Abstract
Measurements of the electrochemical gradient of hydrogen ions, which gives rise to the proton motive force (PMF), were carried out with growing Streptococcus lactis and Staphylococcus aureus cells. The facultative anaerobe was chosen in order to compare the PMF of cells growing aerobically and anaerobically. It was expected that during aerobic growth the cells would have a higher PMF than during anaerobic growth, because the H+-translocating ATPase (BF0F1) operates in the direction of H+ influx and ATP synthesis during respiration, whereas under anaerobic conditions the BF0F1 hydrolyzes glycolytically generated ATP and establishes the proton gradient by extruding H+. The electrical component of the PMF, delta psi, and the chemical gradient of H+, delta pH, were measured with radiolabeled tetraphenylphosphonium and benzoate ions. In both S. lactis and S. aureus cells, the PMF was constant during the exponential phase of batch growth and decreased in the stationary phase. In both species of bacteria, the exponential-phase PMF was not affected by varying the growth rate by adding different sugars to the medium. The relative contributions of delta psi and delta pH to the PMF, however, depended on the pH of the medium. The internal pH of S. aureus was constant at pH 7.4 to 7.6 under all conditions of growth tested. Under aerobic conditions, the delta psi of exponential phase S. aureus remained fairly constant at 160 to 170 mV. Thus, the PMF was 250 to 270 mV in cells growing aerobically in media at pH 6 and progressively lower in media of higher pH, reaching 195 to 205 mV at pH 7. Under anaerobic conditions, the delta psi ranged from 100 to 120 mV in cells at pH 6.3 to 7, resulting in a PMF of 150 to 140 mV. Thus, the mode of energy metabolism (i.e., respiration versus fermentation) and the pH of the medium are the two important factors influencing the PMF of these gram-positive cells during growth.
Full text
PDF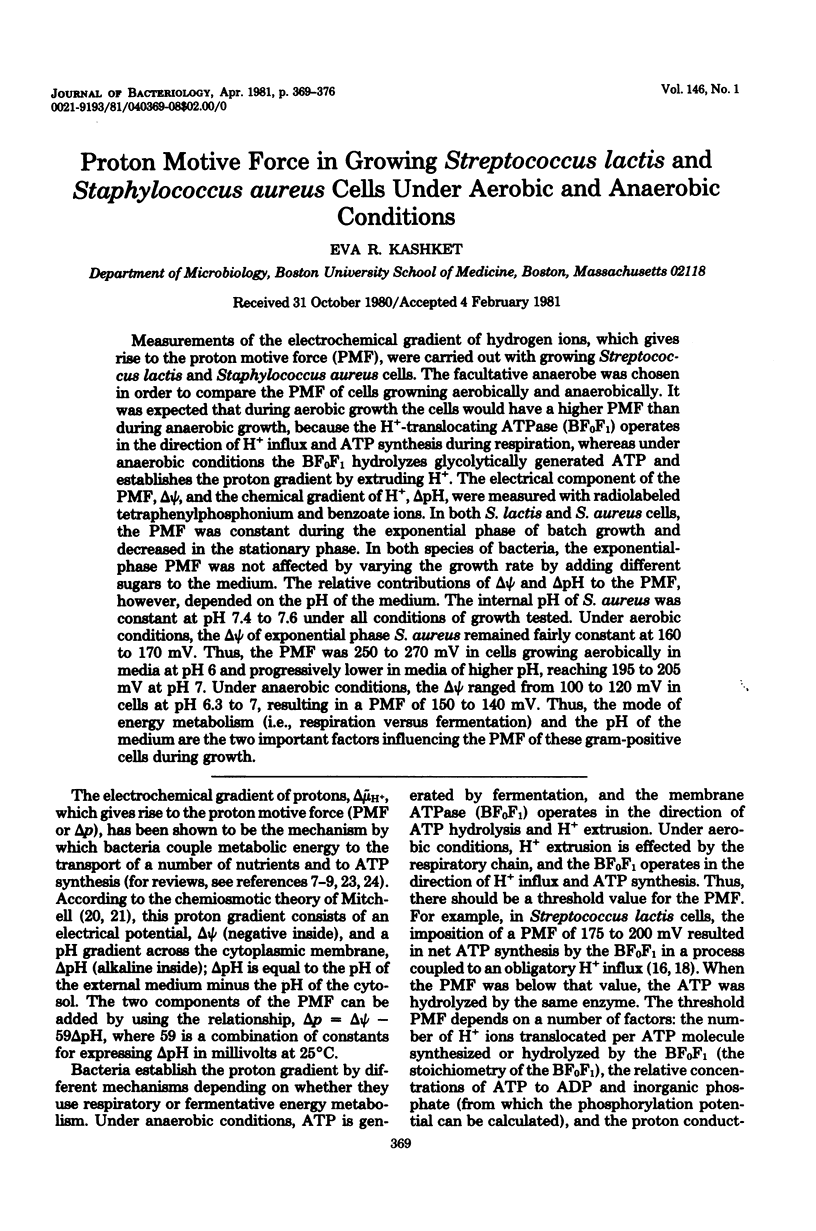
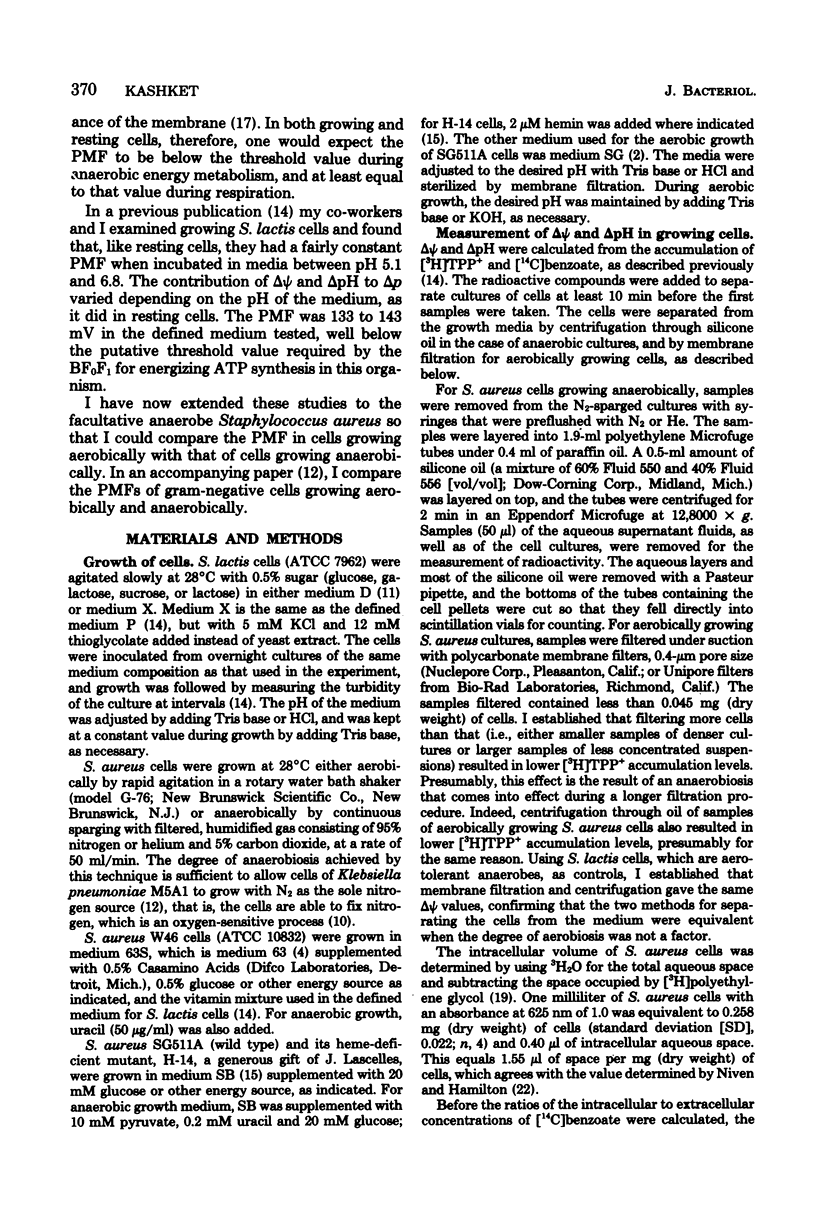
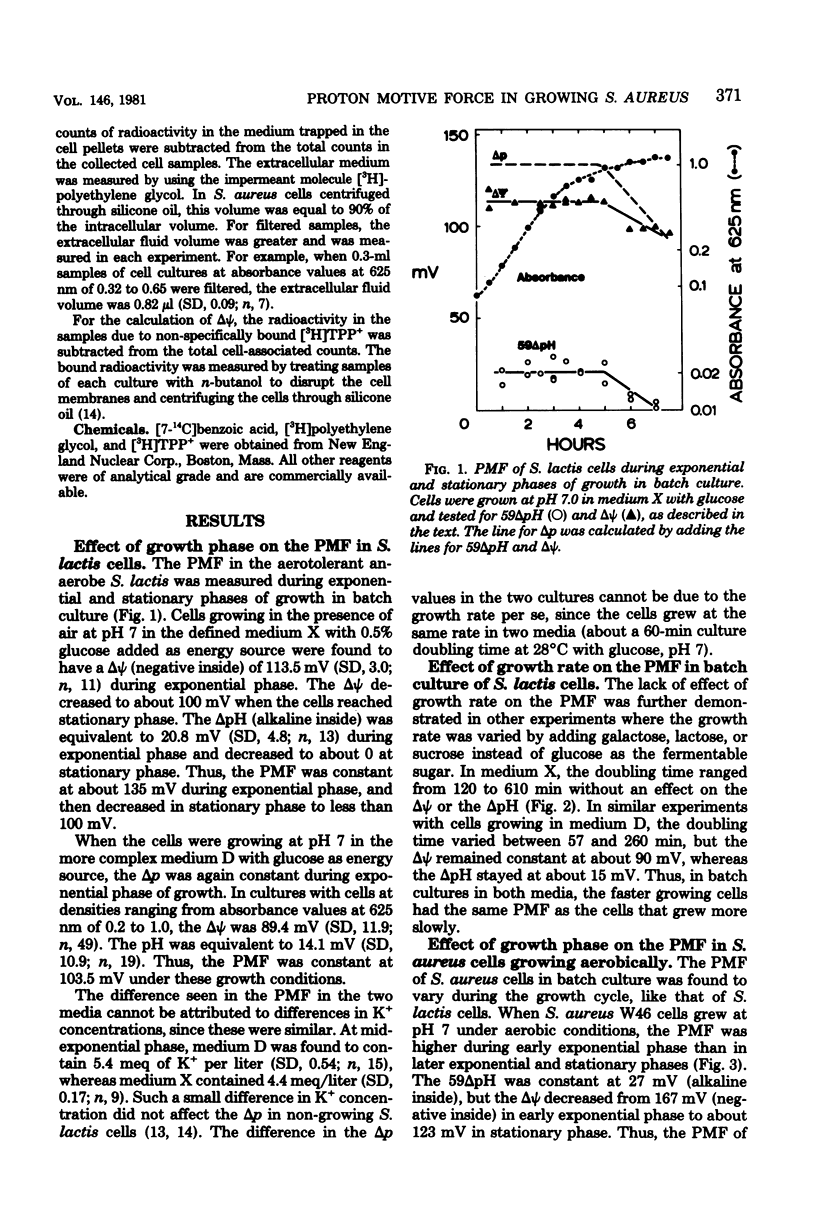
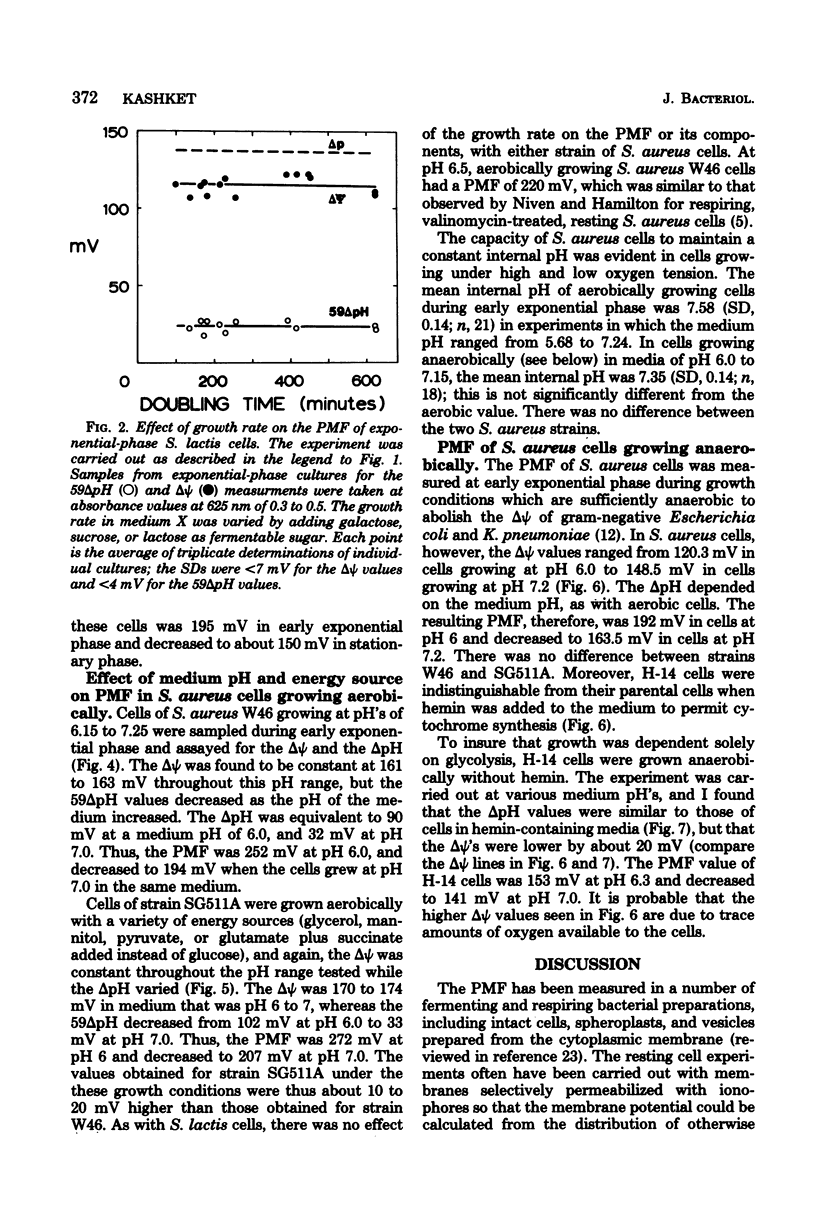
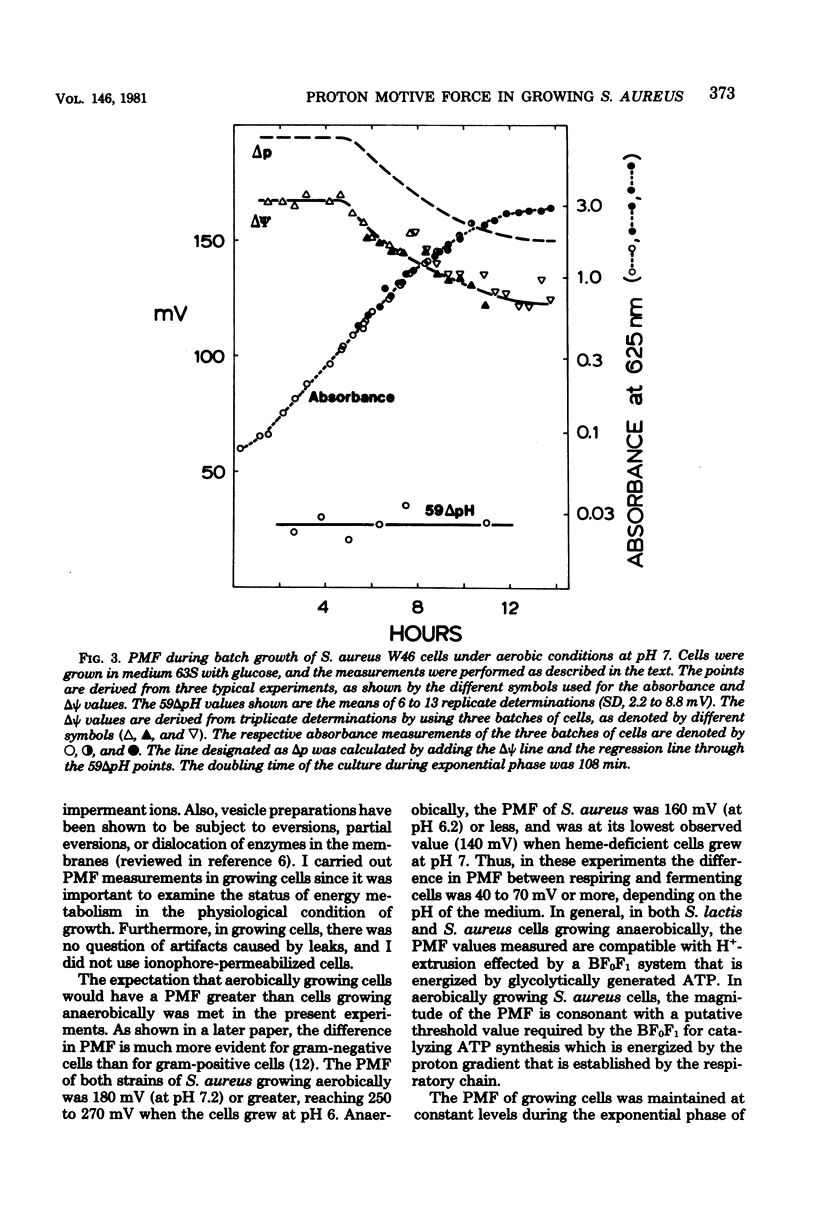
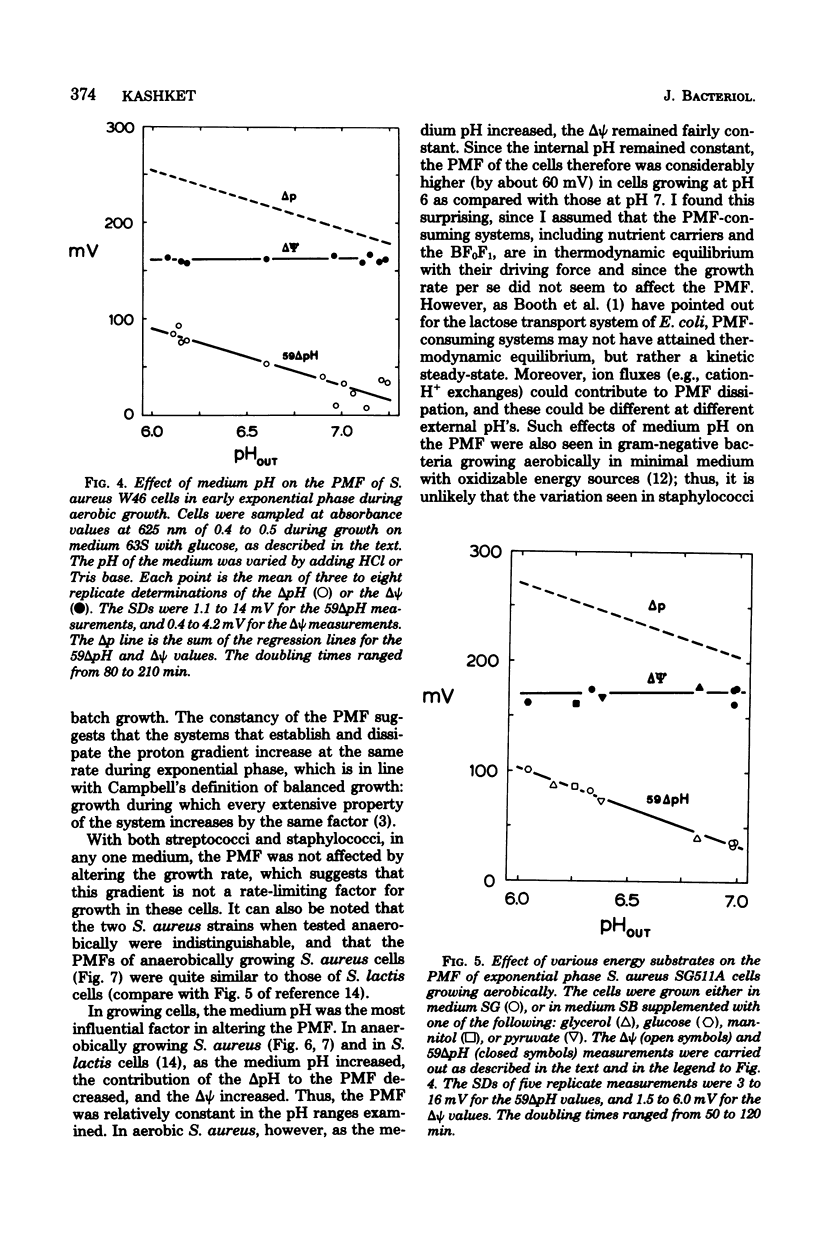
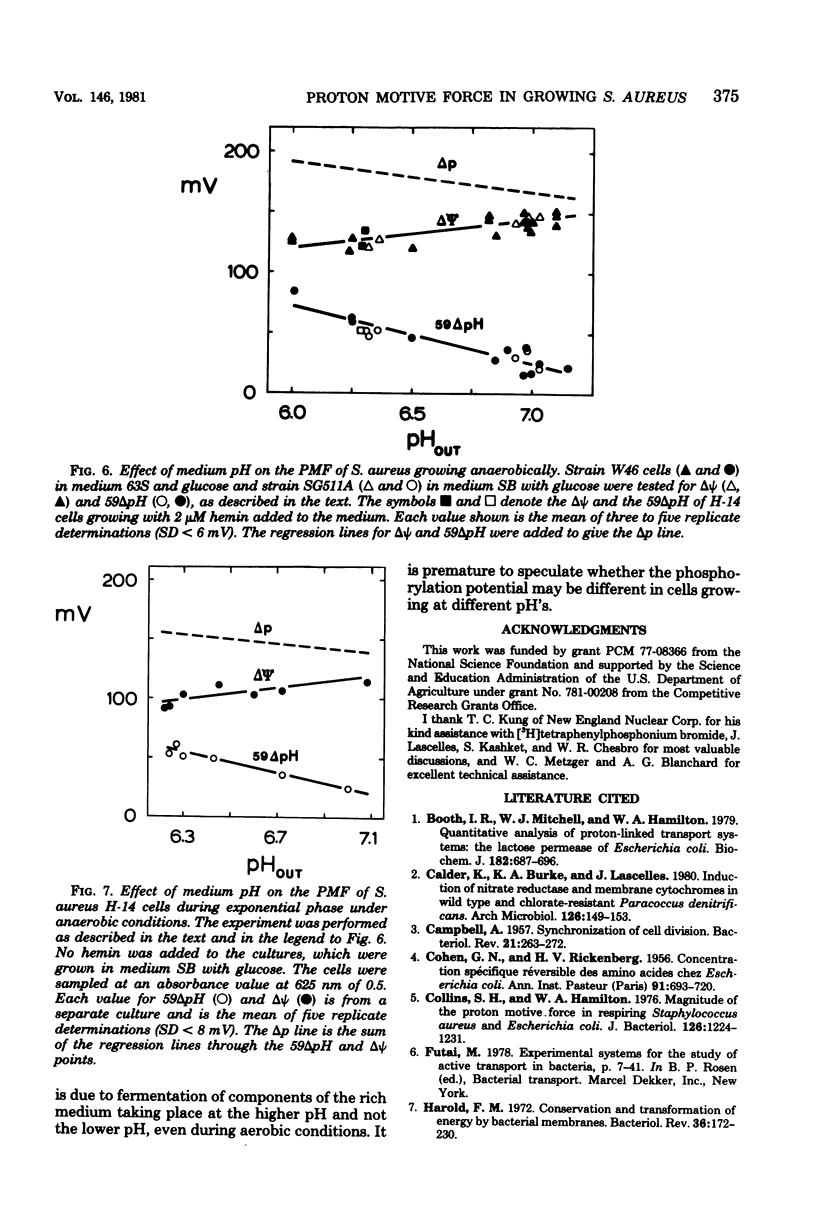
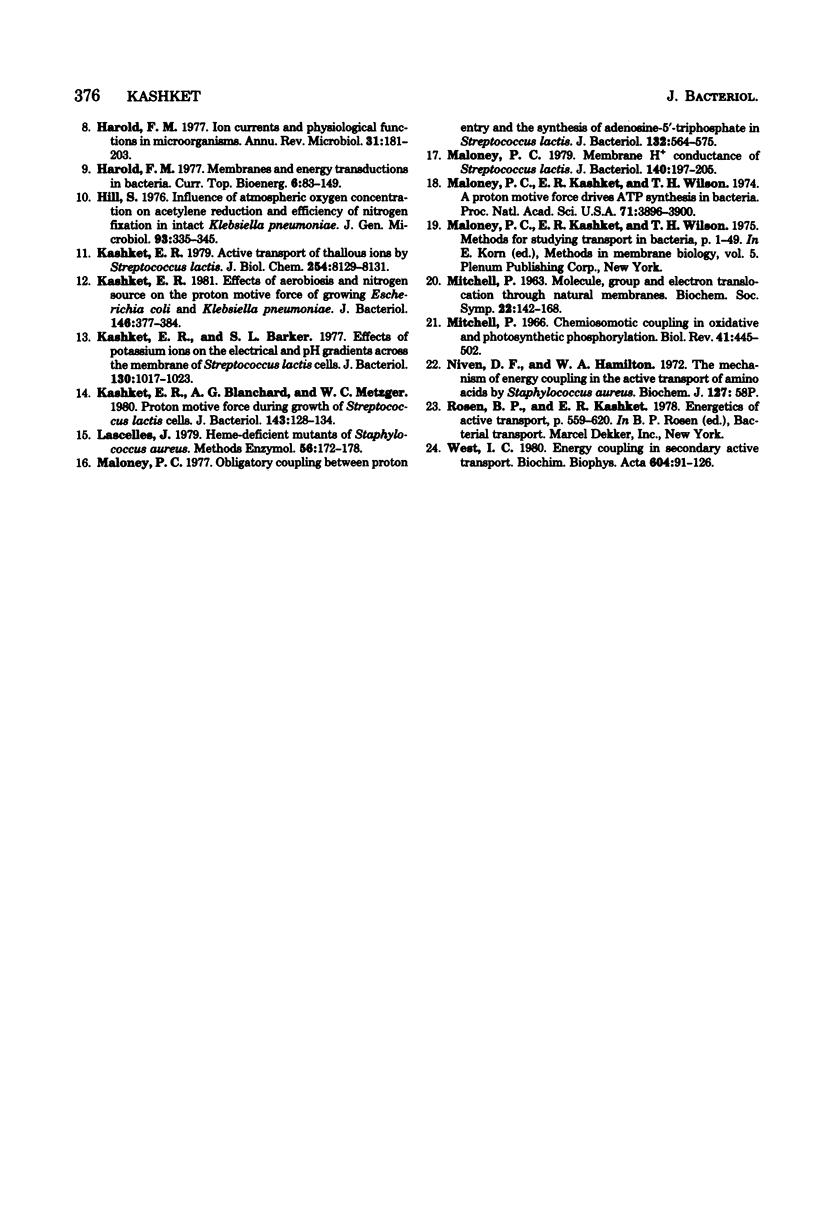
Selected References
These references are in PubMed. This may not be the complete list of references from this article.
- Booth I. R., Mitchell W. J., Hamilton W. A. Quantitative analysis of proton-linked transport systems. The lactose permease of Escherichia coli. Biochem J. 1979 Sep 15;182(3):687–696. doi: 10.1042/bj1820687. [DOI] [PMC free article] [PubMed] [Google Scholar]
- CAMPBELL A. Synchronization of cell division. Bacteriol Rev. 1957 Dec;21(4):263–272. doi: 10.1128/br.21.4.263-272.1957. [DOI] [PMC free article] [PubMed] [Google Scholar]
- COHEN G. N., RICKENBERG H. V. Concentration spécifique réversible des amino acides chez Escherichia coli. Ann Inst Pasteur (Paris) 1956 Nov;91(5):693–720. [PubMed] [Google Scholar]
- Calder K., Burke K. A., Lascelles J. Induction of nitrate reductase and membrane cytochromes in wild type and chlorate-resistant Paracoccus denitrificans. Arch Microbiol. 1980 Jun;126(2):149–153. doi: 10.1007/BF00511220. [DOI] [PubMed] [Google Scholar]
- Collins S. H., Hamilton W. A. Magnitude of the protonmotive force in respiring Staphylococcus aureus and Escherichia coli. J Bacteriol. 1976 Jun;126(3):1224–1231. doi: 10.1128/jb.126.3.1224-1231.1976. [DOI] [PMC free article] [PubMed] [Google Scholar]
- Harold F. M. Conservation and transformation of energy by bacterial membranes. Bacteriol Rev. 1972 Jun;36(2):172–230. doi: 10.1128/br.36.2.172-230.1972. [DOI] [PMC free article] [PubMed] [Google Scholar]
- Harold F. M. Ion currents and physiological functions in microorganisms. Annu Rev Microbiol. 1977;31:181–203. doi: 10.1146/annurev.mi.31.100177.001145. [DOI] [PubMed] [Google Scholar]
- Kashket E. R. Active transport of thallous ions by Streptococcus lactis. J Biol Chem. 1979 Sep 10;254(17):8129–8131. [PubMed] [Google Scholar]
- Kashket E. R., Barker S. L. Effects of potassium ions on the electrical and pH gradients across the membrane of Streptococcus lactis cells. J Bacteriol. 1977 Jun;130(3):1017–1023. doi: 10.1128/jb.130.3.1017-1023.1977. [DOI] [PMC free article] [PubMed] [Google Scholar]
- Kashket E. R. Effects of aerobiosis and nitrogen source on the proton motive force in growing Escherichia coli and Klebsiella pneumoniae cells. J Bacteriol. 1981 Apr;146(1):377–384. doi: 10.1128/jb.146.1.377-384.1981. [DOI] [PMC free article] [PubMed] [Google Scholar]
- Lascelles J. Heme-deficient mutants of Staphylococcus aureus. Methods Enzymol. 1979;56:172–178. doi: 10.1016/0076-6879(79)56019-4. [DOI] [PubMed] [Google Scholar]
- Maloney P. C. Membrane H+ conductance of Streptococcus lactis. J Bacteriol. 1979 Oct;140(1):197–205. doi: 10.1128/jb.140.1.197-205.1979. [DOI] [PMC free article] [PubMed] [Google Scholar]
- Maloney P. C. Obligatory coupling between proton entry and the synthesis of adenosine 5'-triphosphate in Streptococcus lactis. J Bacteriol. 1977 Nov;132(2):564–575. doi: 10.1128/jb.132.2.564-575.1977. [DOI] [PMC free article] [PubMed] [Google Scholar]
- Mitchell P. Chemiosmotic coupling in oxidative and photosynthetic phosphorylation. Biol Rev Camb Philos Soc. 1966 Aug;41(3):445–502. doi: 10.1111/j.1469-185x.1966.tb01501.x. [DOI] [PubMed] [Google Scholar]
- Niven D. F., Hamilton W. A. The mechanism of energy coupling in the active transport of amino acids by Staphylococcus aureus. Biochem J. 1972 Apr;127(3):58P–58P. doi: 10.1042/bj1270058pa. [DOI] [PMC free article] [PubMed] [Google Scholar]
- West I. C. Energy coupling in secondary active transport. Biochim Biophys Acta. 1980 May 27;604(1):91–126. doi: 10.1016/0005-2736(80)90586-6. [DOI] [PubMed] [Google Scholar]