Abstract
The role of fatty aldehyde dehydrogenases (FALDHs) in hexadecane and hexadecanol metabolism was studied in Acinetobacter sp. strain HO1-N. Two distinct FALDHs were demonstrated in Acinetobacter sp. strain HO1-N: a membrane-bound, NADP-dependent FALDH activity induced 5-, 15-, and 9-fold by growth on hexadecanol, dodecyl aldehyde, and hexadecane, respectively, and a constitutive, NAD-dependent, membrane-localized FALDH. The NADP-dependent FALDH exhibited apparent Km and Vmax values for decyl aldehyde of 5.0, 13.0, 18.0, and 18.3 microM and 537.0, 500.0, 25.0, and 38.0 nmol/min in hexadecane-, hexadecanol-, ethanol-, palmitate-grown cells, respectively. FALDH isozymes ald-a, ald-b, and ald-c were demonstrated by gel electrophoresis in extracts of hexadecane- and hexadecanol-grown cells. ald-a, ald-b, and ald-d were present in dodecyl aldehyde-grown cells, while palmitate-grown control cells contained ald-b and ald-d. Dodecyl aldehyde-negative mutants were isolated and grouped into two phenotypic classes based on growth: class 1 mutants were hexadecane and hexadecanol negative and class 2 mutants were hexadecane and hexadecanol positive. Specific activity of NADP-dependent FALDH in Ald21 (class 1 mutant) was 85% lower than that of wild-type FALDH, while the specific activity of Ald24 (class 2 mutant) was 55% greater than that of wild-type FALDH. Ald21R, a dodecyl aldehyde-positive revertant able to grow on hexadecane, hexadecanol, and dodecyl aldehyde, exhibited a 100% increase in the specific activity of the NADP-dependent FALDH. The oxidation of [3H]hexadecane byAld21 yielded the accumulation of 61% more fatty aldehyde than the wild type, while Ald24 accumulated 27% more fatty aldehyde, 95% more fatty alcohol, and 65% more wax ester than the wild type. This study provides genetic and physiological evidence for the role of fatty aldehyde as an essential metabolic intermediate and NADP-dependent FALDH as a key enzyme in the dissimilation of hexadecane, hexadecanol, and dodecyl aldehyde in Acinetobactor sp. strain HO1-N.
Full text
PDF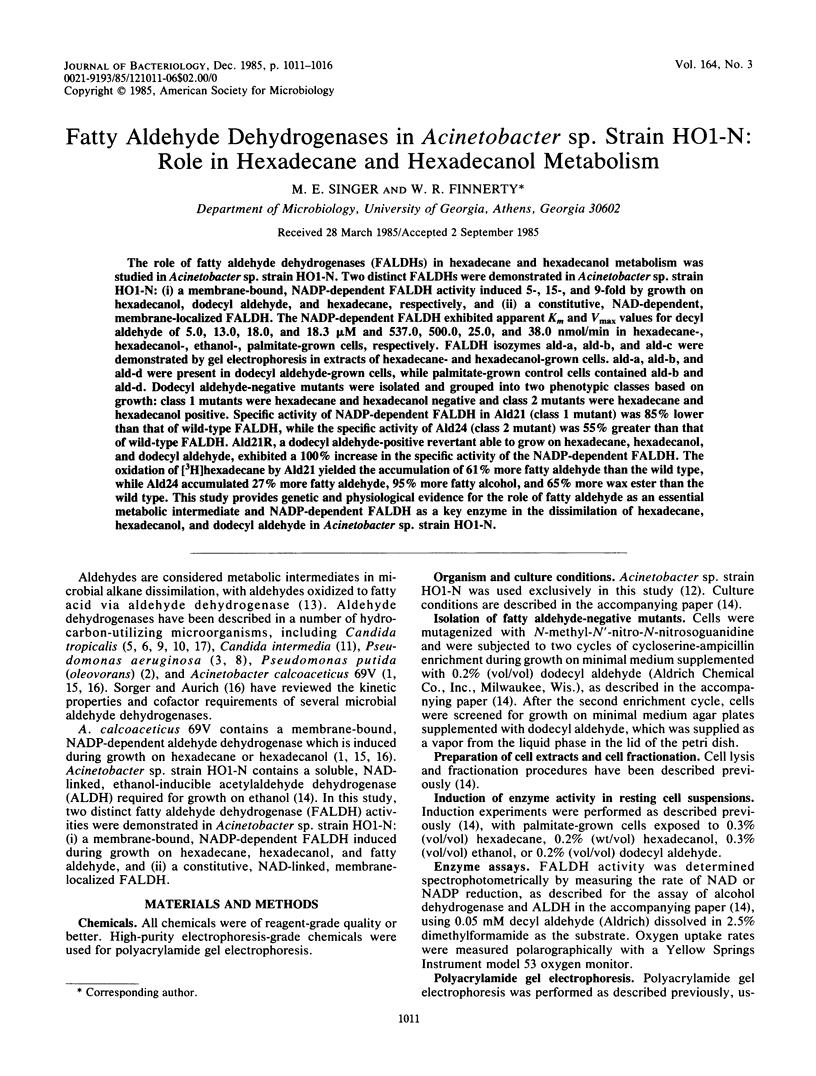
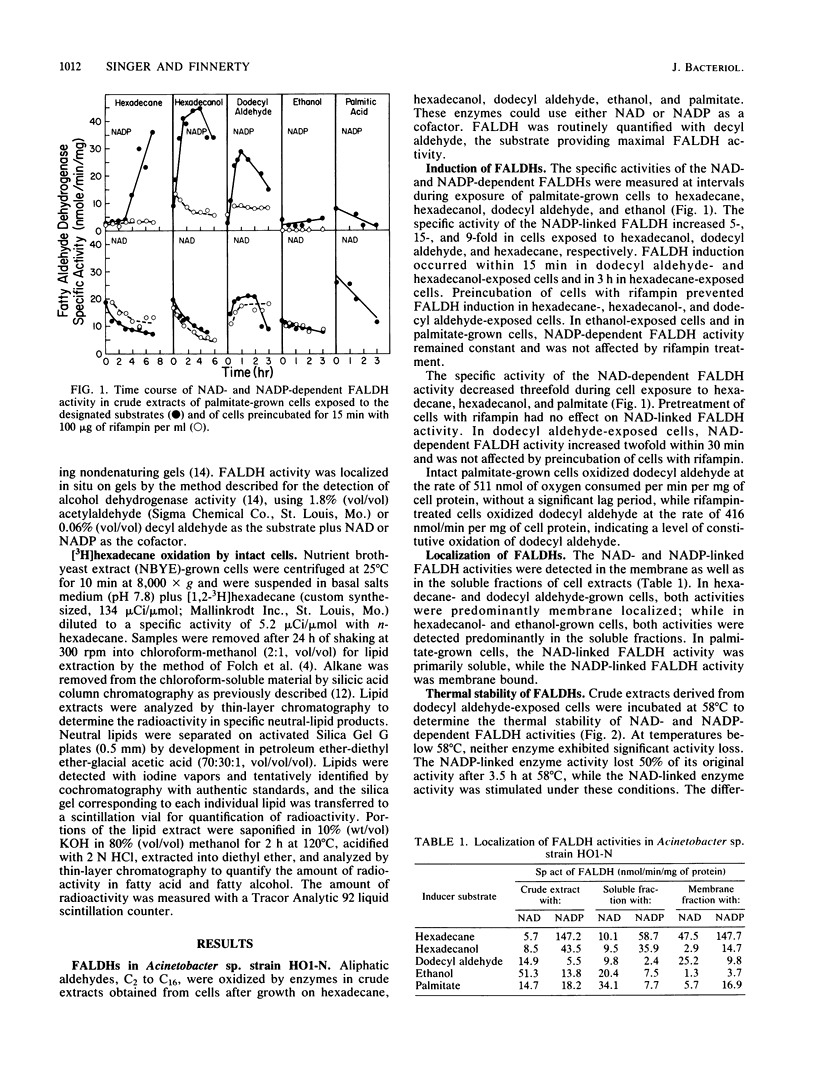
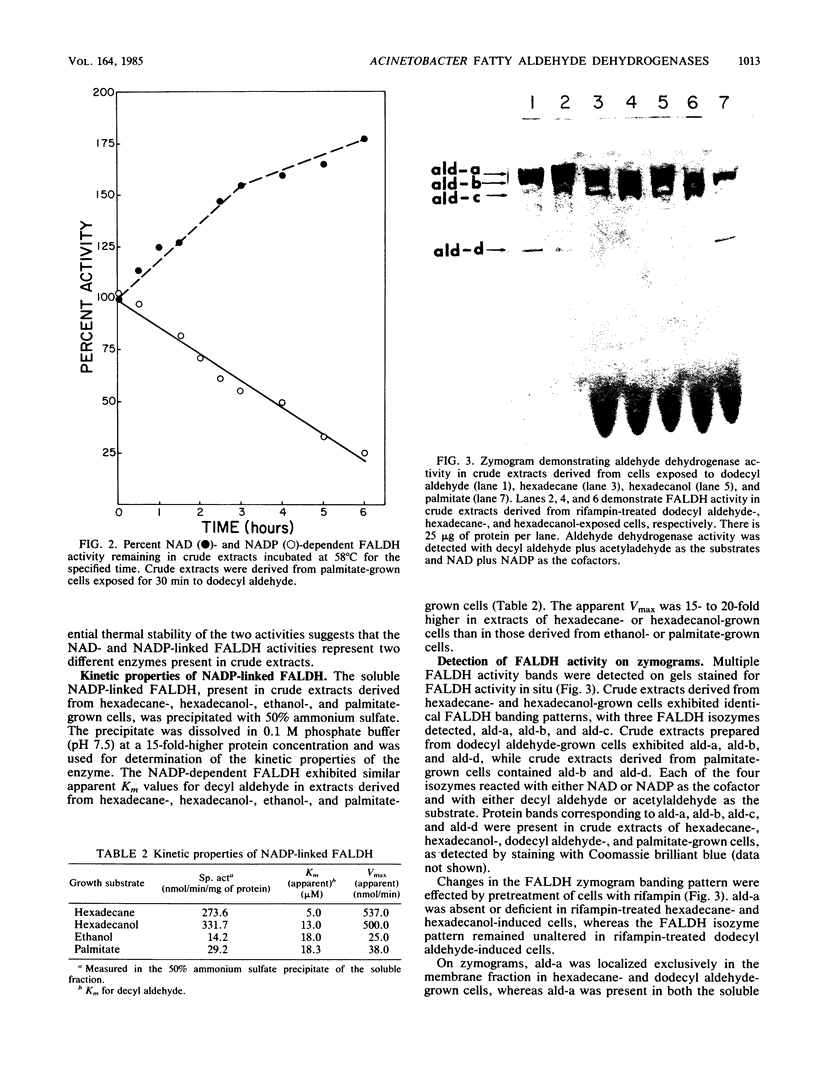
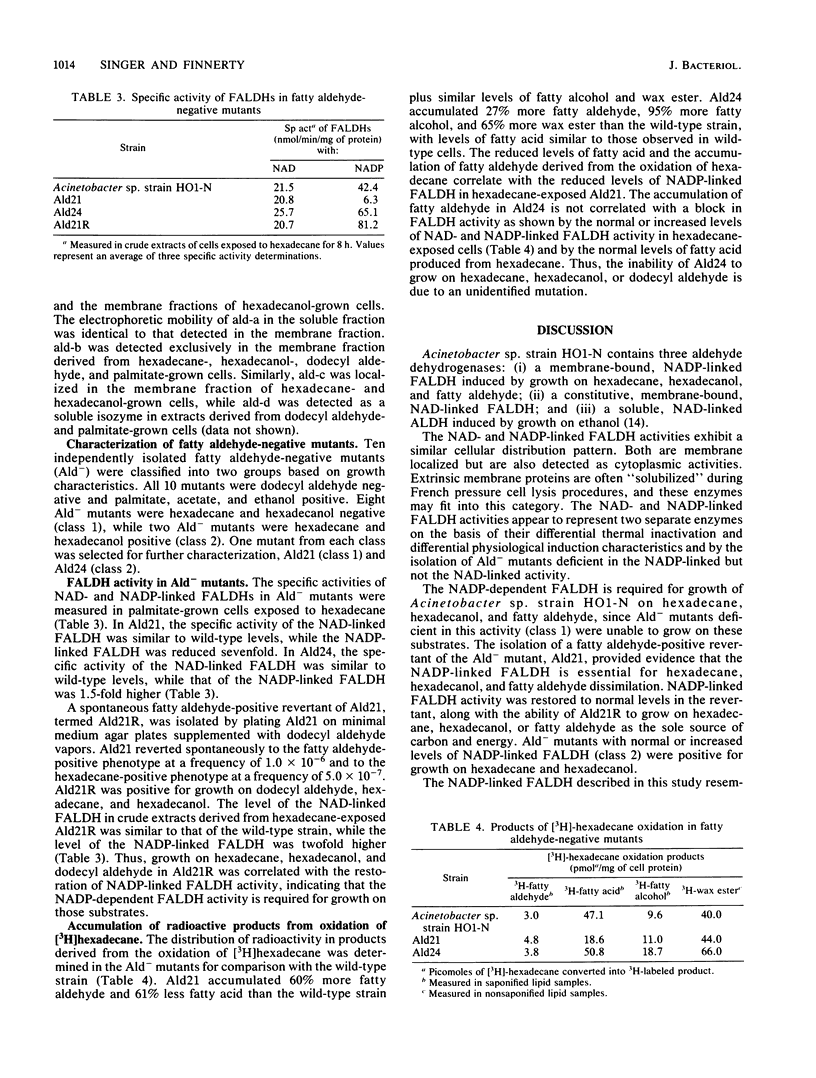
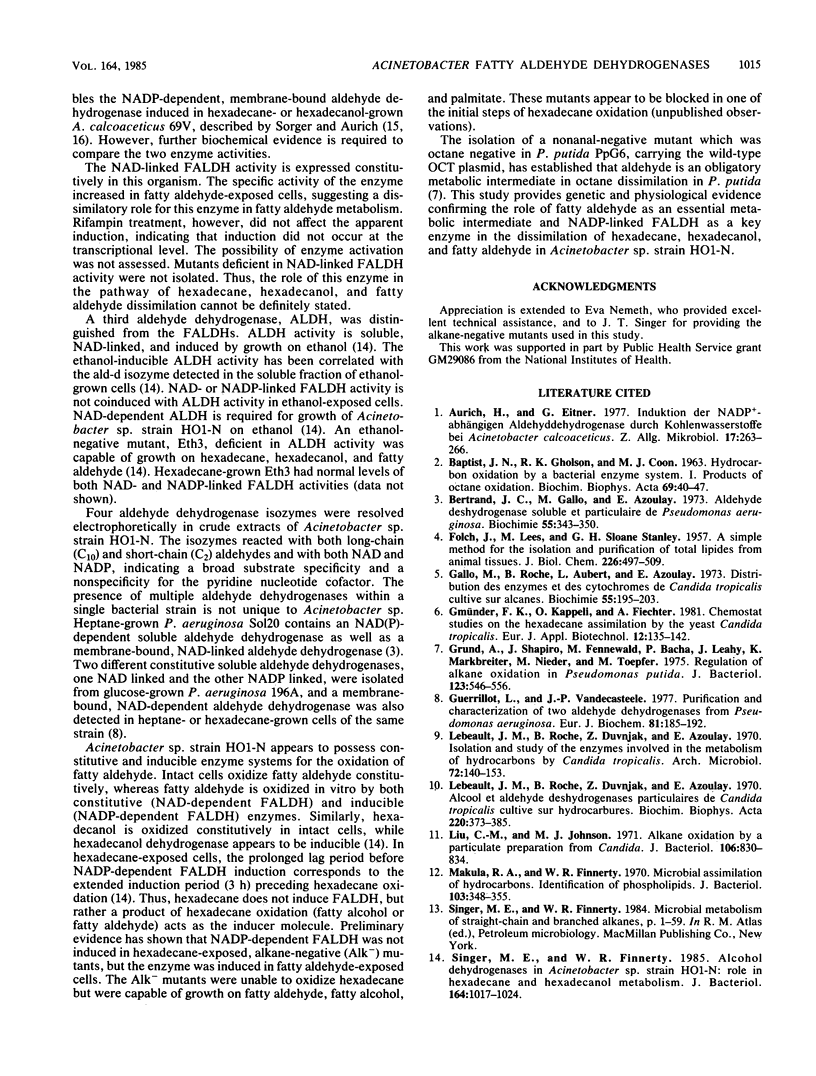
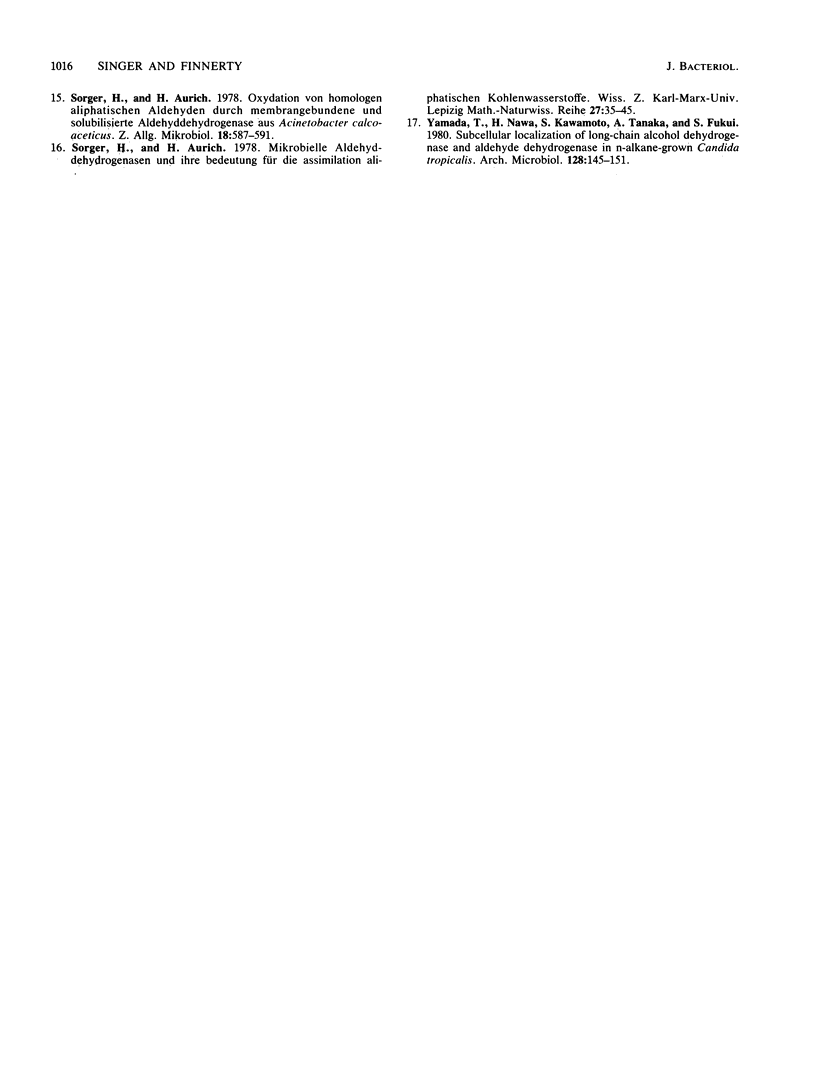
Images in this article
Selected References
These references are in PubMed. This may not be the complete list of references from this article.
- Aurich H., Eitner G. Induktion der NADP+-abhängigen Aldehyddehydrogenase durch Kohlenwasserstoffe bei Acinetobacter calcoaceticus. Z Allg Mikrobiol. 1977;17(4):263–266. doi: 10.1002/jobm.3630170402. [DOI] [PubMed] [Google Scholar]
- BAPTIST J. N., GHOLSON R. K., COON M. J. Hydrocarbon oxidation by a bacterial enzyme system. I. Products of octane oxidation. Biochim Biophys Acta. 1963 Jan 1;69:40–47. doi: 10.1016/0006-3002(63)91223-x. [DOI] [PubMed] [Google Scholar]
- Bertrand J. C., Gallo M., Azoulay E. Aldéhyde déhydrogénases soluble et particulaire de Pseudomonas aeruginosa. Biochimie. 1973;55(3):343–350. doi: 10.1016/s0300-9084(73)80135-x. [DOI] [PubMed] [Google Scholar]
- FOLCH J., LEES M., SLOANE STANLEY G. H. A simple method for the isolation and purification of total lipides from animal tissues. J Biol Chem. 1957 May;226(1):497–509. [PubMed] [Google Scholar]
- Gallo M., Roche B., Aubert L., Azoulay E. Distribution des enzymes et des cytochromes do Candida tropicalis cultivé sur aleanes. Biochimie. 1973;55(2):195–203. doi: 10.1016/s0300-9084(73)80392-x. [DOI] [PubMed] [Google Scholar]
- Grund A., Shapiro J., Fennewald M., Bacha P., Leahy J., Markbreiter K., Nieder M., Toepfer M. Regulation of alkane oxidation in Pseudomonas putida. J Bacteriol. 1975 Aug;123(2):546–556. doi: 10.1128/jb.123.2.546-556.1975. [DOI] [PMC free article] [PubMed] [Google Scholar]
- Guerrillot L., Vandecasteele J. P. Purification and characterization of two aldehyde dehydrogenases from Pseudomonas aeruginosa. Eur J Biochem. 1977 Nov 15;81(1):185–192. doi: 10.1111/j.1432-1033.1977.tb11940.x. [DOI] [PubMed] [Google Scholar]
- Lebeault J. M., Roche B., Duvnjak Z., Azoulay E. Alcool-et aldéhyde-déshydrogénases particulaires de Candida tropicalis cultivé sur hydrocarbures. Biochim Biophys Acta. 1970 Dec 16;220(3):373–385. doi: 10.1016/0005-2744(70)90269-x. [DOI] [PubMed] [Google Scholar]
- Lebeault J. M., Roche B., Duvnjak Z., Azoulay E. Isolation and study of the enzymes involved in the metabolism of hydrocarbons by Candida tropicalis. Arch Mikrobiol. 1970;72(2):140–153. doi: 10.1007/BF00409520. [DOI] [PubMed] [Google Scholar]
- Liu C. M., Johnson M. J. Alkane oxidation by a particulate preparation from Candida. J Bacteriol. 1971 Jun;106(3):830–834. doi: 10.1128/jb.106.3.830-834.1971. [DOI] [PMC free article] [PubMed] [Google Scholar]
- Makula R. A., Finnerty W. R. Microbial assimilation of hydrocarbons: identification of phospholipids. J Bacteriol. 1970 Aug;103(2):348–355. doi: 10.1128/jb.103.2.348-355.1970. [DOI] [PMC free article] [PubMed] [Google Scholar]
- Singer M. E., Finnerty W. R. Alcohol dehydrogenases in Acinetobacter sp. strain HO1-N: role in hexadecane and hexadecanol metabolism. J Bacteriol. 1985 Dec;164(3):1017–1024. doi: 10.1128/jb.164.3.1017-1024.1985. [DOI] [PMC free article] [PubMed] [Google Scholar]
- Sorger H., Aurich H. Oxydation von homologen aliphatischen Aldehyden durch membrangebundene und solubilisierte Aldehyddehydrogenase aus Acinetobacter calcoaceticus. Z Allg Mikrobiol. 1978;18(8):587–591. doi: 10.1002/jobm.3630180806. [DOI] [PubMed] [Google Scholar]
- Yamada T., Nawa H., Kawamoto S., Tanaka A., Fukui S. Subcellular localization of long-chain alcohol dehydrogenase and aldehyde dehydrogenase in n-alkane-grown Candida tropicalis. Arch Microbiol. 1980 Dec;128(2):145–151. doi: 10.1007/BF00406151. [DOI] [PubMed] [Google Scholar]