Abstract
Amino acids produced from protein degradation are the major energy source for differentiation and aging in Dictyostelium discoideum. Considering the reactions involved in the conversion of amino acids from an average protein into tricarboxylic acid cycle intermediates, a route from a cycle intermediate (probably malate) to acetyl coenzyme A is required for the complete utilization of amino acids. Citrate was isolated from cells pulse-labeled with 14C-labeled amino acids and was cleaved with citrate lyase. When cells were pulse-labeled with [U-14C]-glutamate the specific radioactivity of the acetate and oxaloacetate portions of citrate were consistent with the conclusion that one-third of the carbon flowing through the tricarboxylic acid cycle is removed for the synthesis of acetyl coenzyme A. The data were also consistent with the patterns of carbon flux required to maintain steady-state levels of cycle intermediates in cells catabolizing amino acids. It is suggested that the malic enzyme (EC 1.1.1.40) catalyzes the synthesis of acetyl coenzyme A from malate and is responsible for the observed citrate labeling pattern. In cell extracts the activity of this enzyme increased markedly with the onset of differentiation. The properties of partially purified (40-fold) malic enzyme isolated at culmination indicated that the enzyme was allosteric and was positively affected by aspartate and glutamate. Thus, amino acid production from protein degradation would stimulate a reaction essential for the efficient utilization of these amino acids for energy.
Full text
PDF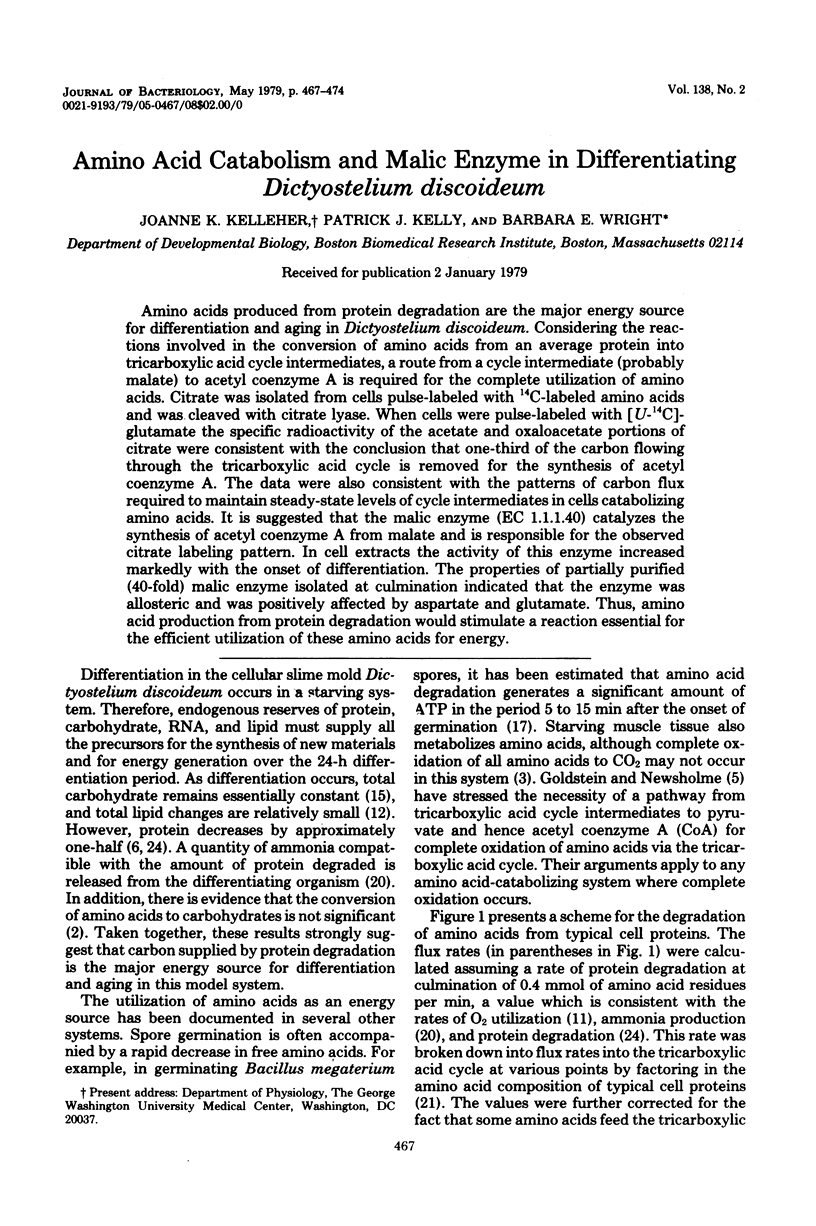
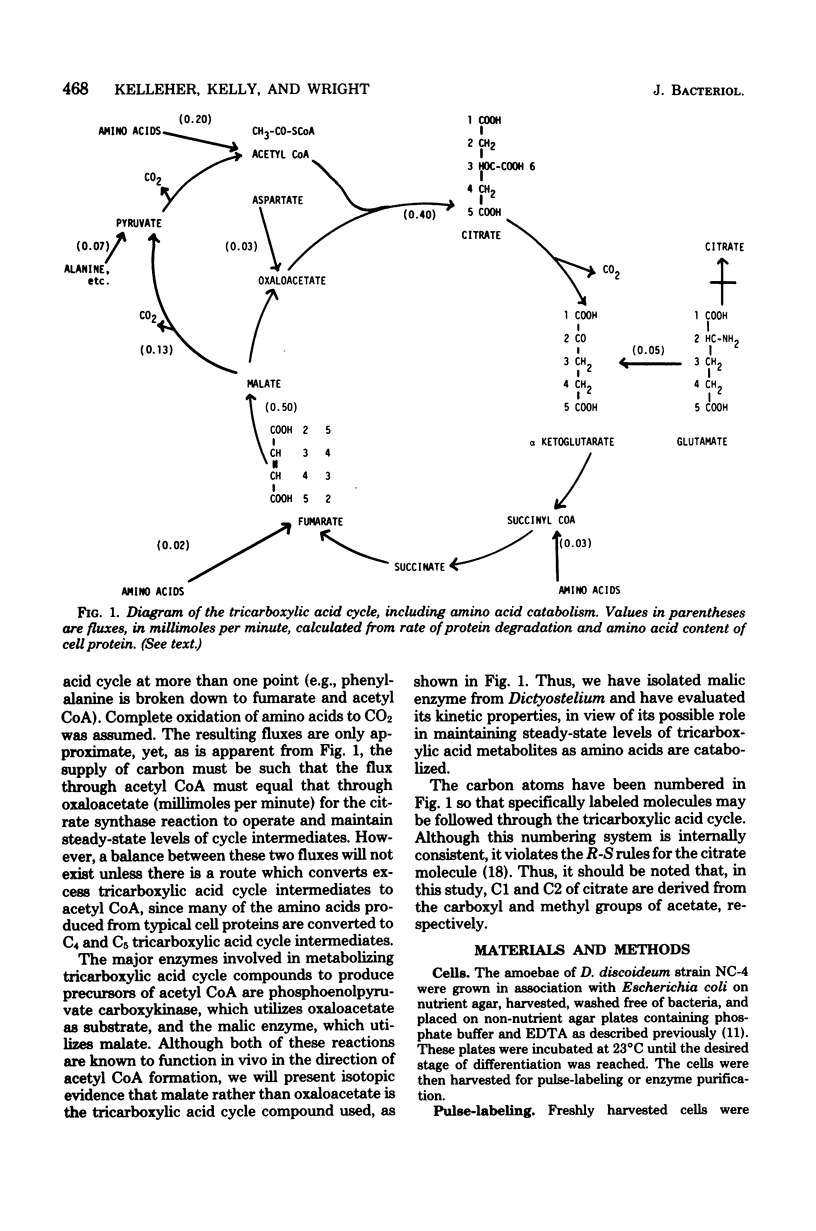
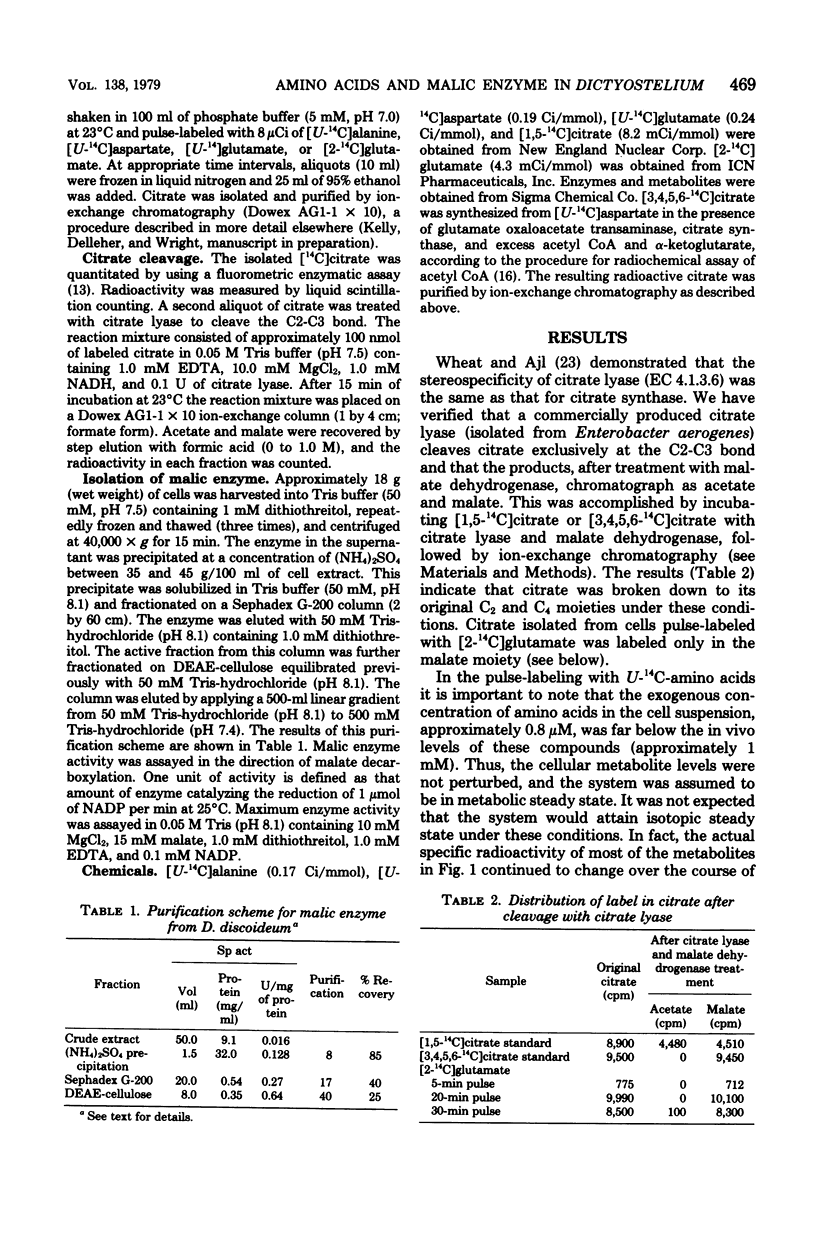
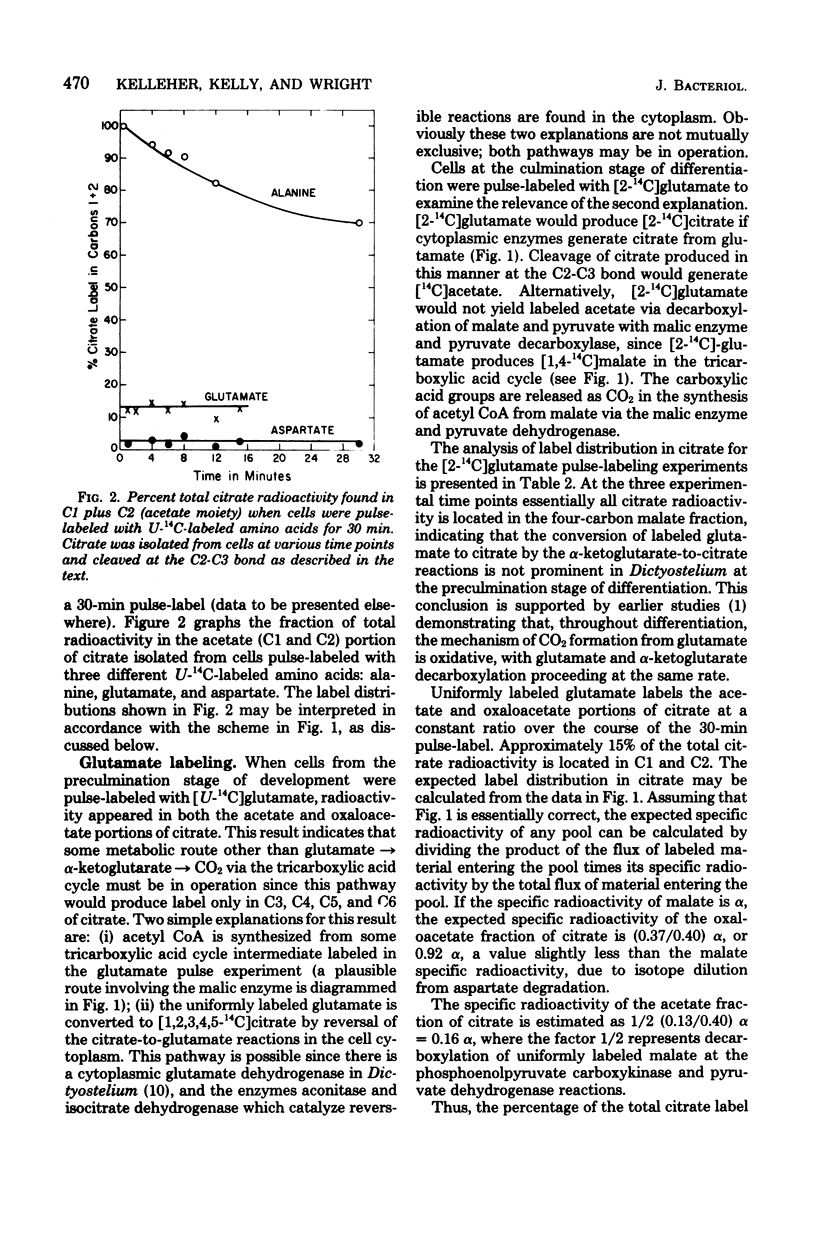
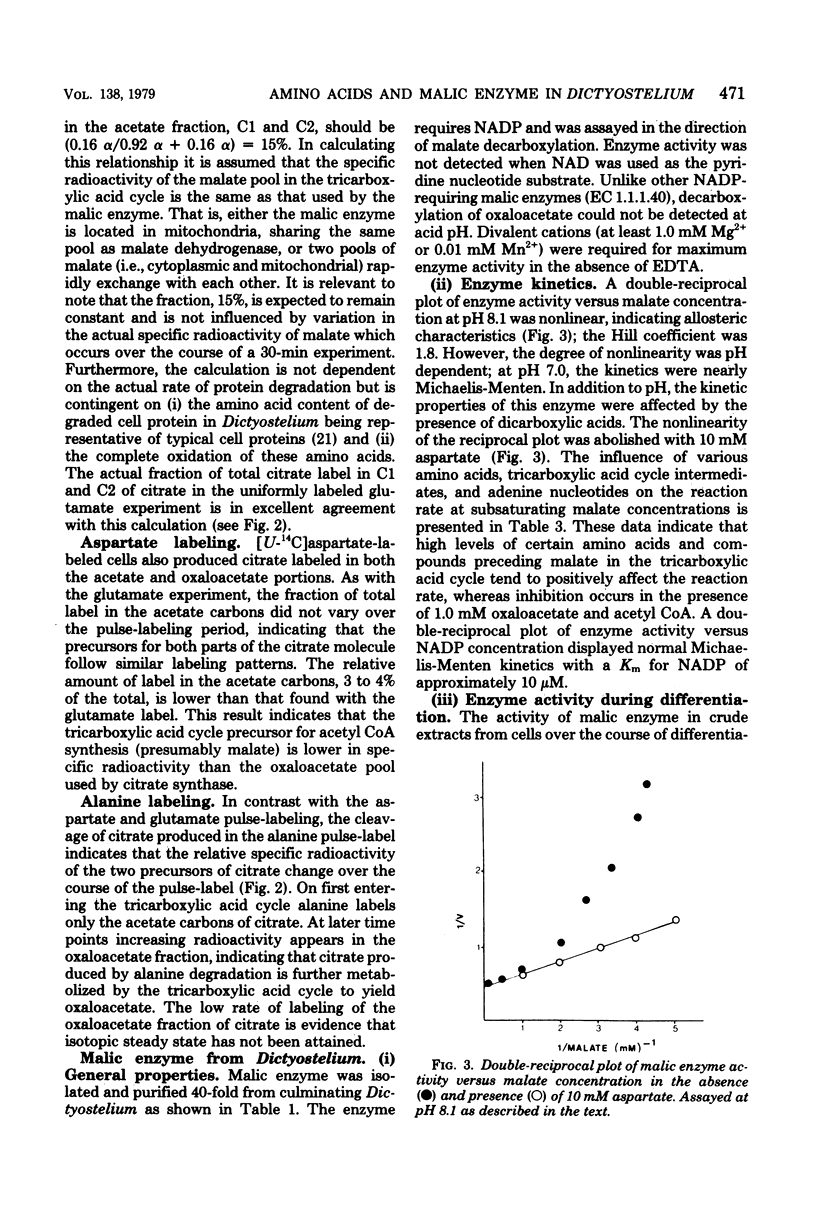
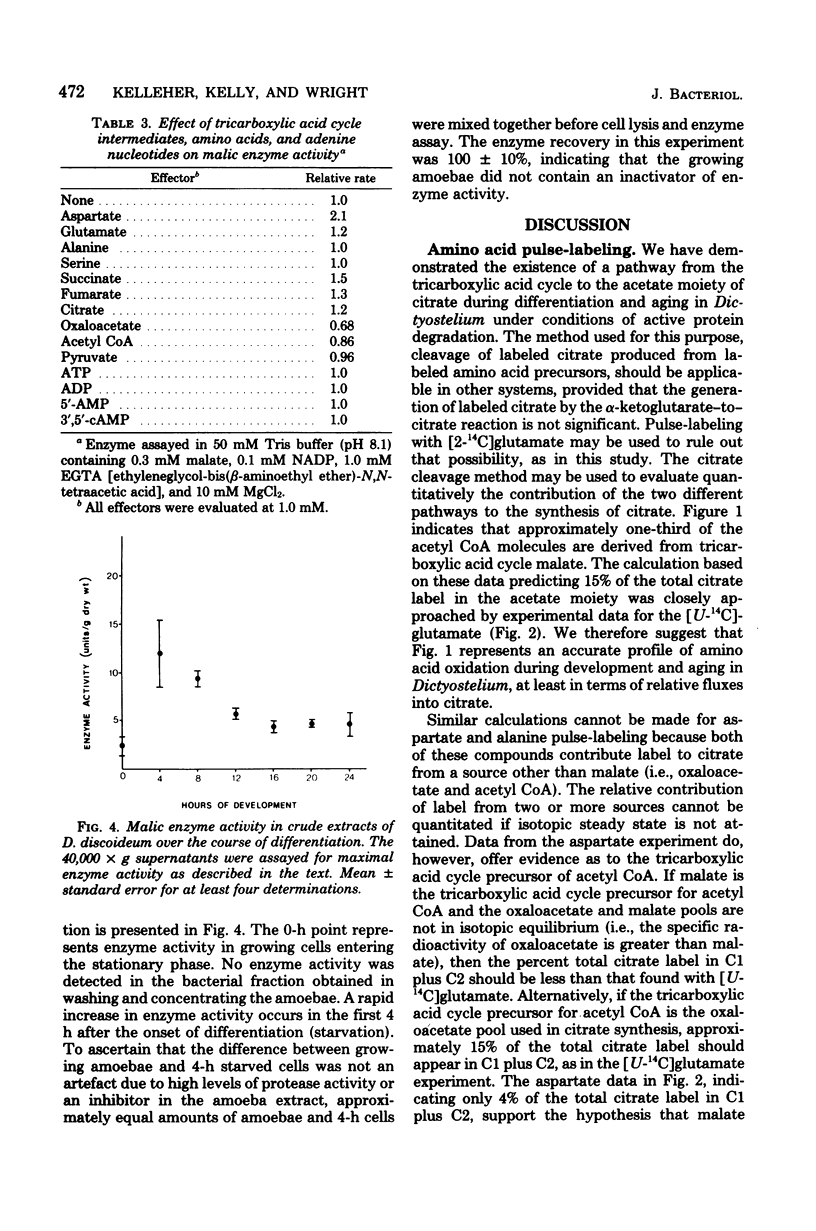
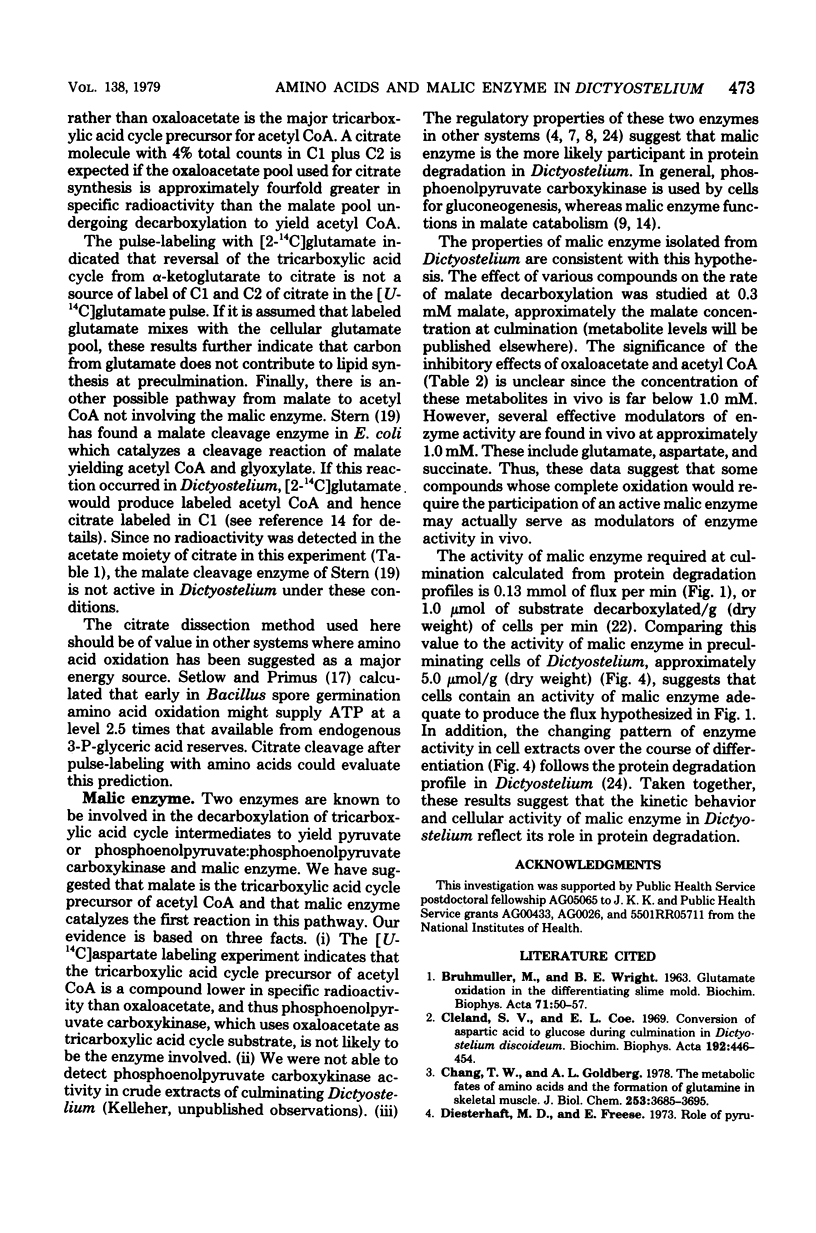
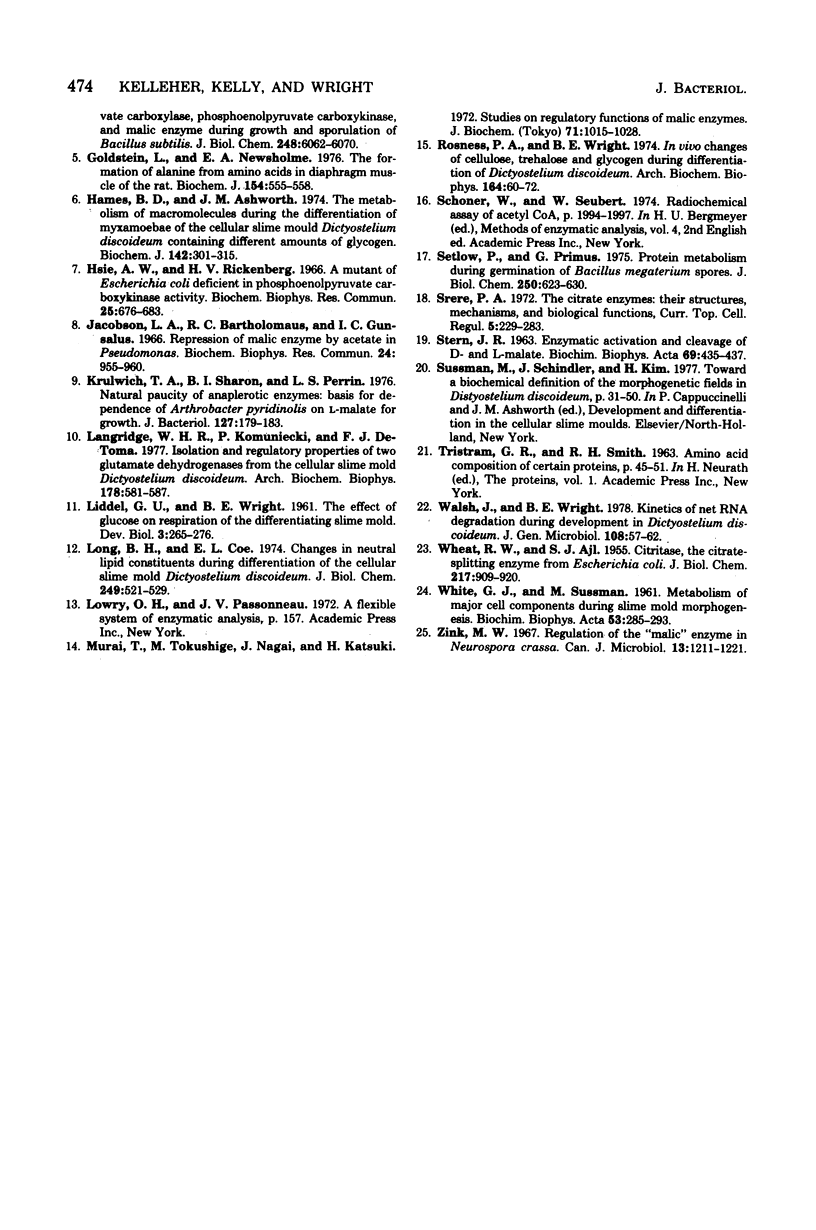
Selected References
These references are in PubMed. This may not be the complete list of references from this article.
- BRUEHMUELLER M., WRIGHT B. E. Glutamate oxidation in the differentiating slime mold. II. Studies in vitro. Biochim Biophys Acta. 1963 Apr 2;71:50–57. doi: 10.1016/0006-3002(63)90984-3. [DOI] [PubMed] [Google Scholar]
- Chang T. W., Goldberg A. L. The metabolic fates of amino acids and the formation of glutamine in skeletal muscle. J Biol Chem. 1978 May 25;253(10):3685–3693. [PubMed] [Google Scholar]
- Cleland S. V., Coe E. L. Conversion of aspartic acid to glucose during culmination of Dictyostelium discoideum. Biochim Biophys Acta. 1969 Dec 30;192(3):446–454. doi: 10.1016/0304-4165(69)90393-6. [DOI] [PubMed] [Google Scholar]
- Goldstein L., Newsholme E. A. The formation of alanine from amino acids in diaphragm muscle of the rat. Biochem J. 1976 Feb 15;154(2):555–558. doi: 10.1042/bj1540555. [DOI] [PMC free article] [PubMed] [Google Scholar]
- Hames B. D., Ashworth J. M. The metabolism of macromolecules during the differentiation of Myxamoebae of the cellular slime mould Dictyostelium discoideum containing different amounts of glycogen. Biochem J. 1974 Aug;142(2):301–315. doi: 10.1042/bj1420301. [DOI] [PMC free article] [PubMed] [Google Scholar]
- Jacobson L. A., Bartholomaus R. C., Gunsalus I. C. Repression of malic enzyme by acetate in Pseudomonas. Biochem Biophys Res Commun. 1966 Sep 22;24(6):955–960. doi: 10.1016/0006-291x(66)90343-3. [DOI] [PubMed] [Google Scholar]
- Krulwich T. A., Sharon B. I., Perrin L. S. Natural paucity of anaplerotic enzymes: basis for dependence of Arthrobacter pyridinolis on L-malate for growth. J Bacteriol. 1976 Jul;127(1):179–183. doi: 10.1128/jb.127.1.179-183.1976. [DOI] [PMC free article] [PubMed] [Google Scholar]
- LIDDEL G. U., WRIGHT B. E. The effect of glucose on respiration of the differentiating slime mold. Dev Biol. 1961 Jun;3:265–276. doi: 10.1016/0012-1606(61)90047-1. [DOI] [PubMed] [Google Scholar]
- Long B. H., Coe E. L. Changes in neutral lipid constituents during differentiation of the cellular slime mold, Dictyostelium discoideum. J Biol Chem. 1974 Jan 25;249(2):521–529. [PubMed] [Google Scholar]
- Rosness P. A., Wright B. E. In vivo changes of cellulose, trehalose and glycogen during differentiation of Dictyostelium discoideum. Arch Biochem Biophys. 1974 Sep;164(1):60–72. doi: 10.1016/0003-9861(74)90008-3. [DOI] [PubMed] [Google Scholar]
- STERN J. R. Enzymic activation and cleavage of D- and L-malate. Biochim Biophys Acta. 1963 Feb 5;69:435–438. doi: 10.1016/0006-3002(63)91288-5. [DOI] [PubMed] [Google Scholar]
- Setlow P., Primus G. Protein metabolism during germination of Bacillus megaterium spores. I. Protein synthesis and amino acid metabolism. J Biol Chem. 1975 Jan 25;250(2):623–630. [PubMed] [Google Scholar]
- Srere P. A. The citrate enzymes: their structures, mechanisms, and biological functions. Curr Top Cell Regul. 1972;5:229–283. doi: 10.1016/b978-0-12-152805-8.50013-7. [DOI] [PubMed] [Google Scholar]
- WHEAT R. W., AJL S. J. Citritase, the citrate-splitting enzyme from Escherichia coli. II. Reaction mechanisms. J Biol Chem. 1955 Dec;217(2):909–920. [PubMed] [Google Scholar]
- WHITE G. J., SUSSMAN M. Metabolism of major cell components during slime mold morphogenesis. Biochim Biophys Acta. 1961 Oct 28;53:285–293. doi: 10.1016/0006-3002(61)90441-3. [DOI] [PubMed] [Google Scholar]
- Walsh J., Wright B. E. Kinetics of net RNA degradation during development in Dictyostelium discoideum. J Gen Microbiol. 1978 Sep;108(1):57–62. doi: 10.1099/00221287-108-1-57. [DOI] [PubMed] [Google Scholar]
- Zink M. W. Regulation of the "malic" enzyme in Neurospora crassa. Can J Microbiol. 1967 Sep;13(9):1211–1221. doi: 10.1139/m67-166. [DOI] [PubMed] [Google Scholar]