Abstract
In vivo rates of glucose uptake and acid production by oral streptococci grown in glucose- or nitrogen-limited continuous culture and batch culture were compared with the glucose phosphorylation activities of harvested, decryptified cells. The strains examined contained significant phosphoenolpyruvate-phosphotransferase system (PTS) activity, measured by a glucose 6-phosphate (G6P) dehydrogenase-linked assay procedure, but this activity was insufficient to account for the in vivo glucose uptake rates. However, ATP was a superior phosphoryl donor to phosphoenolpyruvate, and unlike the PTS, phosphoryl transfer with ATP was insensitive to bacteriostatic concentrations of chlorhexidine, suggesting glucokinase-mediated G6P formation. Again, G6P formation from the PTS and glucokinase reactions was not commensurate with some of the glucose uptake rates observed, implying that other phosphorylation reactions must be occurring. Two novel reactions involving carbamyl phosphate and acetyl phosphate were identified in some of the strains. No G6P formation was detected with these potential phosphoryl donors, but in the presence of phosphoglucomutase, glucose 1-phosphate (G1P) formation was evident, which was insensitive to chlorhexidine. G1P is a precursor of glycogen, and good correlation was obtained between G1P formation activity and endogenous metabolism of washed cells measured either as a rate of acid production at a constant pH 7 or as a decrease in pH with time in the absence of titrant. A "league table" of abilities to synthesize G1P and produce acid from endogenous metabolism was compiled for oral streptococci grown in batch culture. This indicated that Streptococcus mutans Ingbritt and Streptococcus sanguis Challis were unable to form G1P or produce much acid endogenously, whereas increasing activities were obtained with Streptococcus salivarius, Streptococcus sanguis, and Streptococcus mitis. In particular, S. mitis had the highest G1P formation activities and was able to decrease the pH to less than 5 in 15 min by endogenous metabolism alone. The data are consistent with the intracellular accumulation of free glucose driven by proton motive force when PTS activities are low and the subsequent phosphorylation to either G6P for metabolism via glycolysis or G1P for glycogen biosynthesis. The accumulation of acetyl phosphate during glucose-limited growth and the availability of arginine for catabolism to carbamyl phosphate provide an explanation as to why some glucose-limited oral streptococci continue to synthesize glycogen under these conditions, which might prevail in plaque.
Full text
PDF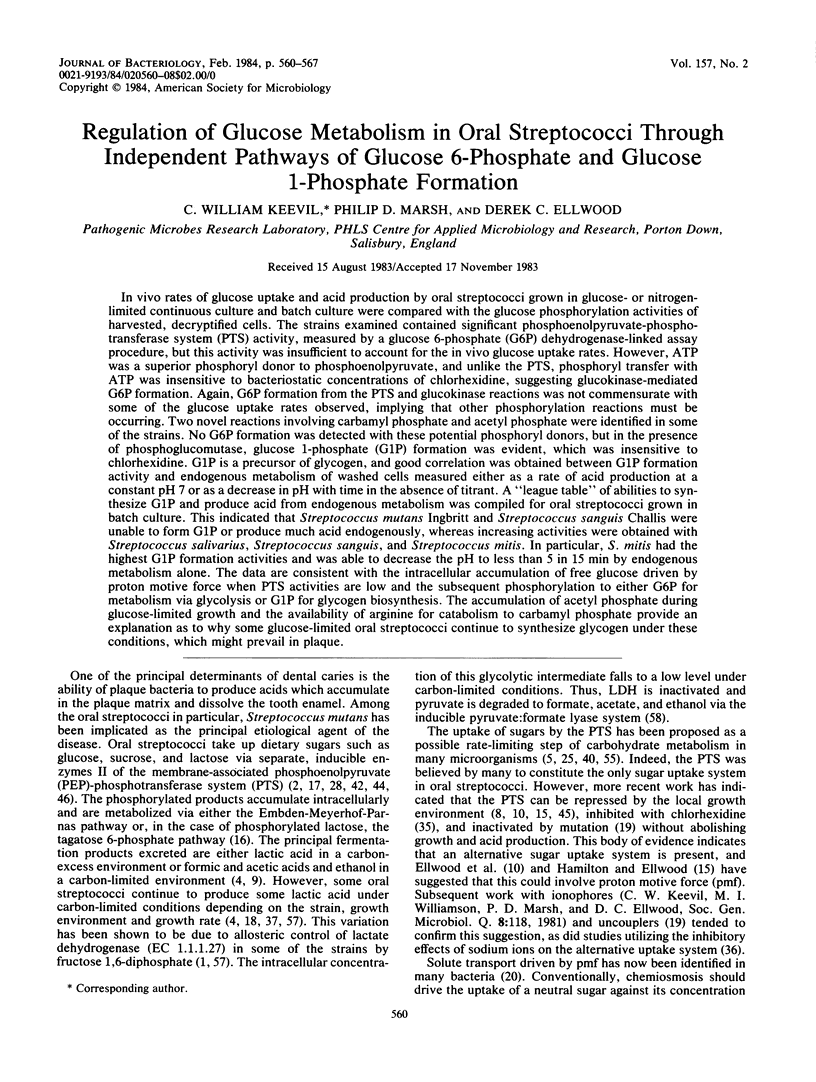
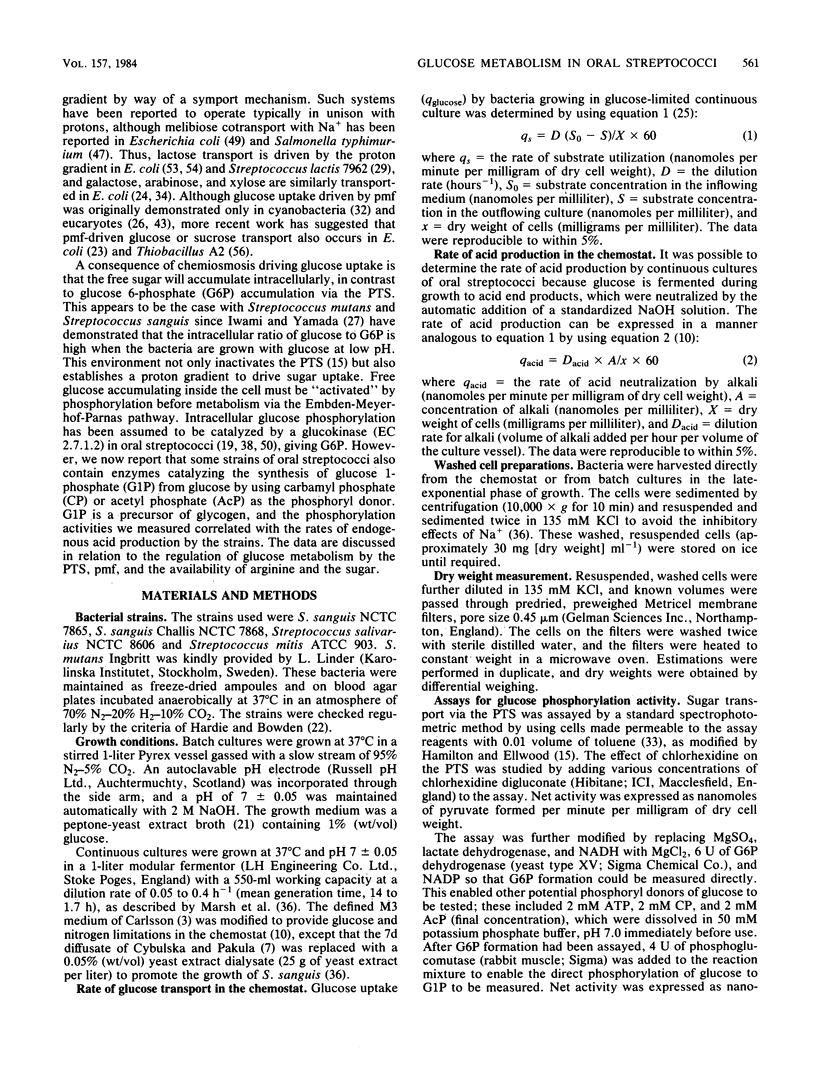
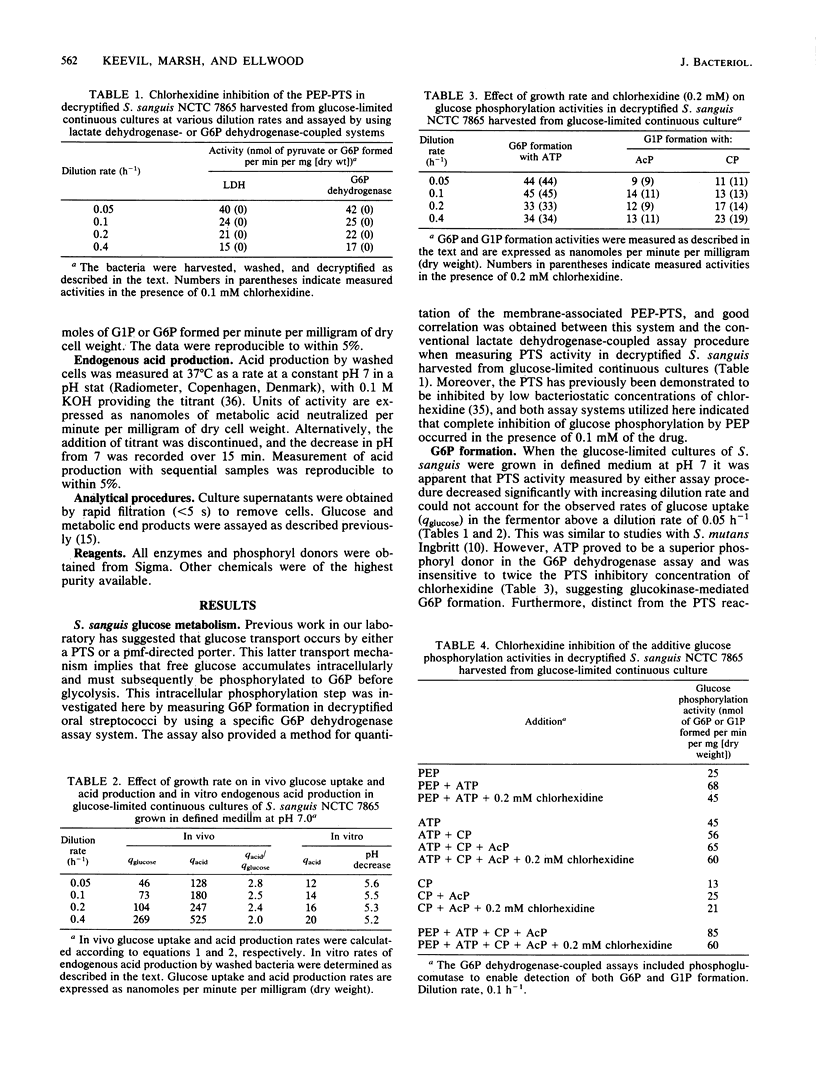
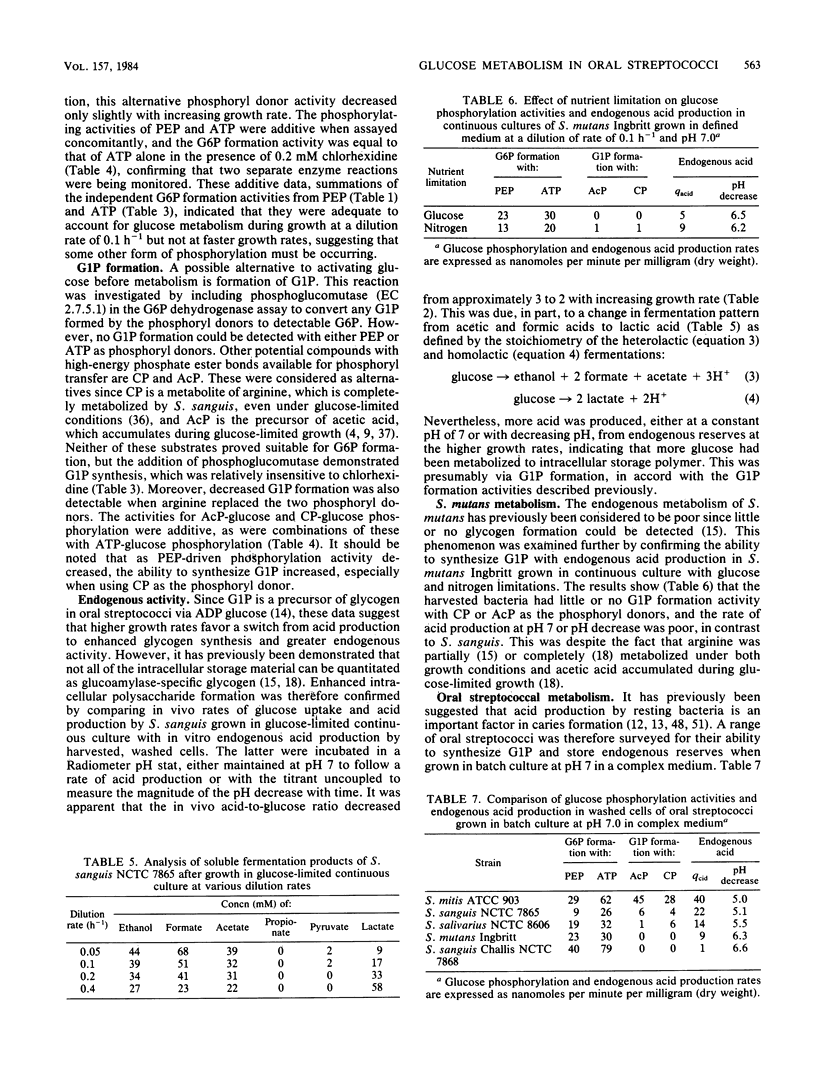
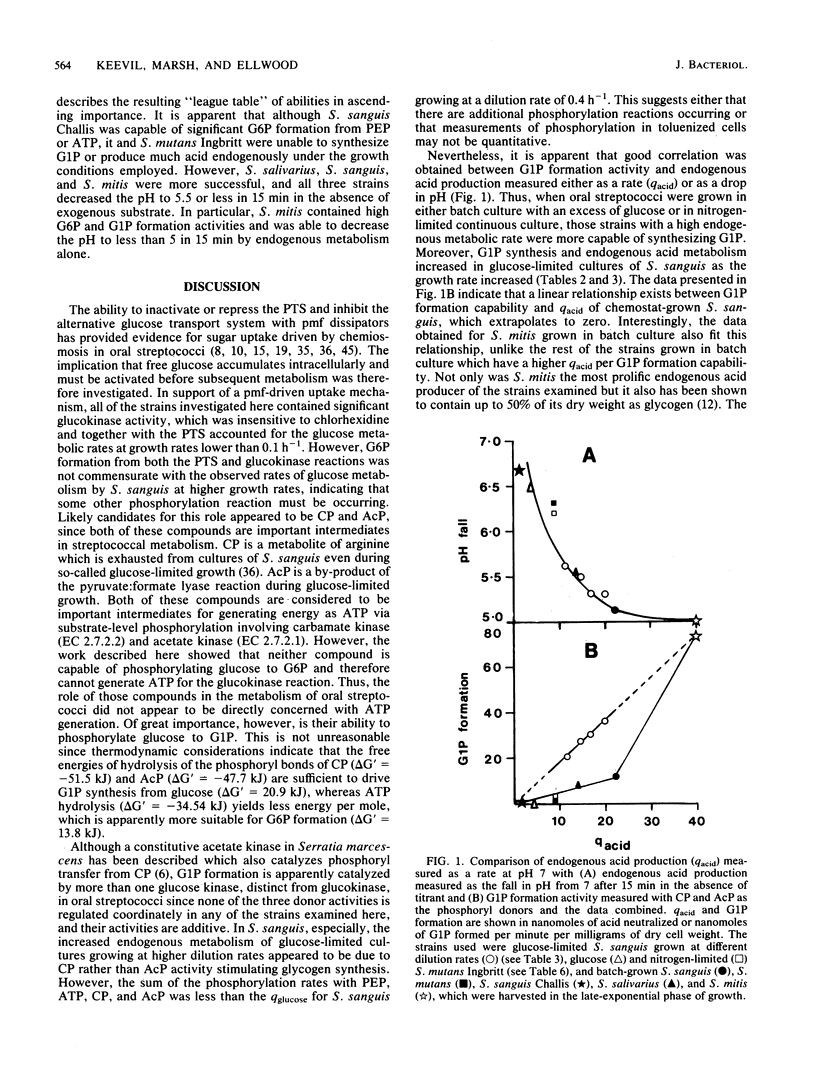
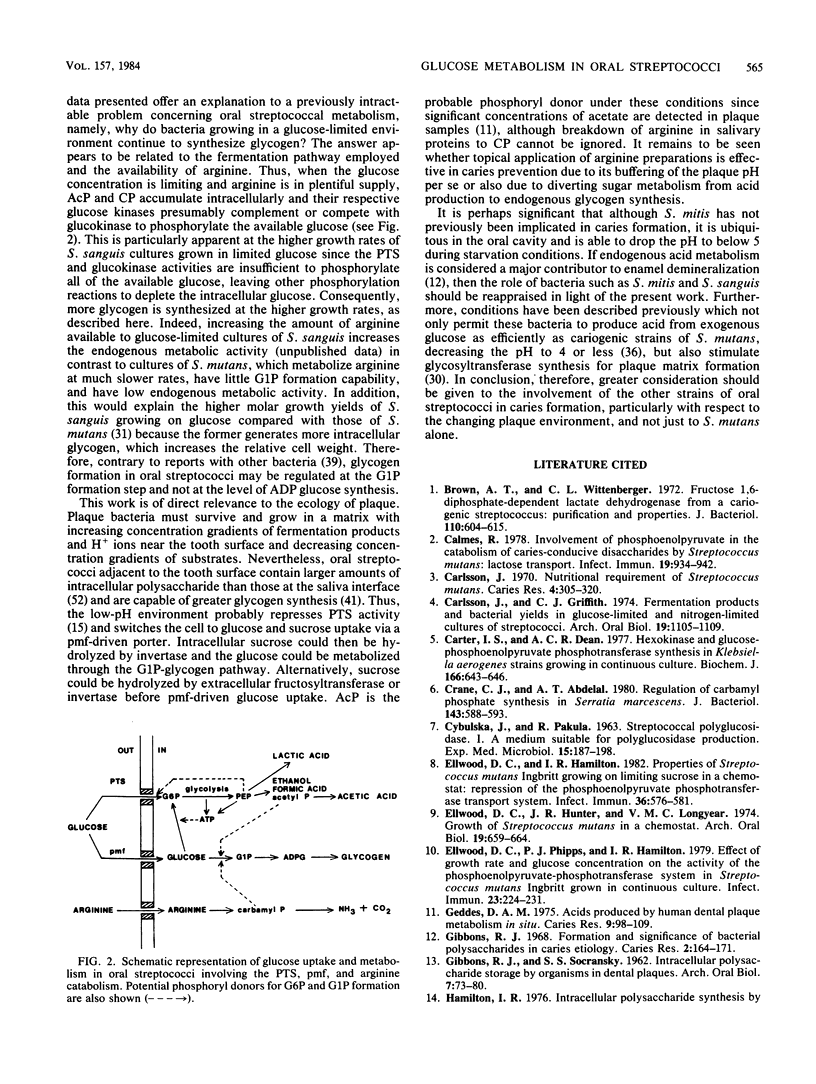
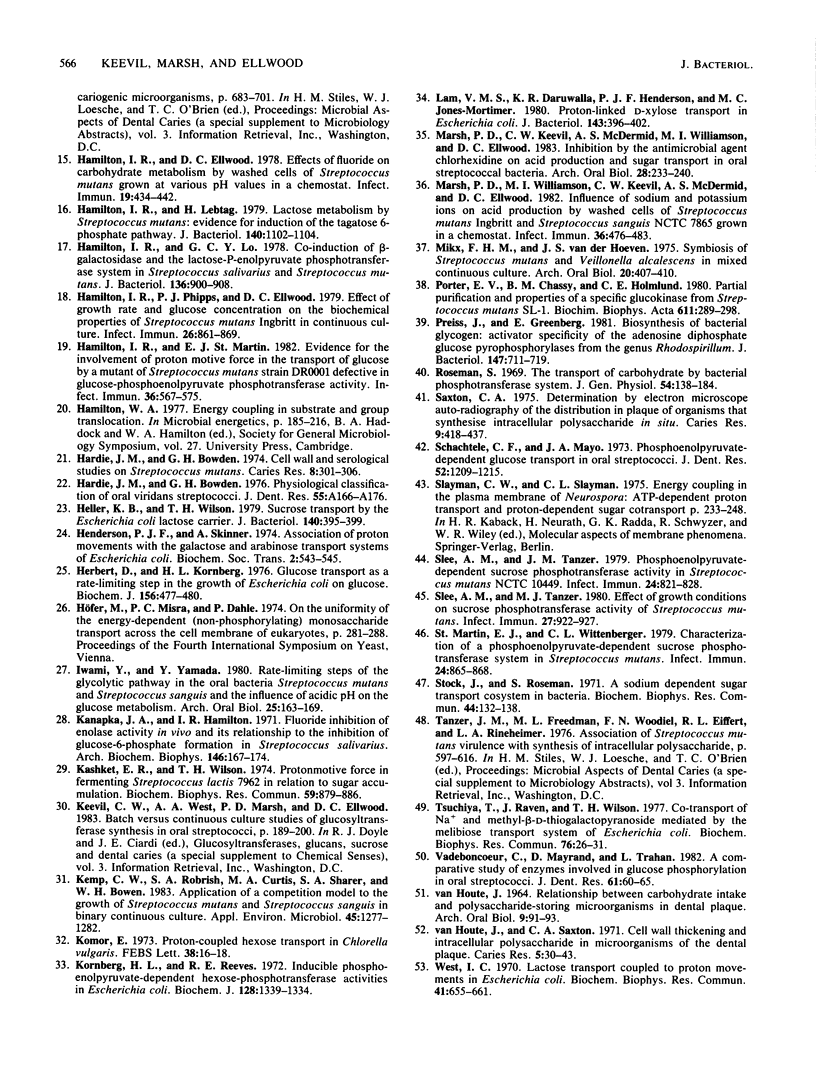
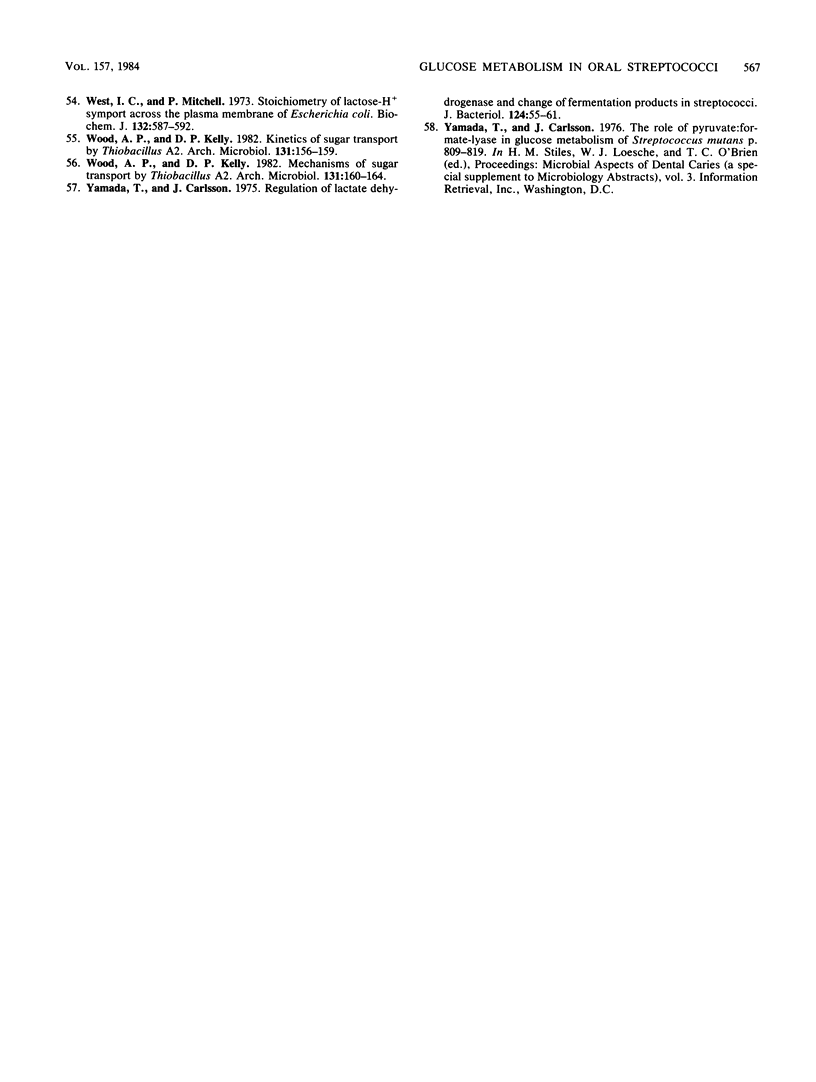
Selected References
These references are in PubMed. This may not be the complete list of references from this article.
- Brown A. T., Wittenberger C. L. Fructose-1,6-diphosphate-dependent lactate dehydrogenase from a cariogenic streptococcus: purification and regulatory properties. J Bacteriol. 1972 May;110(2):604–615. doi: 10.1128/jb.110.2.604-615.1972. [DOI] [PMC free article] [PubMed] [Google Scholar]
- Calmes R. Involvement of phosphoenolpyruvate in the catabolism of caries-conducive disaccharides by Streptococcus mutans: lactose transport. Infect Immun. 1978 Mar;19(3):934–942. doi: 10.1128/iai.19.3.934-942.1978. [DOI] [PMC free article] [PubMed] [Google Scholar]
- Carlsson J., Griffith C. J. Fermentation products and bacterial yields in glucose-limited and nitrogen-limited cultures of streptococci. Arch Oral Biol. 1974 Dec;19(12):1105–1109. doi: 10.1016/0003-9969(74)90238-6. [DOI] [PubMed] [Google Scholar]
- Carlsson J. Nutritional requirements of Streptococcus mutans. Caries Res. 1970;4(4):305–320. doi: 10.1159/000259653. [DOI] [PubMed] [Google Scholar]
- Carter I. S., Dean A. C. Hexokinase and glucose-phosphoenolpyruvate phosphotransferase synthesis in Klebsiella aerogenes strains growing in continuous culture. Biochem J. 1977 Sep 15;166(3):643–646. doi: 10.1042/bj1660643. [DOI] [PMC free article] [PubMed] [Google Scholar]
- Crane C. J., Abdelal A. T. Regulation of carbamylphosphate synthesis in Serratia marcescens. J Bacteriol. 1980 Aug;143(2):588–593. doi: 10.1128/jb.143.2.588-593.1980. [DOI] [PMC free article] [PubMed] [Google Scholar]
- Ellwood D. C., Hamilton I. R. Properties of Streptococcus mutans Ingbritt growing on limiting sucrose in a chemostat: repression of the phosphoenolpyruvate phosphotransferase transport system. Infect Immun. 1982 May;36(2):576–581. doi: 10.1128/iai.36.2.576-581.1982. [DOI] [PMC free article] [PubMed] [Google Scholar]
- Ellwood D. C., Hunter J. R., Longyear V. M. Growth of Streptococcus mutans in a chemostat. Arch Oral Biol. 1974 Aug;19(8):659–664. doi: 10.1016/0003-9969(74)90134-4. [DOI] [PubMed] [Google Scholar]
- Ellwood D. C., Phipps P. J., Hamilton I. R. Effect of growth rate and glucose concentration on the activity of the phosphoenolpyruvate phosphotransferase system in Streptococcus mutans Ingbritt grown in continuous culture. Infect Immun. 1979 Feb;23(2):224–231. doi: 10.1128/iai.23.2.224-231.1979. [DOI] [PMC free article] [PubMed] [Google Scholar]
- GIBBONS R. J., SOCRANSKY S. S. Intracellular polysaccharide storage by organisms in dental plaques. Its relation to dental caries and microbial ecology of the oral cavity. Arch Oral Biol. 1962 Jan-Feb;7:73–79. doi: 10.1016/0003-9969(62)90050-x. [DOI] [PubMed] [Google Scholar]
- Geddes D. A. Acids produced by human dental plaque metabolism in situ. Caries Res. 1975;9(2):98–109. doi: 10.1159/000260149. [DOI] [PubMed] [Google Scholar]
- Gibbons R. J. Formation and significance of bacterial polysaccharides in caries etiology. Caries Res. 1968;2(2):164–171. doi: 10.1159/000259554. [DOI] [PubMed] [Google Scholar]
- Hamilton I. R., Ellwood D. C. Effects of fluoride on carbohydrate metabolism by washed cells of Streptococcus mutans grown at various pH values in a chemostat. Infect Immun. 1978 Feb;19(2):434–442. doi: 10.1128/iai.19.2.434-442.1978. [DOI] [PMC free article] [PubMed] [Google Scholar]
- Hamilton I. R., Lebtag H. Lactose metabolism by Streptococcus mutans: evidence for induction of the tagatose 6-phosphate pathway. J Bacteriol. 1979 Dec;140(3):1102–1104. doi: 10.1128/jb.140.3.1102-1104.1979. [DOI] [PMC free article] [PubMed] [Google Scholar]
- Hamilton I. R., Lo G. C. Co-induction of beta-galactosidase and the lactose-P-enolpyruvate phosphotransferase system in Streptococcus salivarius and Streptococcus mutans. J Bacteriol. 1978 Dec;136(3):900–908. doi: 10.1128/jb.136.3.900-908.1978. [DOI] [PMC free article] [PubMed] [Google Scholar]
- Hamilton I. R., Phipps P. J., Ellwood D. C. Effect of growth rate and glucose concentration on the biochemical properties of Streptococcus mutans Ingbritt in continuous culture. Infect Immun. 1979 Dec;26(3):861–869. doi: 10.1128/iai.26.3.861-869.1979. [DOI] [PMC free article] [PubMed] [Google Scholar]
- Hamilton I. R., St Martin E. J. Evidence for the involvement of proton motive force in the transport of glucose by a mutant of Streptococcus mutans strain DR0001 defective in glucose-phosphoenolpyruvate phosphotransferase activity. Infect Immun. 1982 May;36(2):567–575. doi: 10.1128/iai.36.2.567-575.1982. [DOI] [PMC free article] [PubMed] [Google Scholar]
- Hardie J. M., Bowden G. H. Cell wall and serological studies on Streptococcus mutans. Caries Res. 1974;8(4):301–316. doi: 10.1159/000260120. [DOI] [PubMed] [Google Scholar]
- Hardie J. M., Bowden G. H. Physiological classification of oral viridans streptococci. J Dent Res. 1976 Jan;55:A166–A176. doi: 10.1177/002203457605500108011. [DOI] [PubMed] [Google Scholar]
- Heller K. B., Wilson T. H. Sucrose transport by the Escherichia coli lactose carrier. J Bacteriol. 1979 Nov;140(2):395–399. doi: 10.1128/jb.140.2.395-399.1979. [DOI] [PMC free article] [PubMed] [Google Scholar]
- Herbert D., Kornberg H. L. Glucose transport as rate-limiting step in the growth of Escherichia coli on glucose. Biochem J. 1976 May 15;156(2):477–480. doi: 10.1042/bj1560477. [DOI] [PMC free article] [PubMed] [Google Scholar]
- Iwami Y., Yamada T. Rate-limiting steps of the glycolytic pathway in the oral bacteria Streptococcus mutans and Streptococcus sanguis and the influence of acidic pH on the glucose metabolism. Arch Oral Biol. 1980;25(3):163–169. doi: 10.1016/0003-9969(80)90015-1. [DOI] [PubMed] [Google Scholar]
- Kanapka J. A., Hamilton I. R. Fluoride inhibition of enolase activity in vivo and its relationship to the inhibition of glucose-6-P formation in Streptococcus salivarius. Arch Biochem Biophys. 1971 Sep;146(1):167–174. doi: 10.1016/s0003-9861(71)80053-x. [DOI] [PubMed] [Google Scholar]
- Kashket E. R., Wilson T. H. Protonmotive force in fermenting Streptococcus lactis 7962 in relation to sugar accumulation. Biochem Biophys Res Commun. 1974 Aug 5;59(3):879–886. doi: 10.1016/s0006-291x(74)80061-6. [DOI] [PubMed] [Google Scholar]
- Kemp C. W., Robrish S. A., Curtis M. A., Sharer S. A., Bowen W. H. Application of a competition model to the growth of Streptococcus mutans and Streptococcus sanguis in binary continuous culture. Appl Environ Microbiol. 1983 Apr;45(4):1277–1282. doi: 10.1128/aem.45.4.1277-1282.1983. [DOI] [PMC free article] [PubMed] [Google Scholar]
- Komor E. Proton-coupled hexose transport in Chlorella vulgaris. FEBS Lett. 1973 Dec 15;38(1):16–18. doi: 10.1016/0014-5793(73)80501-0. [DOI] [PubMed] [Google Scholar]
- Kornberg H. L., Reeves R. E. Inducible phosphoenolpyruvate-dependent hexose phosphotransferase activities in Escherichia coli. Biochem J. 1972 Aug;128(5):1339–1344. doi: 10.1042/bj1281339. [DOI] [PMC free article] [PubMed] [Google Scholar]
- Lam V. M., Daruwalla K. R., Henderson P. J., Jones-Mortimer M. C. Proton-linked D-xylose transport in Escherichia coli. J Bacteriol. 1980 Jul;143(1):396–402. doi: 10.1128/jb.143.1.396-402.1980. [DOI] [PMC free article] [PubMed] [Google Scholar]
- Marsh P. D., Keevil C. W., McDermid A. S., Williamson M. I., Ellwood D. C. Inhibition by the antimicrobial agent chlorhexidine of acid production and sugar transport in oral streptococcal bacteria. Arch Oral Biol. 1983;28(3):233–240. doi: 10.1016/0003-9969(83)90152-8. [DOI] [PubMed] [Google Scholar]
- Marsh P. D., Williamson M. I., Keevil C. W., McDermid A. S., Ellwood D. C. Influence of sodium and potassium ions on acid production by washed cells of Streptococcus mutans ingbritt and Streptococcus sanguis NCTC 7865 grown in a chemostat. Infect Immun. 1982 May;36(2):476–483. doi: 10.1128/iai.36.2.476-483.1982. [DOI] [PMC free article] [PubMed] [Google Scholar]
- Mikx F. H., Van der Hoeven J. S. Symbiosis of Streptococcus mutans and Veillonella alcalescens in mixed continuous cultures. Arch Oral Biol. 1975 Jul;20(7):407–410. doi: 10.1016/0003-9969(75)90224-1. [DOI] [PubMed] [Google Scholar]
- Porter E. V., Chassy B. M., Holmlund C. E. Partial purification and properties of a specific glucokinase from Streptococcus mutans SL-1. Biochim Biophys Acta. 1980 Feb 14;611(2):289–298. doi: 10.1016/0005-2744(80)90064-9. [DOI] [PubMed] [Google Scholar]
- Preiss J., Greenberg E. Biosynthesis of bacterial glycogen: activator specificity of the adenosine diphosphate glucose pyrophosphorylases from the genus Rhodospirillum. J Bacteriol. 1981 Sep;147(3):711–719. doi: 10.1128/jb.147.3.711-719.1981. [DOI] [PMC free article] [PubMed] [Google Scholar]
- Saxton C. A. Determination by electron microscope autoradiography of the distribution in plaque of organisms that synthesize intracellular polysaccharide in situ. Caries Res. 1975;9(6):418–437. doi: 10.1159/000260171. [DOI] [PubMed] [Google Scholar]
- Schachtele C. F., Mayo J. A. Phosphoenolpyruvate-dependent glucose transport in oral streptococci. J Dent Res. 1973 Nov-Dec;52(6):1209–1215. doi: 10.1177/00220345730520060801. [DOI] [PubMed] [Google Scholar]
- Slee A. M., Tanzer J. M. Effect of growth conditions on sucrose phosphotransferase activity of Streptococcus mutans. Infect Immun. 1980 Mar;27(3):922–927. doi: 10.1128/iai.27.3.922-927.1980. [DOI] [PMC free article] [PubMed] [Google Scholar]
- Slee A. M., Tanzer J. M. Phosphoenolpyruvate-dependent sucrose phosphotransferase activity in Streptococcus mutans NCTC 10449. Infect Immun. 1979 Jun;24(3):821–828. doi: 10.1128/iai.24.3.821-828.1979. [DOI] [PMC free article] [PubMed] [Google Scholar]
- St Martin E. J., Wittenberger C. L. Characterization of a phosphoenolpyruvate-dependent sucrose phosphotransferase system in Streptococcus mutans. Infect Immun. 1979 Jun;24(3):865–868. doi: 10.1128/iai.24.3.865-868.1979. [DOI] [PMC free article] [PubMed] [Google Scholar]
- Stock J., Roseman S. A sodium-dependent sugar co-transport system in bacteria. Biochem Biophys Res Commun. 1971 Jul 2;44(1):132–138. doi: 10.1016/s0006-291x(71)80168-7. [DOI] [PubMed] [Google Scholar]
- Tsuchiya T., Raven J., Wilson T. H. Co-transport of Na+ and methul-beta-D-thiogalactopyranoside mediated by the melibiose transport system of Escherichia coli. Biochem Biophys Res Commun. 1977 May 9;76(1):26–31. doi: 10.1016/0006-291x(77)91663-1. [DOI] [PubMed] [Google Scholar]
- Vadeboncoeur C., Mayrand D., Trahan L. A comparative study of enzymes involved in glucose phosphorylation in oral streptococci. J Dent Res. 1982 Jan;61(1):60–65. doi: 10.1177/00220345820610011401. [DOI] [PubMed] [Google Scholar]
- West I. C. Lactose transport coupled to proton movements in Escherichia coli. Biochem Biophys Res Commun. 1970 Nov 9;41(3):655–661. doi: 10.1016/0006-291x(70)90063-x. [DOI] [PubMed] [Google Scholar]
- West I. C., Mitchell P. Stoicheiometry of lactose-H+ symport across the plasma membrane of Escherichia coli. Biochem J. 1973 Mar;132(3):587–592. doi: 10.1042/bj1320587. [DOI] [PMC free article] [PubMed] [Google Scholar]
- Yamada T., Carlsson J. Regulation of lactate dehydrogenase and change of fermentation products in streptococci. J Bacteriol. 1975 Oct;124(1):55–61. doi: 10.1128/jb.124.1.55-61.1975. [DOI] [PMC free article] [PubMed] [Google Scholar]
- van Houte J., Saxton C. A. Cell wall thickening and intracellular polysaccharide in microorganisms of the dental plaque. Caries Res. 1971;5(1):30–43. doi: 10.1159/000259730. [DOI] [PubMed] [Google Scholar]