Abstract
Isolated membrane vesicles from the obligately acidophilic bacterium Bacillus acidocaldarius generated an electrochemical gradient of protons (delta mu- H+) upon energization with ascorbate-phenazine methosulfate at pH 6.0 or 3.0. At pH 6.0, there was little or no transmembrane pH gradient (delta pH), but a transmembrane electrical potential (delta psi) of ca. -77 mV, positive out, was observed. At pH 3.0, a delta pH equivalent to - 100 mV, acid out, and a delta psi of -73 mV, positive out, were observed upon energization. The total magnitude of the delta mu- H+ was higher than that of whole cells at acid pH, but the very large delta pHs and the reversed delta psi s, i.e., inside positive, that are typical of acidophile cells were not observed in the vesicles. The vesicles exhibited energy-dependent accumulation of alpha-aminoisobutyric acid that was inhibited by both nigericin and valinomycin (plus K+) at pH 3.0 but was inhibited little by nigericin at pH 6.0.
Full text
PDF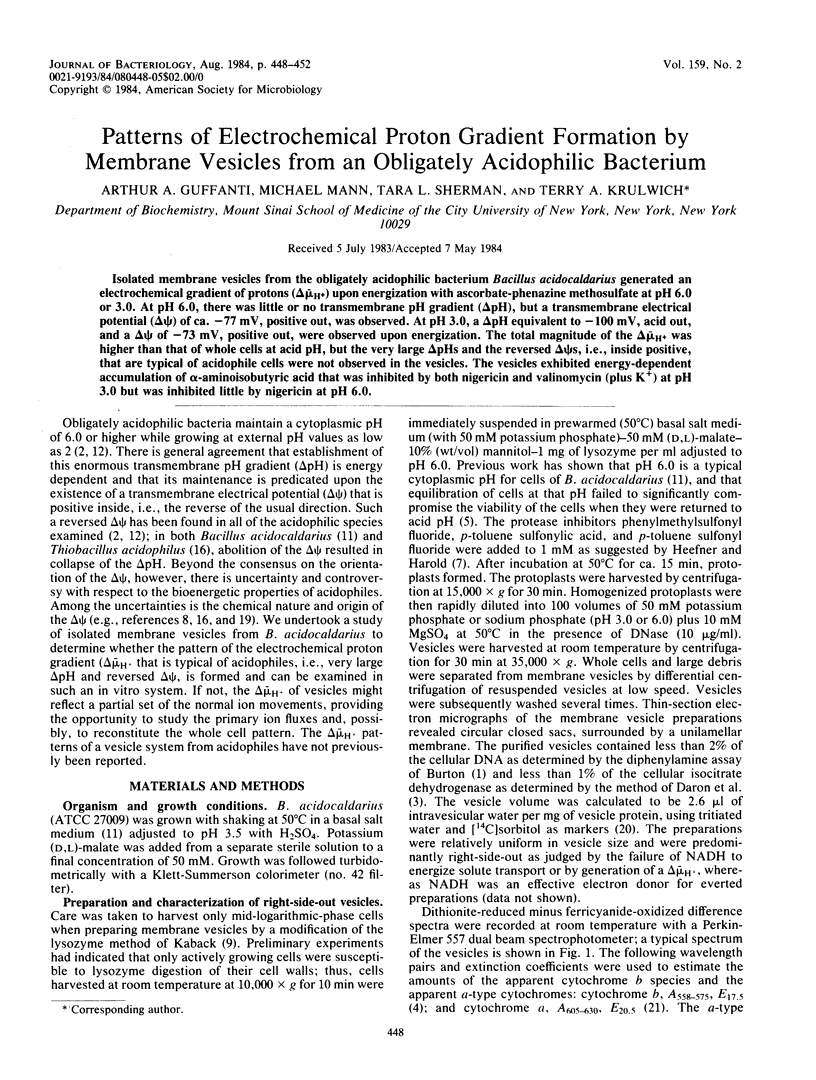
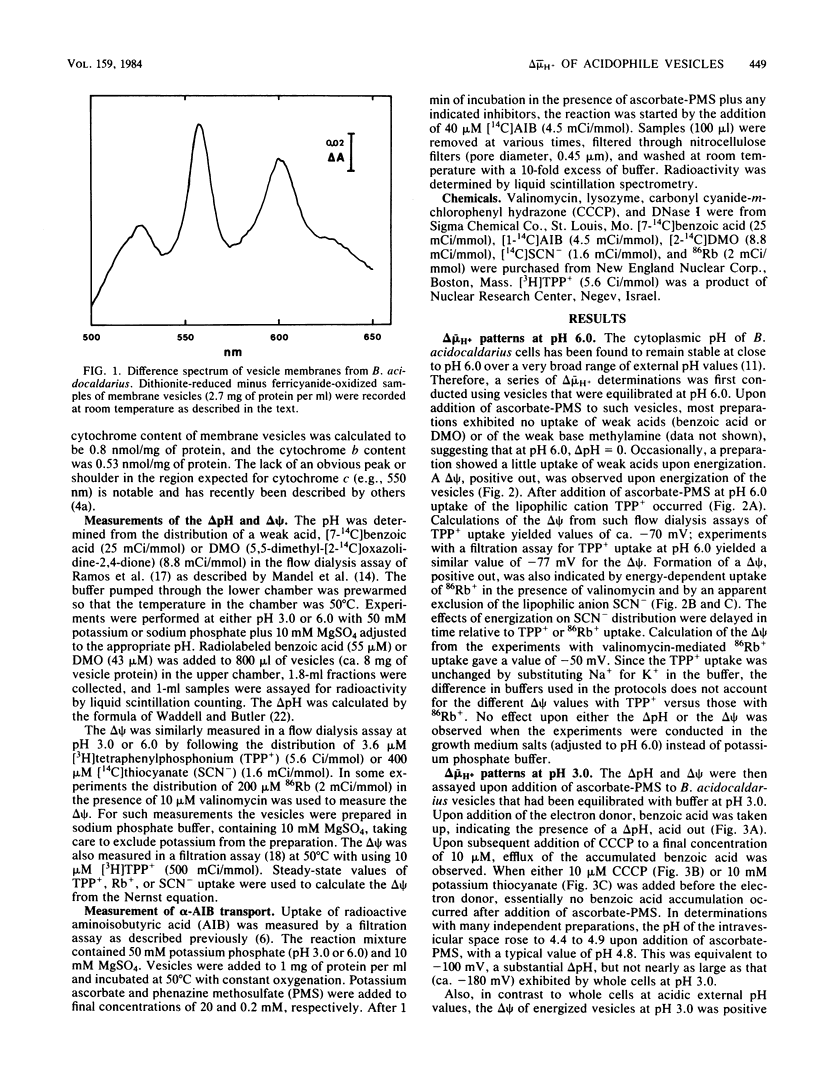
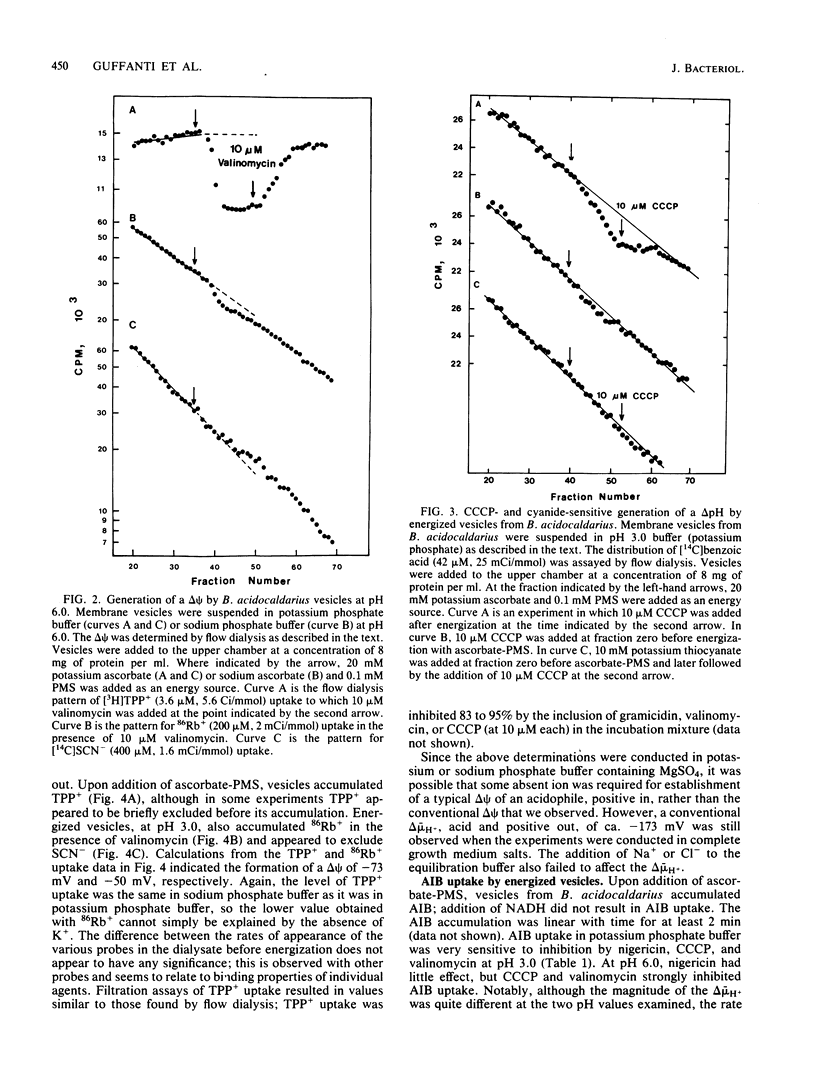
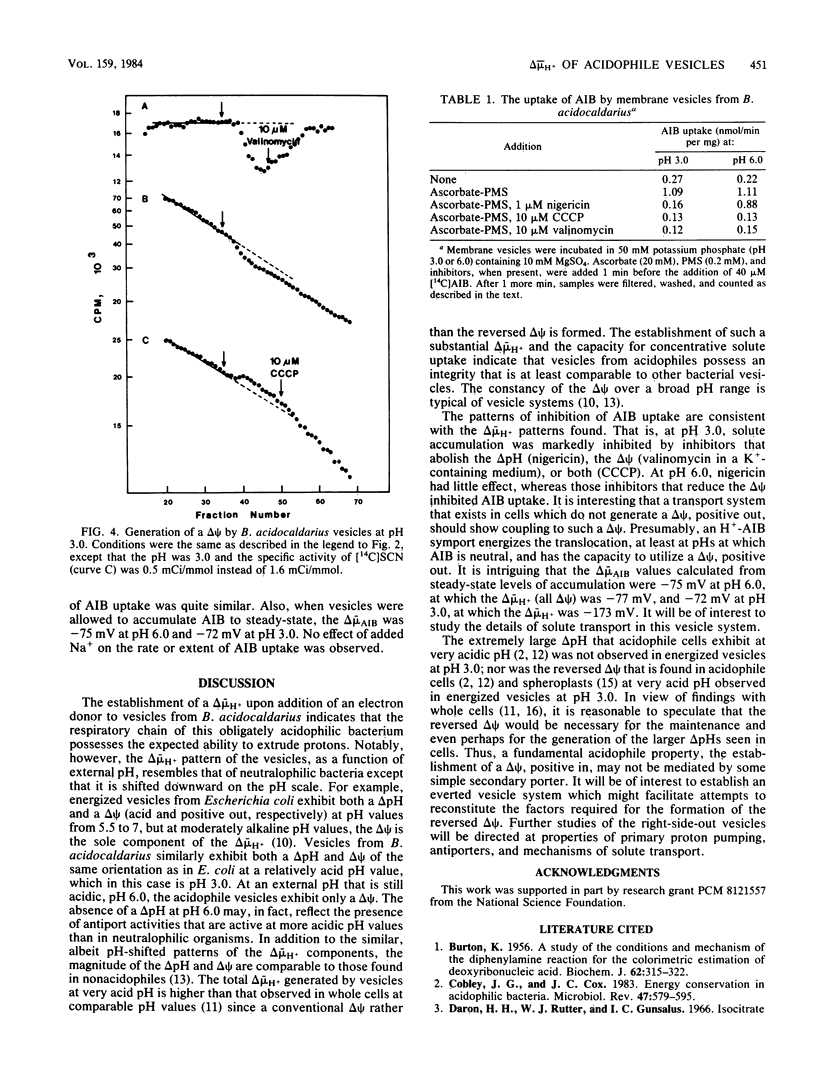
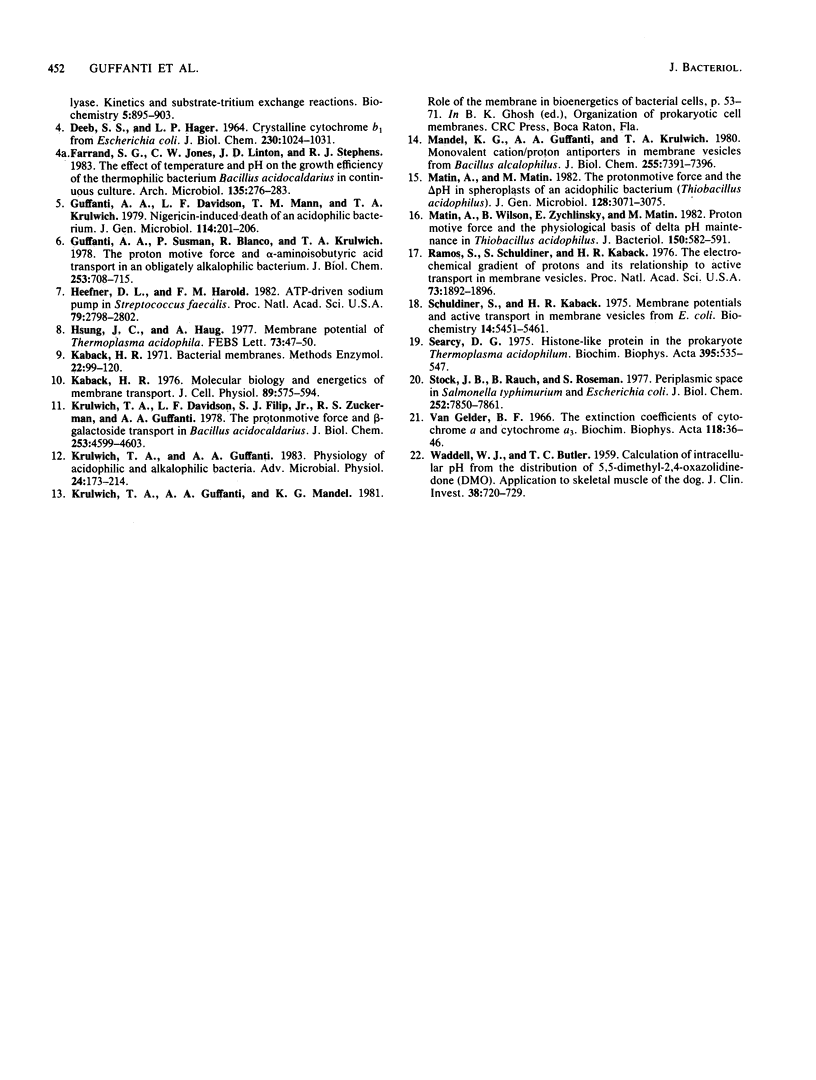
Selected References
These references are in PubMed. This may not be the complete list of references from this article.
- BURTON K. A study of the conditions and mechanism of the diphenylamine reaction for the colorimetric estimation of deoxyribonucleic acid. Biochem J. 1956 Feb;62(2):315–323. doi: 10.1042/bj0620315. [DOI] [PMC free article] [PubMed] [Google Scholar]
- Cobley J. G., Cox J. C. Energy conservation in acidophilic bacteria. Microbiol Rev. 1983 Dec;47(4):579–595. doi: 10.1128/mr.47.4.579-595.1983. [DOI] [PMC free article] [PubMed] [Google Scholar]
- DEEB S. S., HAGER L. P. CRYSTALLINE CYTOCHROME B1 FROM ESCHERICHIA COLI. J Biol Chem. 1964 Apr;239:1024–1031. [PubMed] [Google Scholar]
- Guffanti A. A., Davidson L. F., Mann T. M., Krulwich T. A. Nigericin-induced death of an acidophilic bacterium. J Gen Microbiol. 1979 Sep;114(1):201–206. doi: 10.1099/00221287-114-1-201. [DOI] [PubMed] [Google Scholar]
- Guffanti A. A., Susman P., Blanco R., Krulwich T. A. The protonmotive force and alpha-aminoisobutyric acid transport in an obligately alkalophilic bacterium. J Biol Chem. 1978 Feb 10;253(3):708–715. [PubMed] [Google Scholar]
- Heefner D. L., Harold F. M. ATP-driven sodium pump in Streptococcus faecalis. Proc Natl Acad Sci U S A. 1982 May;79(9):2798–2802. doi: 10.1073/pnas.79.9.2798. [DOI] [PMC free article] [PubMed] [Google Scholar]
- Hsung J. C., Haug A. Membrane potential of Thermoplasma acidophila. FEBS Lett. 1977 Jan 15;73(1):47–50. doi: 10.1016/0014-5793(77)80011-2. [DOI] [PubMed] [Google Scholar]
- Kaback H. R. Molecular biology and energetics of membrane transport. J Cell Physiol. 1976 Dec;89(4):575–593. doi: 10.1002/jcp.1040890414. [DOI] [PubMed] [Google Scholar]
- Krulwich T. A., Davidson L. F., Filip S. J., Jr, Zuckerman R. S., Guffanti A. A. The protonmotive force and beta-galactoside transport in Bacillus acidocaldarius. J Biol Chem. 1978 Jul 10;253(13):4599–4603. [PubMed] [Google Scholar]
- Krulwich T. A., Guffanti A. A. Physiology of acidophilic and alkalophilic bacteria. Adv Microb Physiol. 1983;24:173–214. doi: 10.1016/s0065-2911(08)60386-0. [DOI] [PubMed] [Google Scholar]
- Mandel K. G., Guffanti A. A., Krulwich T. A. Monovalent cation/proton antiporters in membrane vesicles from Bacillus alcalophilus. J Biol Chem. 1980 Aug 10;255(15):7391–7396. [PubMed] [Google Scholar]
- Matin A., Wilson B., Zychlinsky E., Matin M. Proton motive force and the physiological basis of delta pH maintenance in thiobacillus acidophilus. J Bacteriol. 1982 May;150(2):582–591. doi: 10.1128/jb.150.2.582-591.1982. [DOI] [PMC free article] [PubMed] [Google Scholar]
- Ramos S., Schuldiner S., Kaback H. R. The electrochemical gradient of protons and its relationship to active transport in Escherichia coli membrane vesicles. Proc Natl Acad Sci U S A. 1976 Jun;73(6):1892–1896. doi: 10.1073/pnas.73.6.1892. [DOI] [PMC free article] [PubMed] [Google Scholar]
- Schuldiner S., Kaback H. R. Membrane potential and active transport in membrane vesicles from Escherichia coli. Biochemistry. 1975 Dec 16;14(25):5451–5461. doi: 10.1021/bi00696a011. [DOI] [PubMed] [Google Scholar]
- Searcy D. G. Histone-like protein in the prokaryote Thermoplasma acidophilum. Biochim Biophys Acta. 1975 Jul 23;395(4):535–547. doi: 10.1016/0005-2787(75)90076-3. [DOI] [PubMed] [Google Scholar]
- Stock J. B., Rauch B., Roseman S. Periplasmic space in Salmonella typhimurium and Escherichia coli. J Biol Chem. 1977 Nov 10;252(21):7850–7861. [PubMed] [Google Scholar]
- WADDELL W. J., BUTLER T. C. Calculation of intracellular pH from the distribution of 5,5-dimethyl-2,4-oxazolidinedione (DMO); application to skeletal muscle of the dog. J Clin Invest. 1959 May;38(5):720–729. doi: 10.1172/JCI103852. [DOI] [PMC free article] [PubMed] [Google Scholar]
- van Gelder B. F. On cytochrome c oxidase. I. The extinction coefficients of cytochrome a and cytochrome a3. Biochim Biophys Acta. 1966 Apr 12;118(1):36–46. doi: 10.1016/s0926-6593(66)80142-x. [DOI] [PubMed] [Google Scholar]