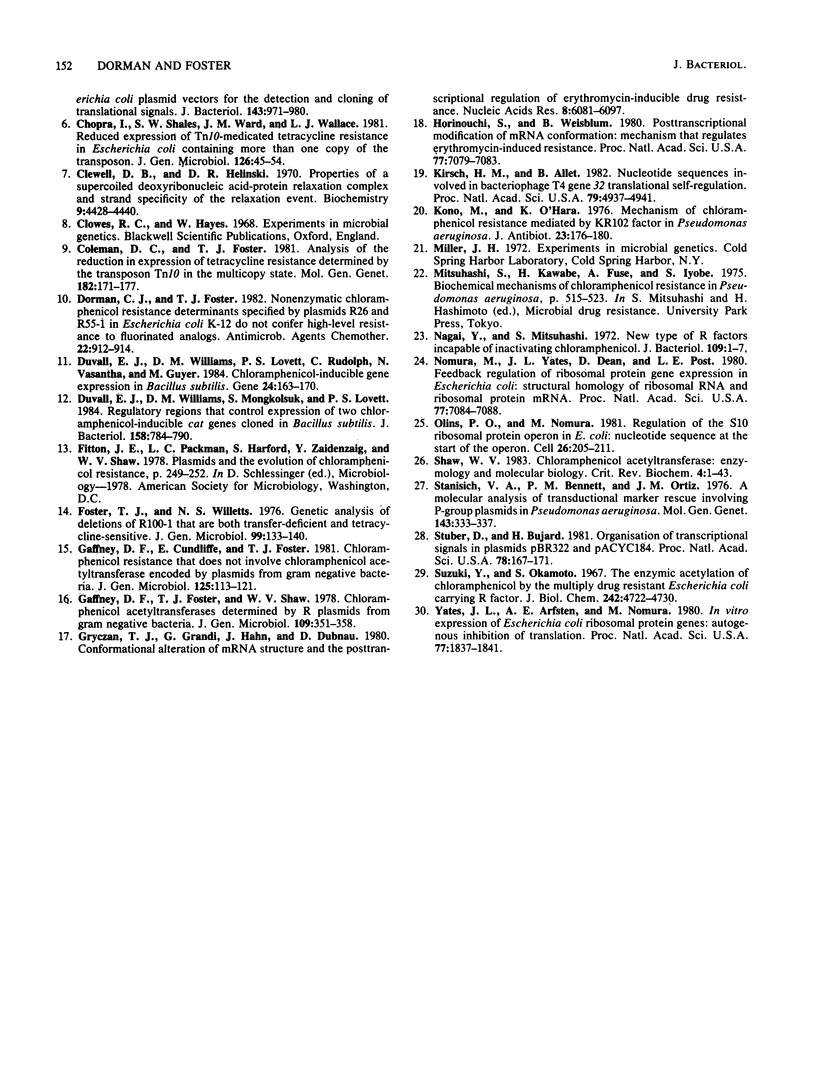
Selected References
These references are in PubMed. This may not be the complete list of references from this article.
- Aaij C., Borst P. The gel electrophoresis of DNA. Biochim Biophys Acta. 1972 May 10;269(2):192–200. doi: 10.1016/0005-2787(72)90426-1. [DOI] [PubMed] [Google Scholar]
- Bolivar F., Rodriguez R. L., Betlach M. C., Boyer H. W. Construction and characterization of new cloning vehicles. I. Ampicillin-resistant derivatives of the plasmid pMB9. Gene. 1977;2(2):75–93. doi: 10.1016/0378-1119(77)90074-9. [DOI] [PubMed] [Google Scholar]
- Bolivar F., Rodriguez R. L., Greene P. J., Betlach M. C., Heyneker H. L., Boyer H. W., Crosa J. H., Falkow S. Construction and characterization of new cloning vehicles. II. A multipurpose cloning system. Gene. 1977;2(2):95–113. [PubMed] [Google Scholar]
- Byeon W. H., Weisblum B. Post-transcriptional regulation of chloramphenicol acetyl transferase. J Bacteriol. 1984 May;158(2):543–550. doi: 10.1128/jb.158.2.543-550.1984. [DOI] [PMC free article] [PubMed] [Google Scholar]
- Chopra I., Shales S. W., Ward J. M., Wallace L. J. Reduced expression of Tn 10-mediated tetracycline resistance in Escherichia coli containing more than one copy of the transposon. J Gen Microbiol. 1981 Sep;126(1):45–54. doi: 10.1099/00221287-126-1-45. [DOI] [PubMed] [Google Scholar]
- Clewell D. B., Helinski D. R. Properties of a supercoiled deoxyribonucleic acid-protein relaxation complex and strand specificity of the relaxation event. Biochemistry. 1970 Oct 27;9(22):4428–4440. doi: 10.1021/bi00824a026. [DOI] [PubMed] [Google Scholar]
- Coleman D. C., Foster T. J. Analysis of the reduction in expression of tetracycline resistance determined by transposon Tn10 in the multicopy state. Mol Gen Genet. 1981;182(1):171–177. doi: 10.1007/BF00422786. [DOI] [PubMed] [Google Scholar]
- Dorman C. J., Foster T. J. Nonenzymatic chloramphenicol resistance determinants specified by plasmids R26 and R55-1 in Escherichia coli K-12 do not confer high-level resistance to fluorinated analogs. Antimicrob Agents Chemother. 1982 Nov;22(5):912–914. doi: 10.1128/aac.22.5.912. [DOI] [PMC free article] [PubMed] [Google Scholar]
- Duvall E. J., Williams D. M., Mongkolsuk S., Lovett P. S. Regulatory regions that control expression of two chloramphenicol-inducible cat genes cloned in Bacillus subtilis. J Bacteriol. 1984 Jun;158(3):784–790. doi: 10.1128/jb.158.3.784-790.1984. [DOI] [PMC free article] [PubMed] [Google Scholar]
- Foster T. J., Willetts N. S. Genetic analysis of deletions of R100-1 that are both transfer-deficient and tetracycline-sensitive. J Gen Microbiol. 1976 Mar;93(1):133–140. doi: 10.1099/00221287-93-1-133. [DOI] [PubMed] [Google Scholar]
- Gaffney D. F., Cundliffe E., Foster T. J. Chloramphenicol resistance that does not involve chloramphenicol acetyltransferase encoded by plasmids from gram-negative bacteria. J Gen Microbiol. 1981 Jul;125(1):113–121. doi: 10.1099/00221287-125-1-113. [DOI] [PubMed] [Google Scholar]
- Gryczan T. J., Grandi G., Hahn J., Grandi R., Dubnau D. Conformational alteration of mRNA structure and the posttranscriptional regulation of erythromycin-induced drug resistance. Nucleic Acids Res. 1980 Dec 20;8(24):6081–6097. doi: 10.1093/nar/8.24.6081. [DOI] [PMC free article] [PubMed] [Google Scholar]
- Horinouchi S., Weisblum B. Posttranscriptional modification of mRNA conformation: mechanism that regulates erythromycin-induced resistance. Proc Natl Acad Sci U S A. 1980 Dec;77(12):7079–7083. doi: 10.1073/pnas.77.12.7079. [DOI] [PMC free article] [PubMed] [Google Scholar]
- Kono M., O'Hara K. Mechanism of chloramphenicol-resistance mediated by kR102 factor in Pseudomonas aeruginosa. J Antibiot (Tokyo) 1976 Feb;29(2):176–180. doi: 10.7164/antibiotics.29.176. [DOI] [PubMed] [Google Scholar]
- Krisch H. M., Allet B. Nucleotide sequences involved in bacteriophage T4 gene 32 translational self-regulation. Proc Natl Acad Sci U S A. 1982 Aug;79(16):4937–4941. doi: 10.1073/pnas.79.16.4937. [DOI] [PMC free article] [PubMed] [Google Scholar]
- Nomura M., Yates J. L., Dean D., Post L. E. Feedback regulation of ribosomal protein gene expression in Escherichia coli: structural homology of ribosomal RNA and ribosomal protein MRNA. Proc Natl Acad Sci U S A. 1980 Dec;77(12):7084–7088. doi: 10.1073/pnas.77.12.7084. [DOI] [PMC free article] [PubMed] [Google Scholar]
- Olins P. O., Nomura M. Regulation of the S10 ribosomal protein operon in E. coli: nucleotide sequence at the start of the operon. Cell. 1981 Oct;26(2 Pt 2):205–211. doi: 10.1016/0092-8674(81)90303-2. [DOI] [PubMed] [Google Scholar]
- Shaw W. V. Chloramphenicol acetyltransferase: enzymology and molecular biology. CRC Crit Rev Biochem. 1983;14(1):1–46. doi: 10.3109/10409238309102789. [DOI] [PubMed] [Google Scholar]
- Stanisich V. A., Bennett P. M., Oritz J. M. A molecular analysis of transductional marker rescue involving P-group plasmids in Pseudomonas aeruginosa. Mol Gen Genet. 1976 Feb 2;143(3):333–337. doi: 10.1007/BF00269412. [DOI] [PubMed] [Google Scholar]
- Stüber D., Bujard H. Organization of transcriptional signals in plasmids pBR322 and pACYC184. Proc Natl Acad Sci U S A. 1981 Jan;78(1):167–171. doi: 10.1073/pnas.78.1.167. [DOI] [PMC free article] [PubMed] [Google Scholar]
- Suzuki Y., Okamoto S. The enzymatic acetylation of chloramphenicol by the multiple drug-resistant Escherichia coli carrying R factor. J Biol Chem. 1967 Oct 25;242(20):4722–4730. [PubMed] [Google Scholar]
- Yates J. L., Arfsten A. E., Nomura M. In vitro expression of Escherichia coli ribosomal protein genes: autogenous inhibition of translation. Proc Natl Acad Sci U S A. 1980 Apr;77(4):1837–1841. doi: 10.1073/pnas.77.4.1837. [DOI] [PMC free article] [PubMed] [Google Scholar]