Abstract
Lactobacillus plantarum is aerotolerant during log-phase growth on glucose, but is an obligate aerobe on polyols. Respiration was cyanide resistant and under certain conditions was associated with the accumulation of millimolar concentrations of H2O2. On glucose, optimal growth was observed in the absence of O2. Extracts of L. plantarum did not catalyze the reduction of paraquat by reduced nicotinamide adenine dinucleotide, but plumbagin (5-hydroxy-2-methyl-1,4-naphthoquinone) was readily reduced. Such extracts produced O2− in the presence of NADH plus plumbagin. Plumbagin caused a 10-fold increase in the rate of respiration of intact cells in the presence of glucose and also imposed a loss of viability which was dependent upon both glucose and O2. Although extracts of L. plantarum were devoid of true superoxide dismutase activity, this organism was comparable to superoxide dismutase-containing species in its resistance toward hyperbaric O2 and toward the oxygen-dependent lethality of plumbagin. L. plantarum required Mn-rich media and actively accumulated Mn(II). Soluble extracts were found to contain approximately 9 μg of Mn per mg of protein and 75 to 90% of this Mn was dialyzable. Such extracts exhibited a dialyzable and ethylenediaminetetraacetic acid-inhibitable ability to scavenge O2−. This O2−-scavenging activity was due to the dialyzable Mn(II) present in these extracts and could be mimicked by MnCl2. Cells grown in Mn-rich media were enriched in dialyzable Mn and were more resistant toward oxygen toxicity and toward the oxygen-dependent plumbagin toxicity than were cells grown in Mn-deficient media. L. plantarum exhibited no nutritional requirement for iron and little or no iron was present in these cells, even when they were grown in iron-rich media. L. plantarum thus appears to use millimolar levels of Mn(II) to scavenge O2−, much as most other organisms use micromolar levels of superoxide dismutases.
Full text
PDF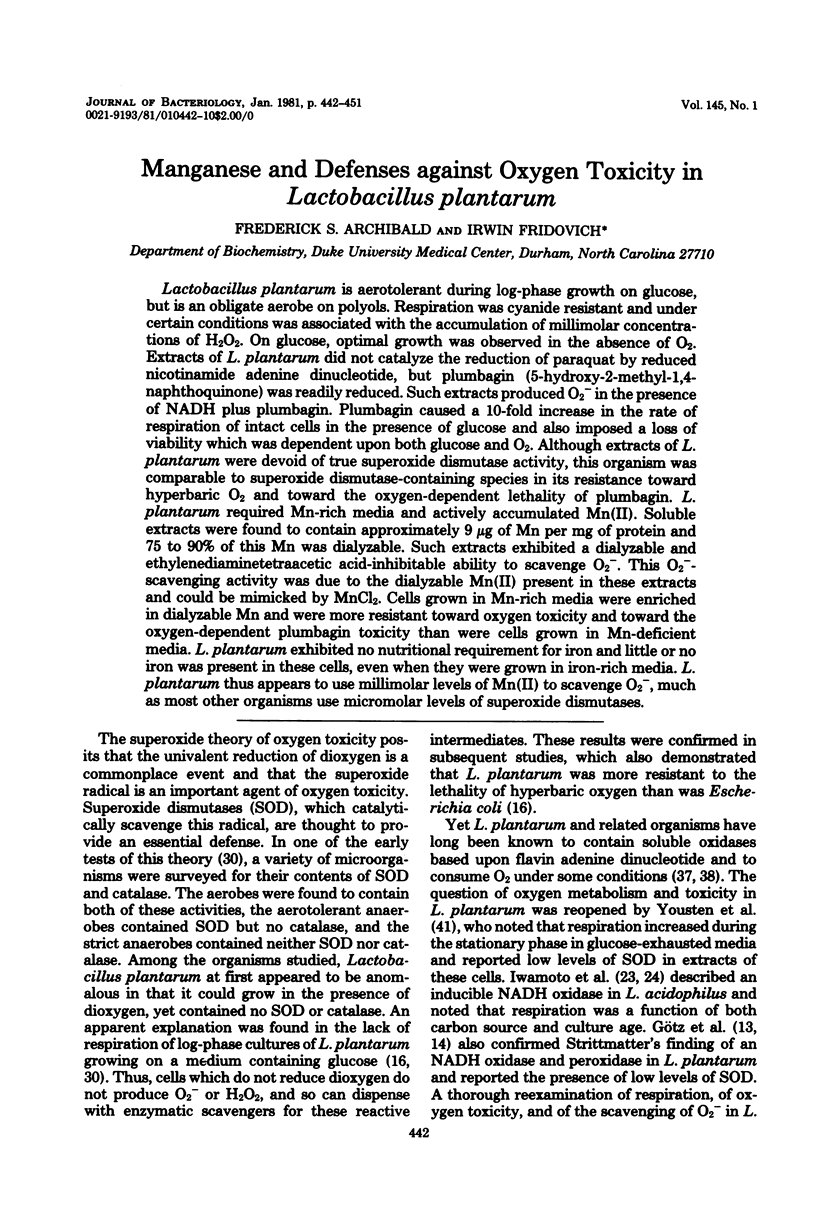
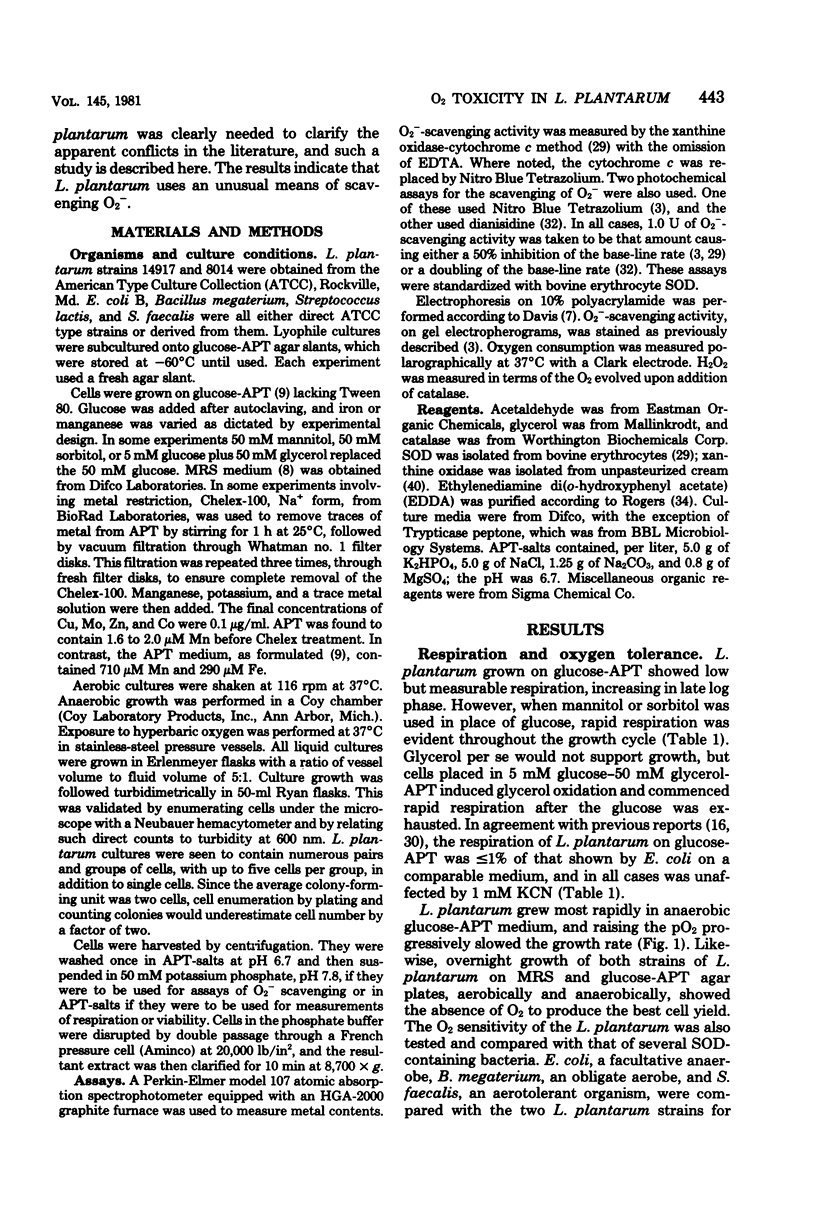
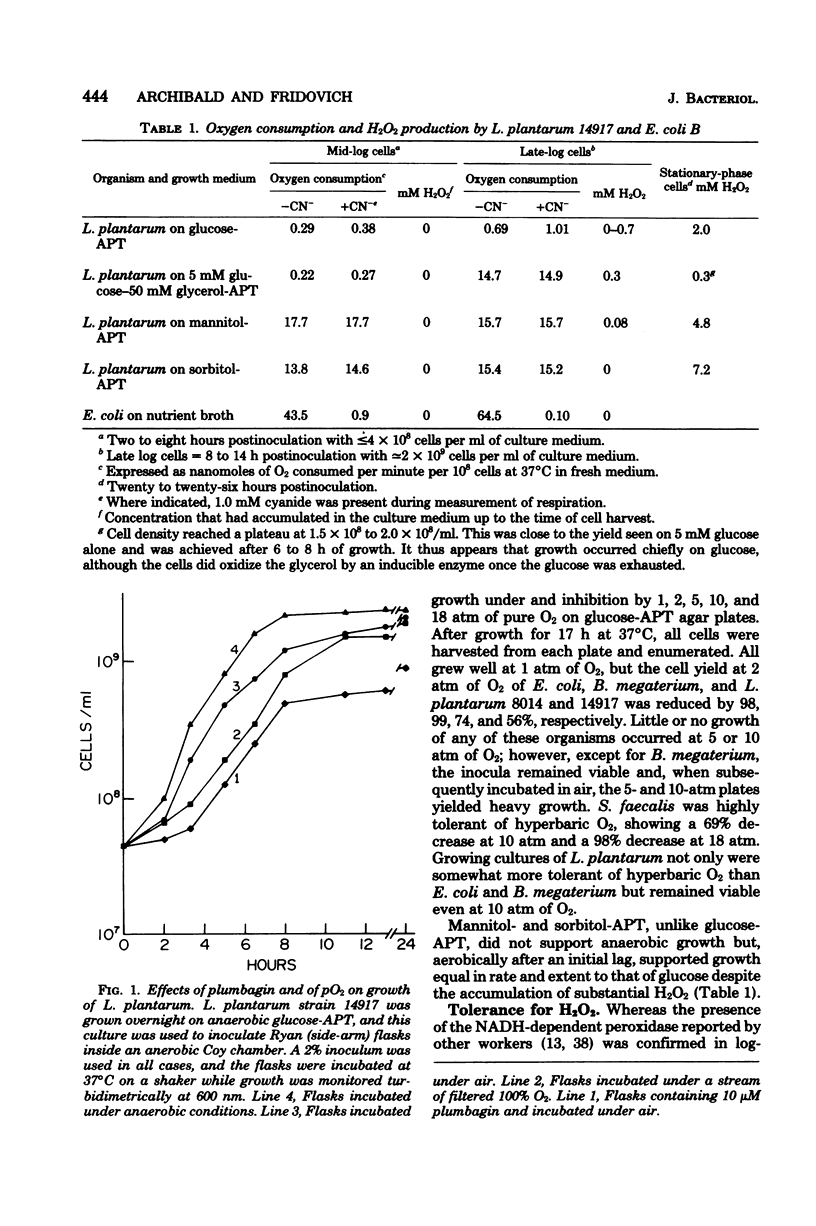
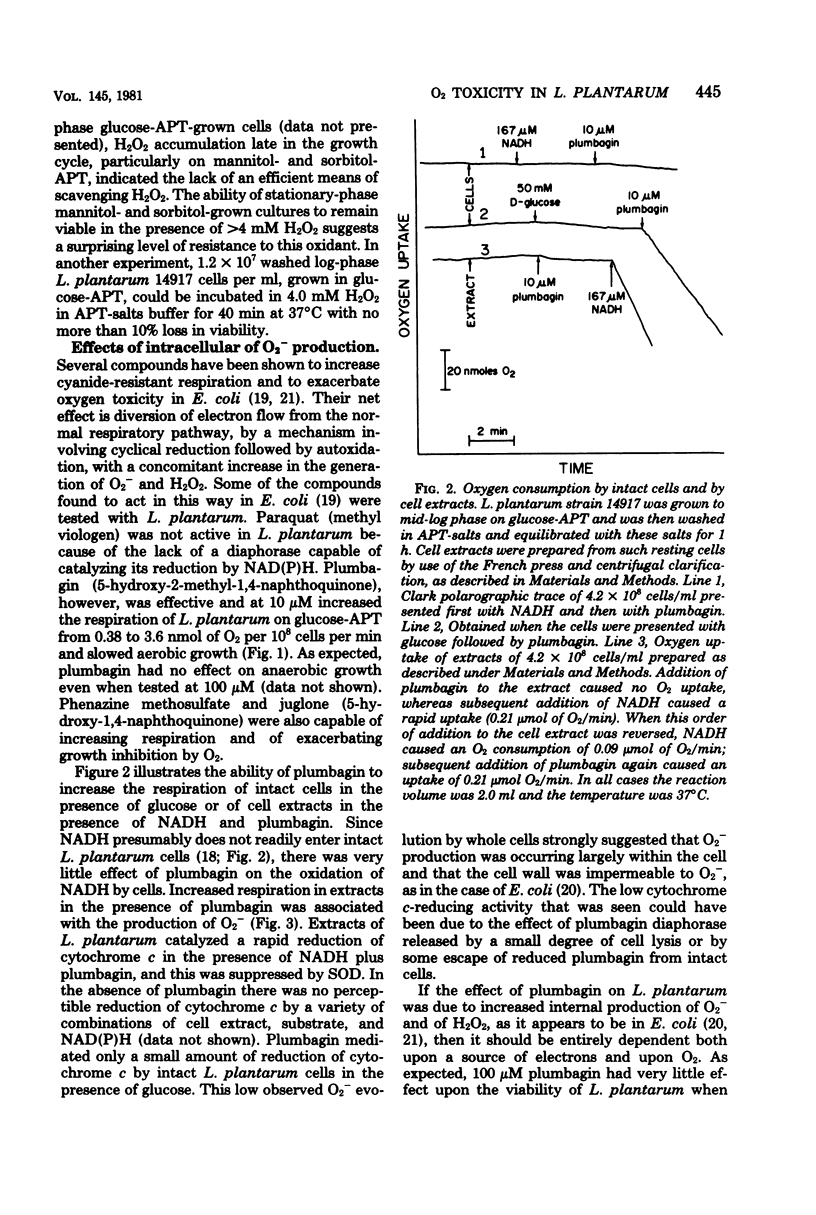
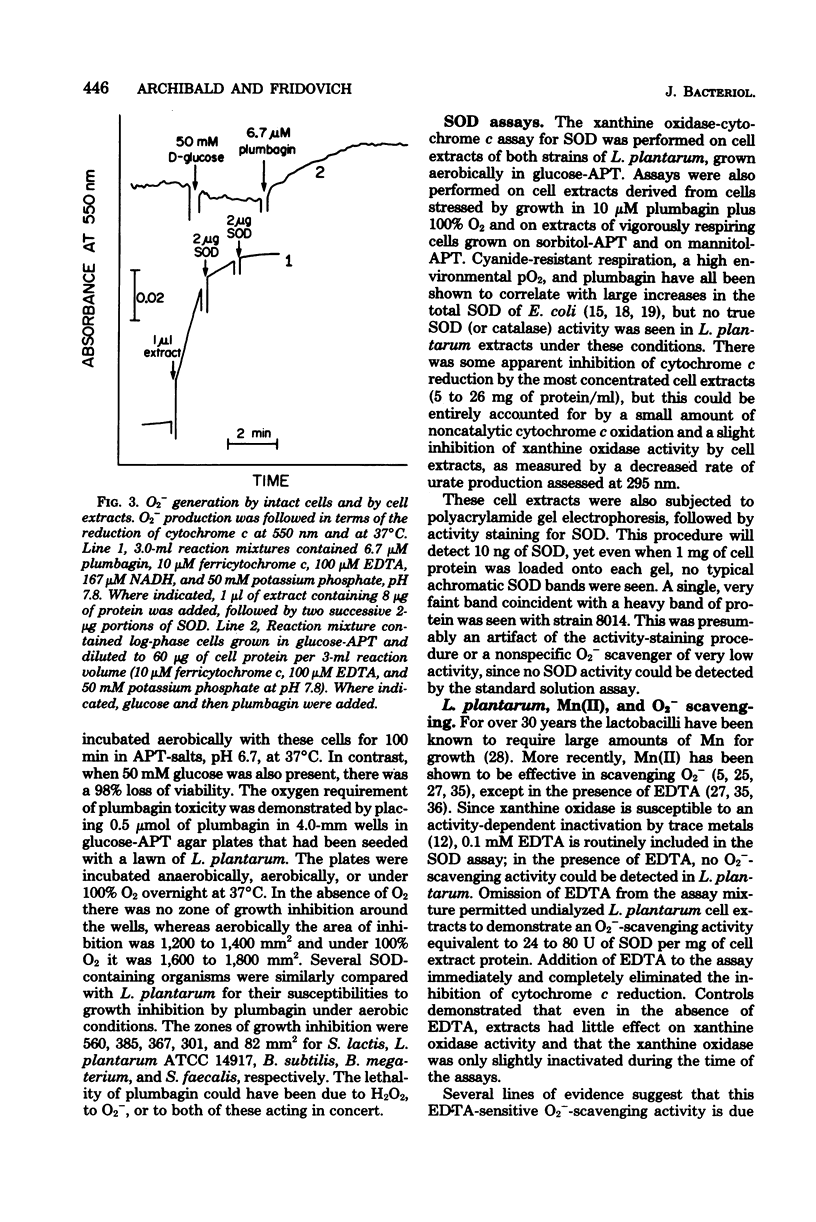
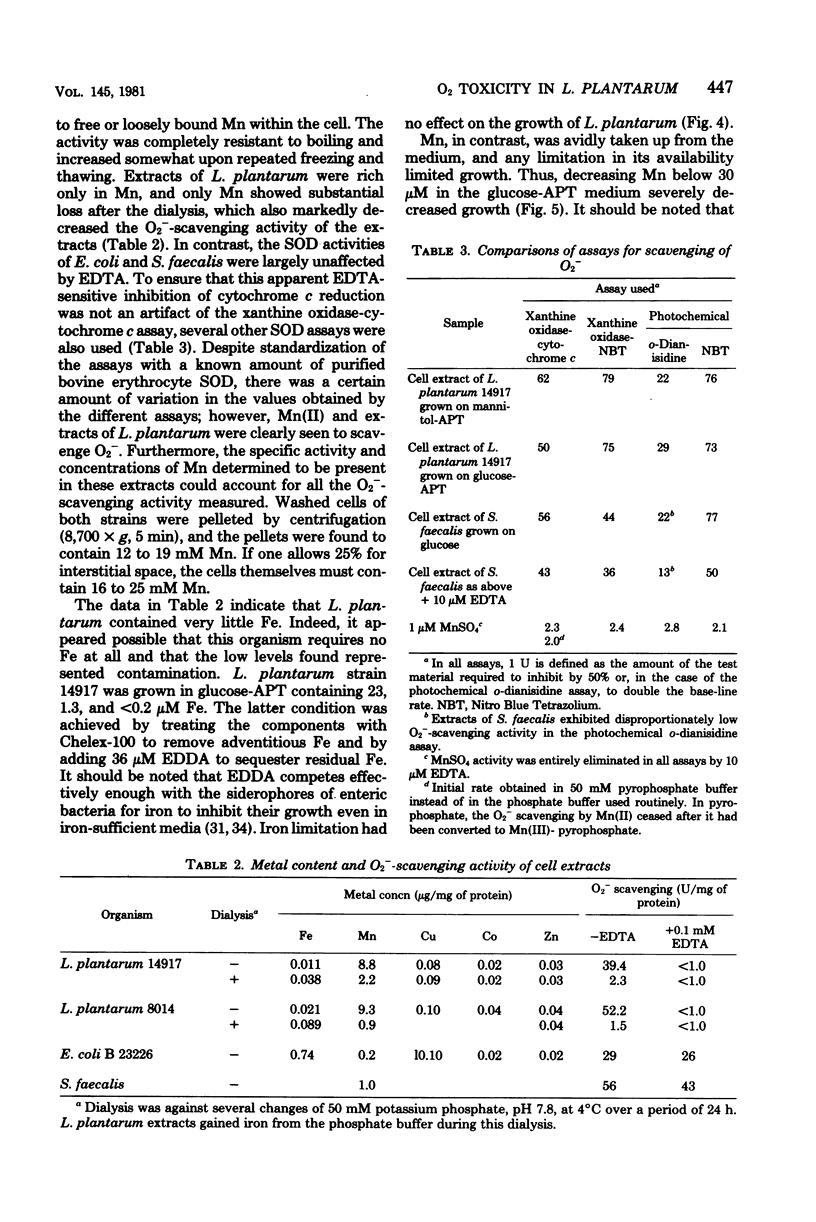
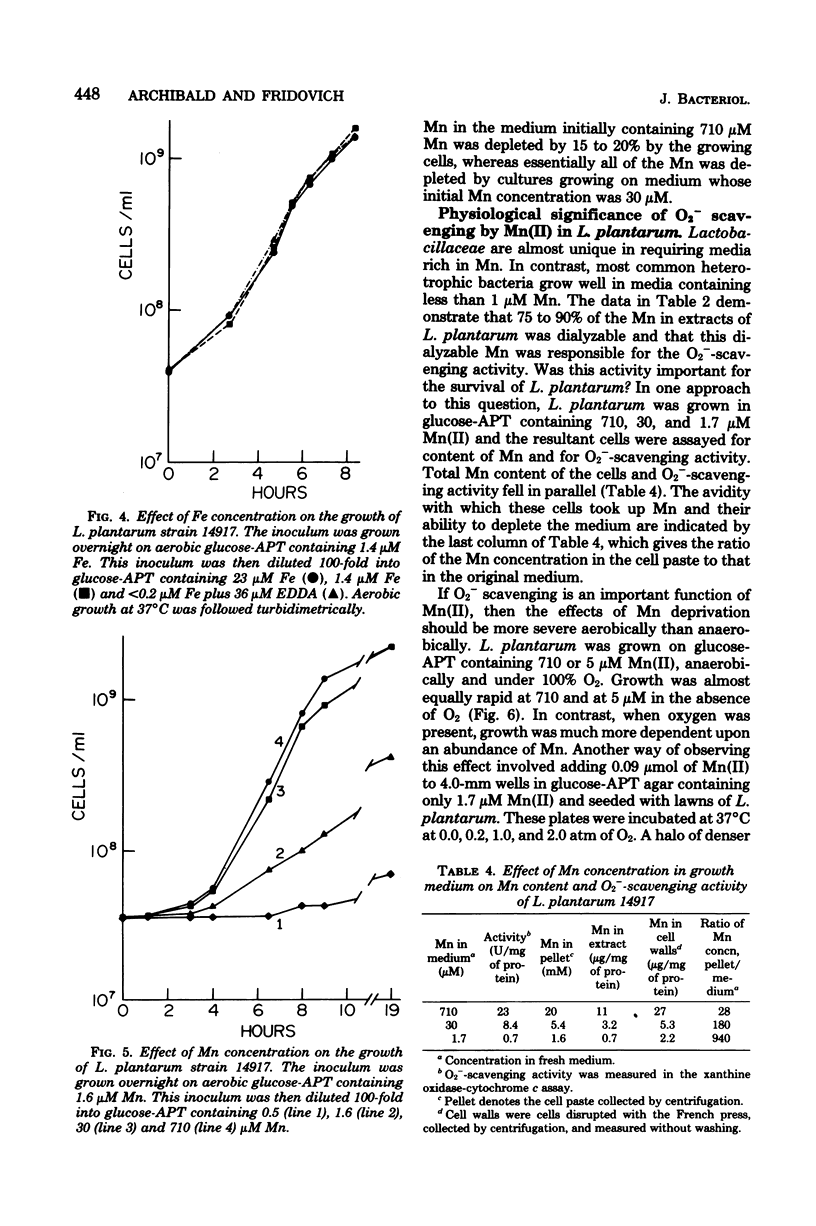
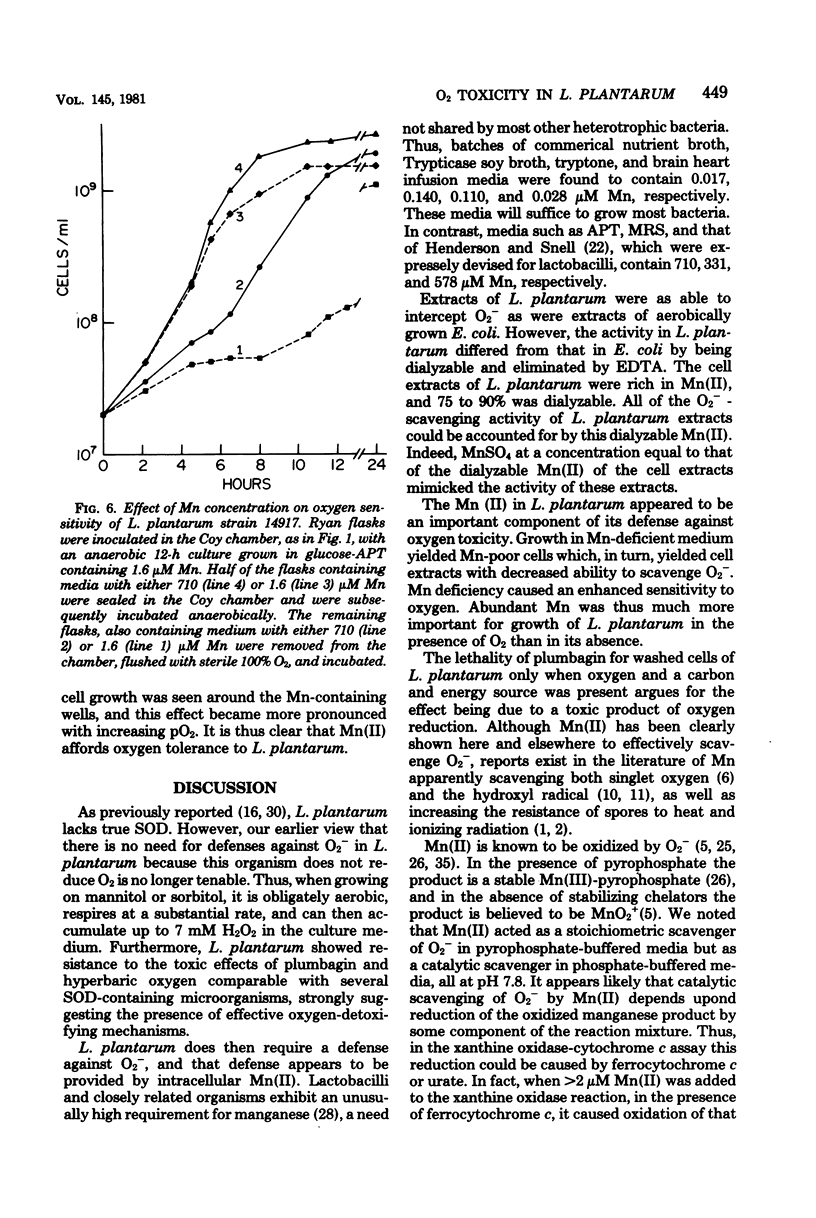
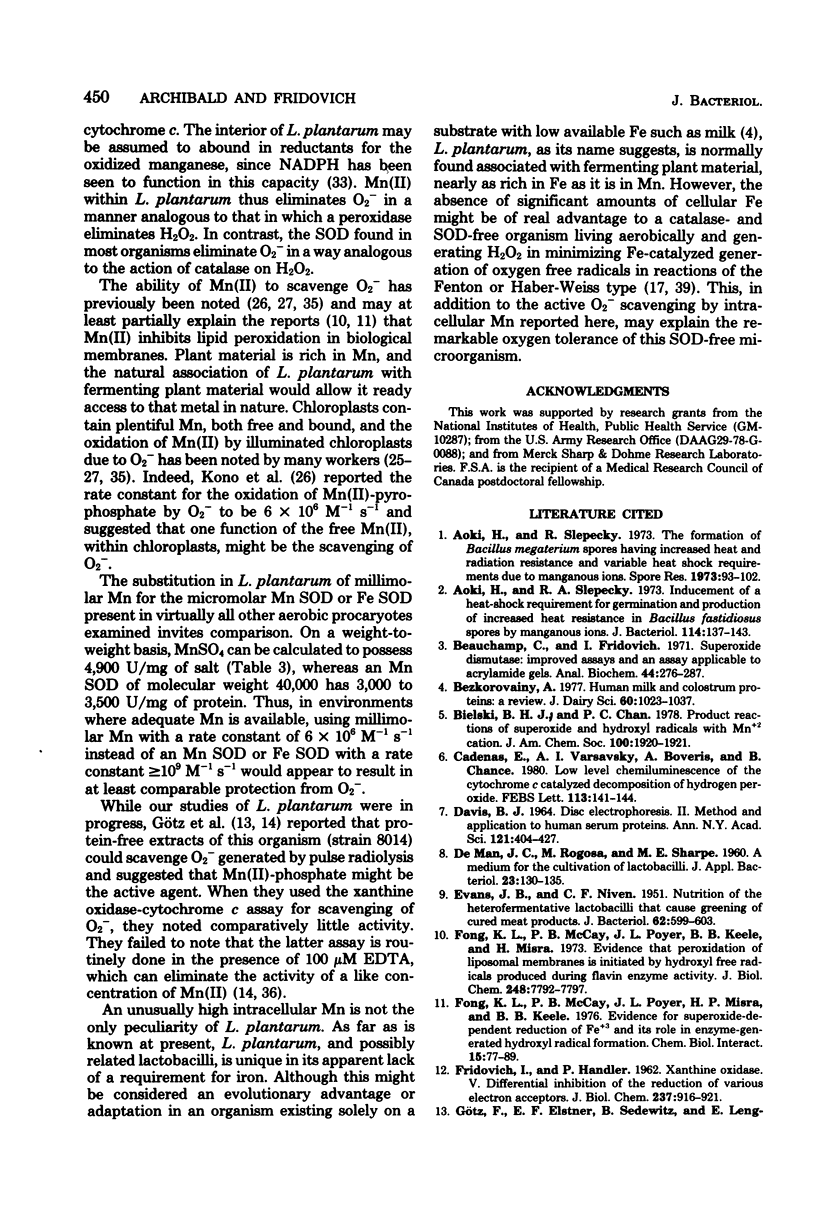
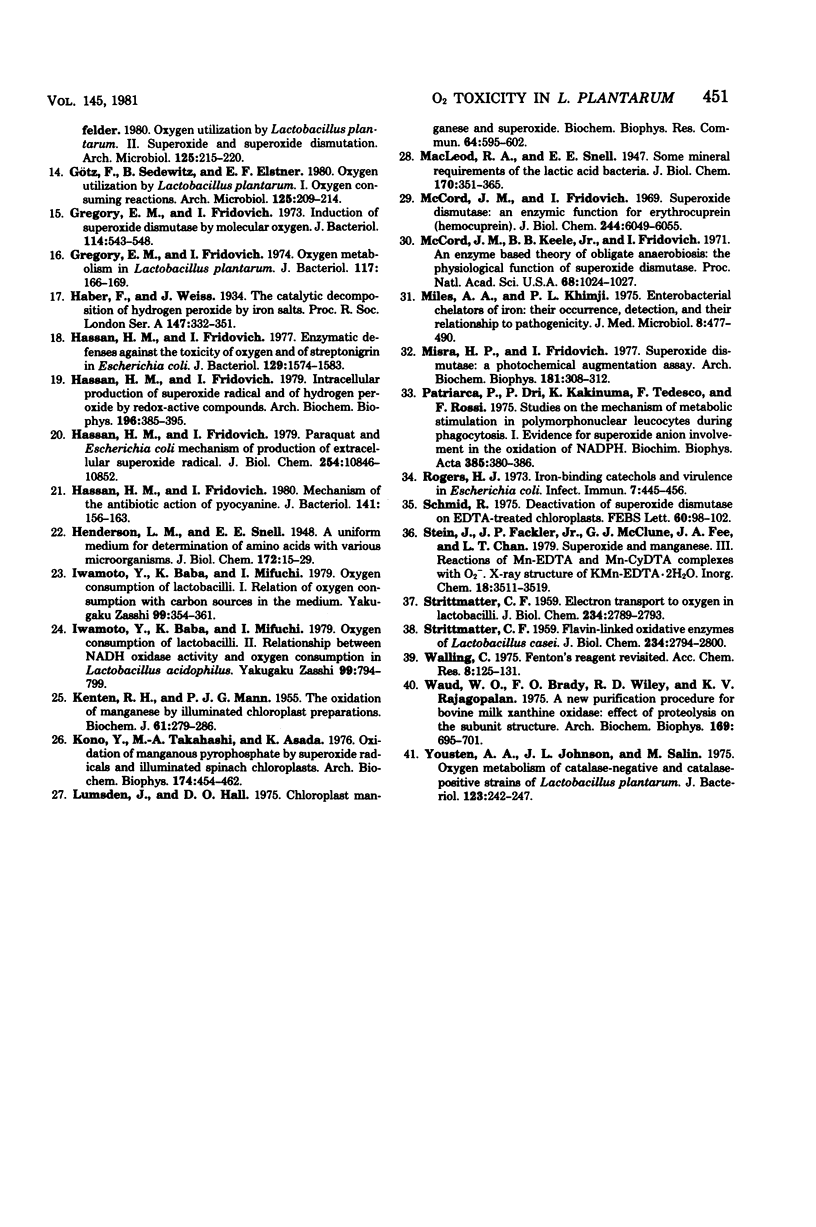
Selected References
These references are in PubMed. This may not be the complete list of references from this article.
- Aoki H., Slepecky R. A. Inducement of a heat-shock requirement for germination and production of increased heat resistance in Bacillus fastidiosus spores by manganous ions. J Bacteriol. 1973 Apr;114(1):137–143. doi: 10.1128/jb.114.1.137-143.1973. [DOI] [PMC free article] [PubMed] [Google Scholar]
- Beauchamp C., Fridovich I. Superoxide dismutase: improved assays and an assay applicable to acrylamide gels. Anal Biochem. 1971 Nov;44(1):276–287. doi: 10.1016/0003-2697(71)90370-8. [DOI] [PubMed] [Google Scholar]
- Bexkorovainy A. Human mild and colostrum proteins: a review. J Dairy Sci. 1977 Jul;60(7):1023–1037. doi: 10.3168/jds.s0022-0302(77)83984-2. [DOI] [PubMed] [Google Scholar]
- Cadenas E., Varsavsky A. I., Boveris A., Chance B. Low level chemiluminescence of the cytochrome c-catalyzed decomposition of hydrogen peroxide. FEBS Lett. 1980 May 5;113(2):141–144. doi: 10.1016/0014-5793(80)80577-1. [DOI] [PubMed] [Google Scholar]
- DAVIS B. J. DISC ELECTROPHORESIS. II. METHOD AND APPLICATION TO HUMAN SERUM PROTEINS. Ann N Y Acad Sci. 1964 Dec 28;121:404–427. doi: 10.1111/j.1749-6632.1964.tb14213.x. [DOI] [PubMed] [Google Scholar]
- EVANS J. B., NIVEN C. F., Jr Nutrition of the heterofermentative Lactobacilli that cause greening of cured meat products. J Bacteriol. 1951 Nov;62(5):599–603. doi: 10.1128/jb.62.5.599-603.1951. [DOI] [PMC free article] [PubMed] [Google Scholar]
- FRIDOVICH I., HANDLER P. Xanthine oxidase. V. Differential inhibition of the reduction of various electron acceptors. J Biol Chem. 1962 Mar;237:916–921. [PubMed] [Google Scholar]
- Fong K. L., McCay P. B., Poyer J. L. Evidence for superoxide-dependent reduction of Fe3+ and its role in enzyme-generated hydroxyl radical formation. Chem Biol Interact. 1976 Sep;15(1):77–89. doi: 10.1016/0009-2797(76)90130-7. [DOI] [PubMed] [Google Scholar]
- Fong K. L., McCay P. B., Poyer J. L., Keele B. B., Misra H. Evidence that peroxidation of lysosomal membranes is initiated by hydroxyl free radicals produced during flavin enzyme activity. J Biol Chem. 1973 Nov 25;248(22):7792–7797. [PubMed] [Google Scholar]
- Gregory E. M., Fridovich I. Induction of superoxide dismutase by molecular oxygen. J Bacteriol. 1973 May;114(2):543–548. doi: 10.1128/jb.114.2.543-548.1973. [DOI] [PMC free article] [PubMed] [Google Scholar]
- Gregory E. M., Fridovich I. Oxygen metabolism in Lactobacillus plantarum. J Bacteriol. 1974 Jan;117(1):166–169. doi: 10.1128/jb.117.1.166-169.1974. [DOI] [PMC free article] [PubMed] [Google Scholar]
- Götz F., Sedewitz B., Elstner E. F. Oxygen utilization by Lactobacillus plantarum. I. Oxygen consuming reactions. Arch Microbiol. 1980 Apr;125(3):209–214. doi: 10.1007/BF00446878. [DOI] [PubMed] [Google Scholar]
- Hassan H. M., Fridovich I. Enzymatic defenses against the toxicity of oxygen and of streptonigrin in Escherichia coli. J Bacteriol. 1977 Mar;129(3):1574–1583. doi: 10.1128/jb.129.3.1574-1583.1977. [DOI] [PMC free article] [PubMed] [Google Scholar]
- Hassan H. M., Fridovich I. Intracellular production of superoxide radical and of hydrogen peroxide by redox active compounds. Arch Biochem Biophys. 1979 Sep;196(2):385–395. doi: 10.1016/0003-9861(79)90289-3. [DOI] [PubMed] [Google Scholar]
- Hassan H. M., Fridovich I. Mechanism of the antibiotic action pyocyanine. J Bacteriol. 1980 Jan;141(1):156–163. doi: 10.1128/jb.141.1.156-163.1980. [DOI] [PMC free article] [PubMed] [Google Scholar]
- Hassan H. M., Fridovich I. Paraquat and Escherichia coli. Mechanism of production of extracellular superoxide radical. J Biol Chem. 1979 Nov 10;254(21):10846–10852. [PubMed] [Google Scholar]
- Iwamoto Y., Baba K., Mifuchi I. [Oxygen consumption of lactobacilli. I. Relation of oxygen consumption with carbon sources in the medium (author's transl)]. Yakugaku Zasshi. 1979 Apr;99(4):354–361. doi: 10.1248/yakushi1947.99.4_354. [DOI] [PubMed] [Google Scholar]
- Iwamoto Y., Baba K., Mifuchi I. [Oxygen consumption of lactobacilli. II. relationship between NADH oxidase activity and oxygen consumption of Lactobacillus acidophilus (author's transl)]. Yakugaku Zasshi. 1979 Aug;99(8):794–799. doi: 10.1248/yakushi1947.99.8_794. [DOI] [PubMed] [Google Scholar]
- KENTEN R. H., MANN P. J. The oxidation of manganese by illuminated chloroplast preparations. Biochem J. 1955 Oct;61(2):279–286. doi: 10.1042/bj0610279. [DOI] [PMC free article] [PubMed] [Google Scholar]
- Kono Y., Takahashi M. A., Asada K. Oxidation of manganous pyrophosphate by superoxide radicals and illuminated spinach chloroplasts. Arch Biochem Biophys. 1976 Jun;174(2):454–462. doi: 10.1016/0003-9861(76)90373-8. [DOI] [PubMed] [Google Scholar]
- Lumsden J., Hall D. O. Chloroplast manganese and superoxide. Biochem Biophys Res Commun. 1975 May 19;64(2):595–602. doi: 10.1016/0006-291x(75)90363-0. [DOI] [PubMed] [Google Scholar]
- McCord J. M., Fridovich I. Superoxide dismutase. An enzymic function for erythrocuprein (hemocuprein). J Biol Chem. 1969 Nov 25;244(22):6049–6055. [PubMed] [Google Scholar]
- McCord J. M., Keele B. B., Jr, Fridovich I. An enzyme-based theory of obligate anaerobiosis: the physiological function of superoxide dismutase. Proc Natl Acad Sci U S A. 1971 May;68(5):1024–1027. doi: 10.1073/pnas.68.5.1024. [DOI] [PMC free article] [PubMed] [Google Scholar]
- Miles A. A., Khimji P. L. Enterobacterial chelators of iron: their occurrence, detection, and relation to pathogenicity. J Med Microbiol. 1975 Nov;8(4):477–490. doi: 10.1099/00222615-8-4-477. [DOI] [PubMed] [Google Scholar]
- Misra H. P., Fridovich I. Superoxide dismutase: a photochemical augmentation assay. Arch Biochem Biophys. 1977 May;181(1):308–312. doi: 10.1016/0003-9861(77)90509-4. [DOI] [PubMed] [Google Scholar]
- Patriarca P., Dri P., Kakinuma K., Tedesco F., Rossi F. Studies on the mechanism of metabolic stimulation in polymorphonuclear leucocytes during phagocytosis. I. Evidence for superoxide anion involvement in the oxidation of NADPH2. Biochim Biophys Acta. 1975 Apr 7;385(2):380–386. doi: 10.1016/0304-4165(75)90367-0. [DOI] [PubMed] [Google Scholar]
- Rogers H. J. Iron-Binding Catechols and Virulence in Escherichia coli. Infect Immun. 1973 Mar;7(3):445–456. doi: 10.1128/iai.7.3.445-456.1973. [DOI] [PMC free article] [PubMed] [Google Scholar]
- STRITTMATTER C. F. Flavin-linked oxidative enzymes of Lactobacillus casei. J Biol Chem. 1959 Oct;234:2794–2800. [PubMed] [Google Scholar]
- STRITTMATTTER C. F. Electron transport to oxygen in lactobacilli. J Biol Chem. 1959 Oct;234:2789–2793. [PubMed] [Google Scholar]
- Waud W. R., Brady F. O., Wiley R. D., Rajagopalan K. V. A new purification procedure for bovine milk xanthine oxidase: effect of proteolysis on the subunit structure. Arch Biochem Biophys. 1975 Aug;169(2):695–701. doi: 10.1016/0003-9861(75)90214-3. [DOI] [PubMed] [Google Scholar]
- Yousten A. A., Johnson J. L., Salin M. Oxygen metabolism of catalase-negative and catalase-positive strains of Lactobacillus plantarum. J Bacteriol. 1975 Jul;123(1):242–247. doi: 10.1128/jb.123.1.242-247.1975. [DOI] [PMC free article] [PubMed] [Google Scholar]