Abstract
Transport of 2-deoxyglucose or glucose in Streptococcus agalactiae was strongly inhibited if the cells were first exposed to a combination of lactoperoxidase-thiocyanate-hydrogen peroxide (LP-complex). The inhibition was completely reversible with dithiothreitol. N-ethylmaleimide and p-chloromercuribenzoate inhibited sugar transport, and the inhibition was also reversible with dithiothreitol. Sodium fluoride also inhibited sugar transport. Glucolysis was completely inhibited, and dithiothreitol completely reversed the inhibition. Phosphoenolpyruvate-dependent phosphotransferase activity in S. agalactiae was not strongly inhibited by the LP-complex. Interference of the entry of glucose into cells of S. agalactiae by the LP-complex could well account for its growth inhibitory properties with this organism. The inhibition of glucose transport by the LP-complex and its reversibility with dithiothreitol suggest the modification of functional sulfhydryl groups in the cell membrane as a cause of transport inhibition.
Full text
PDF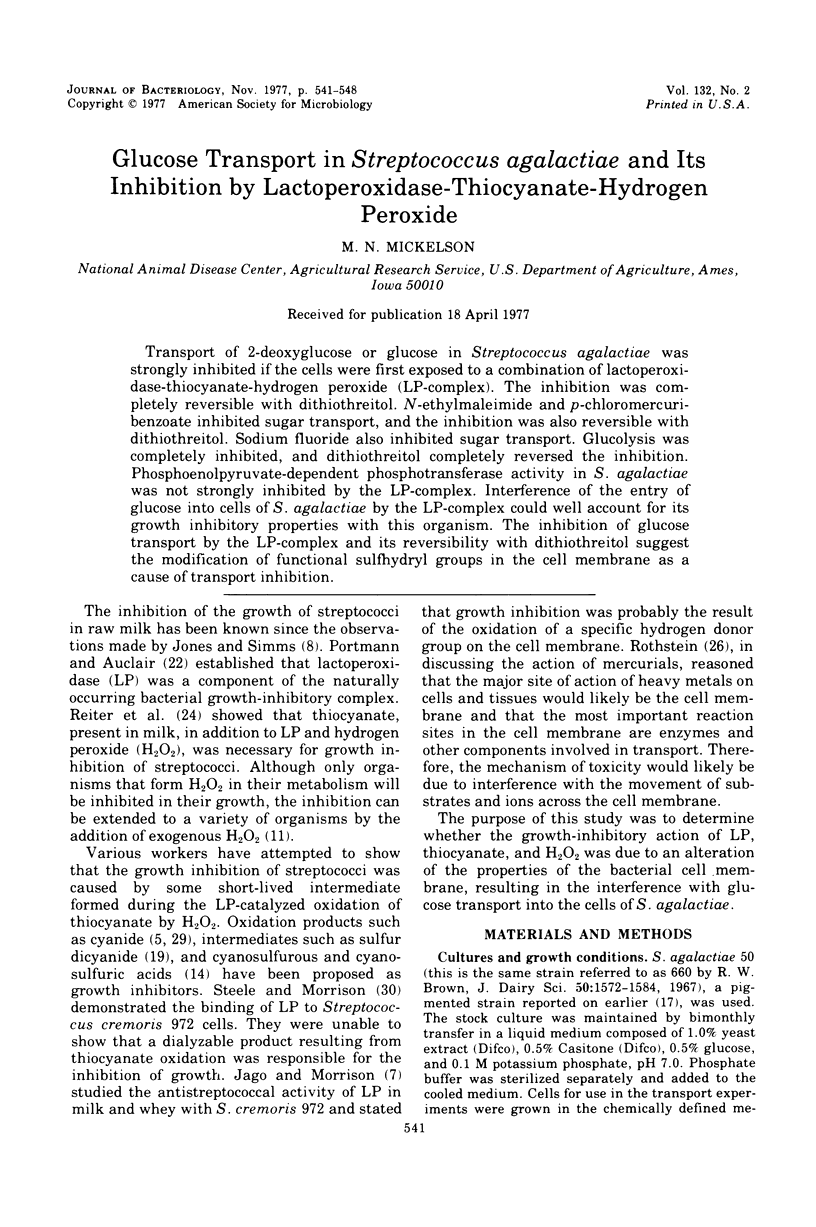
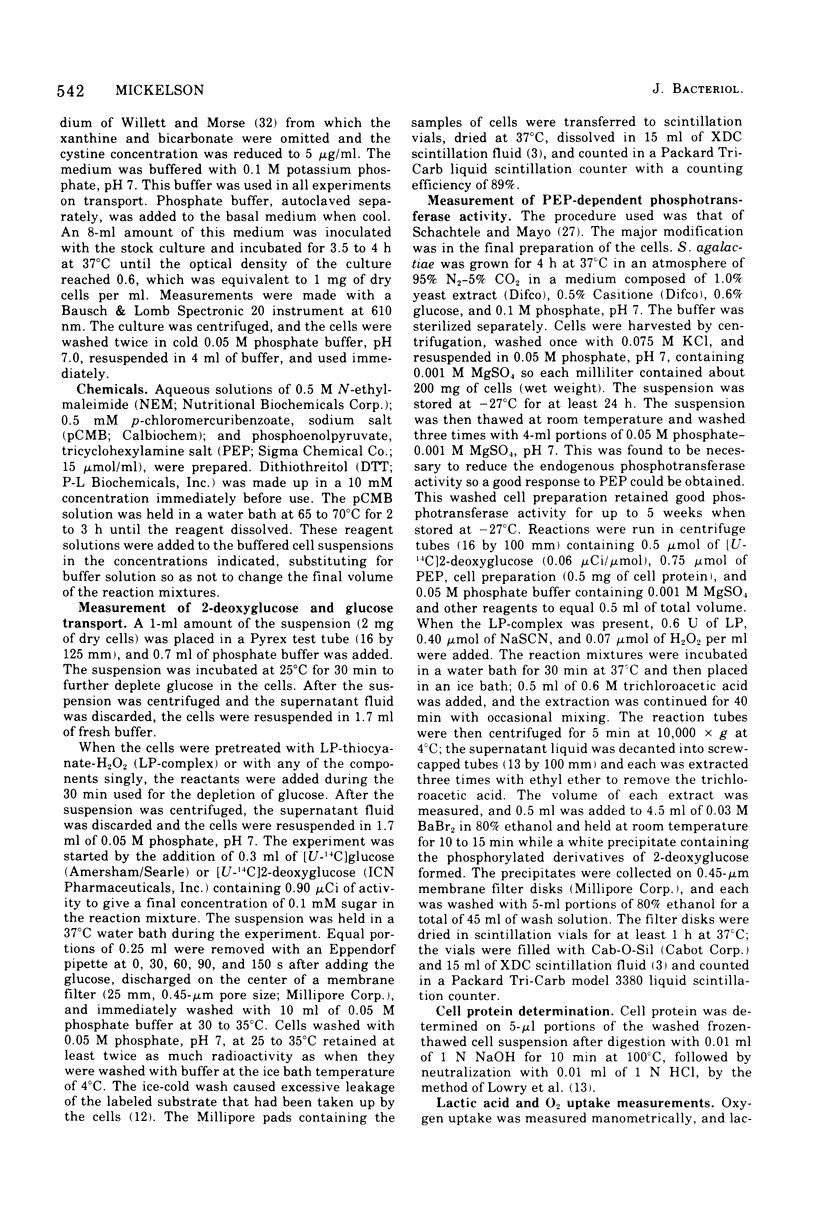
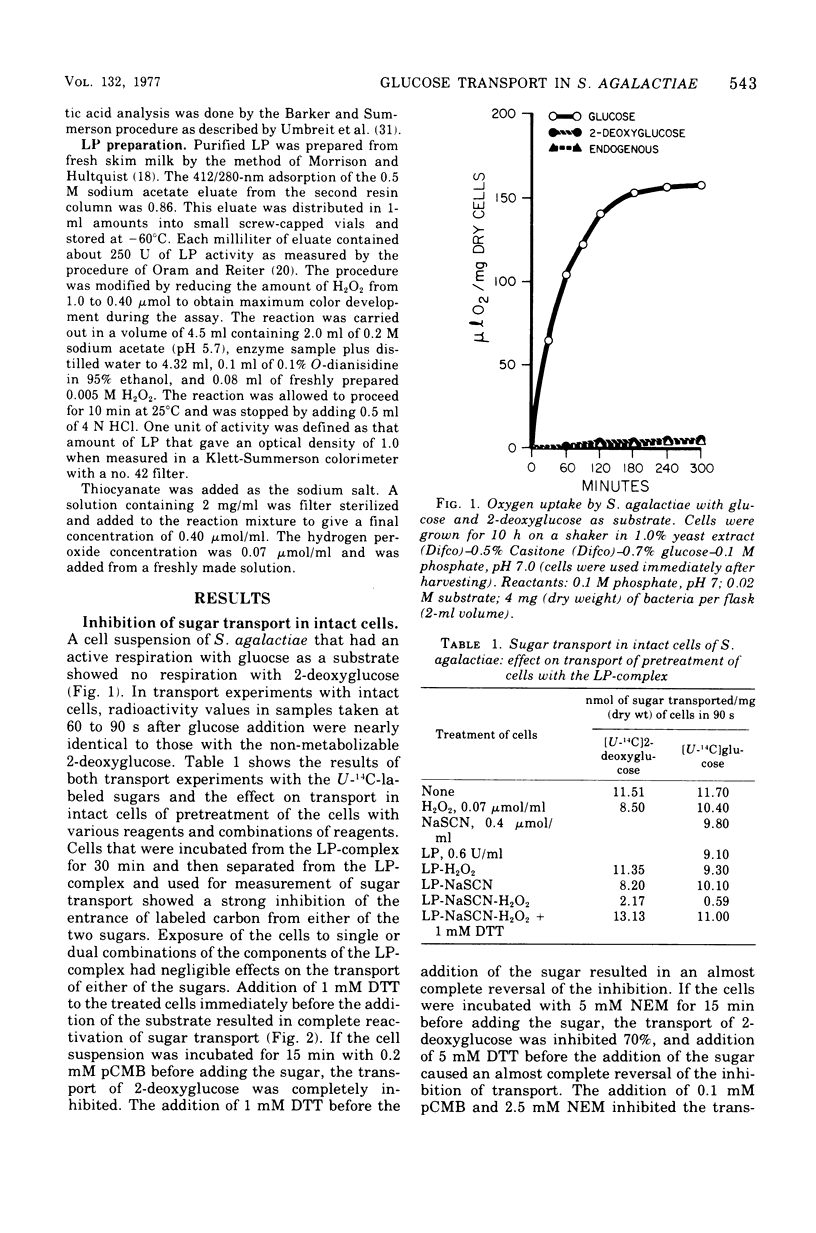
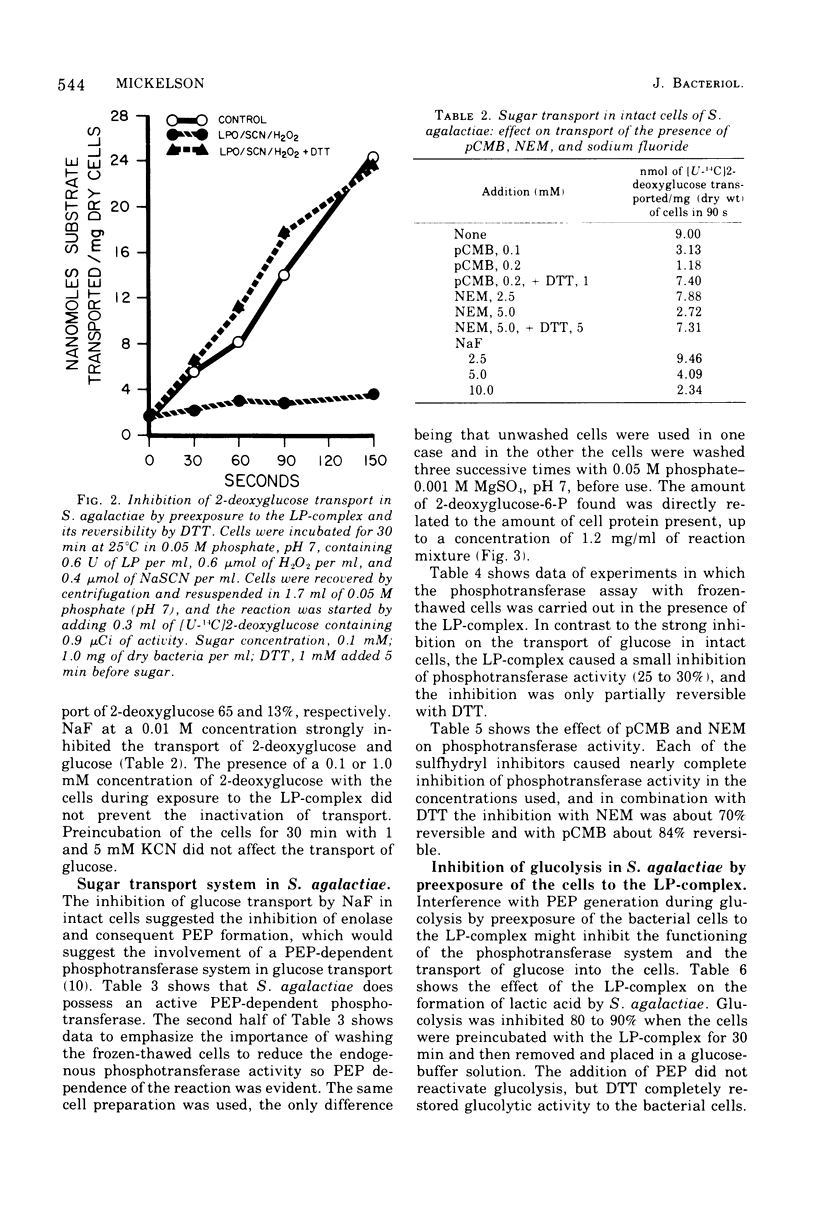
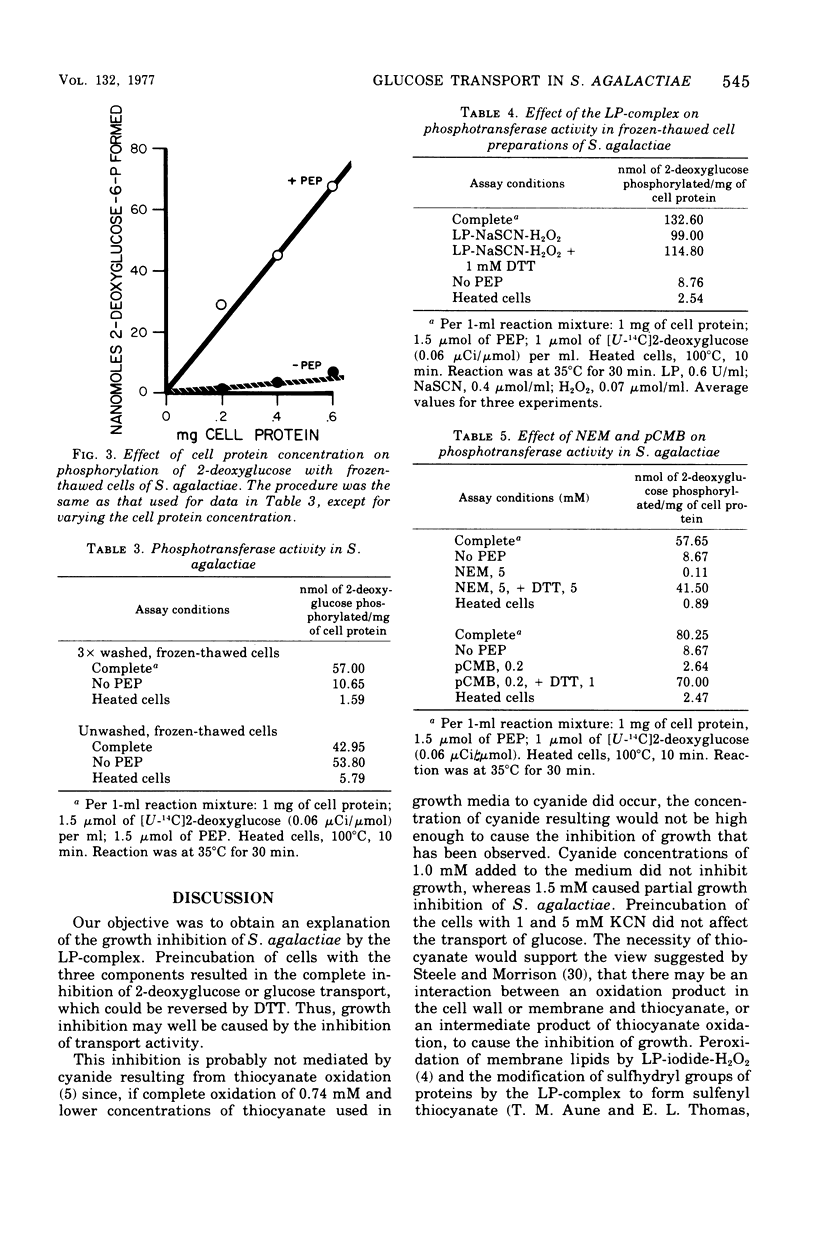
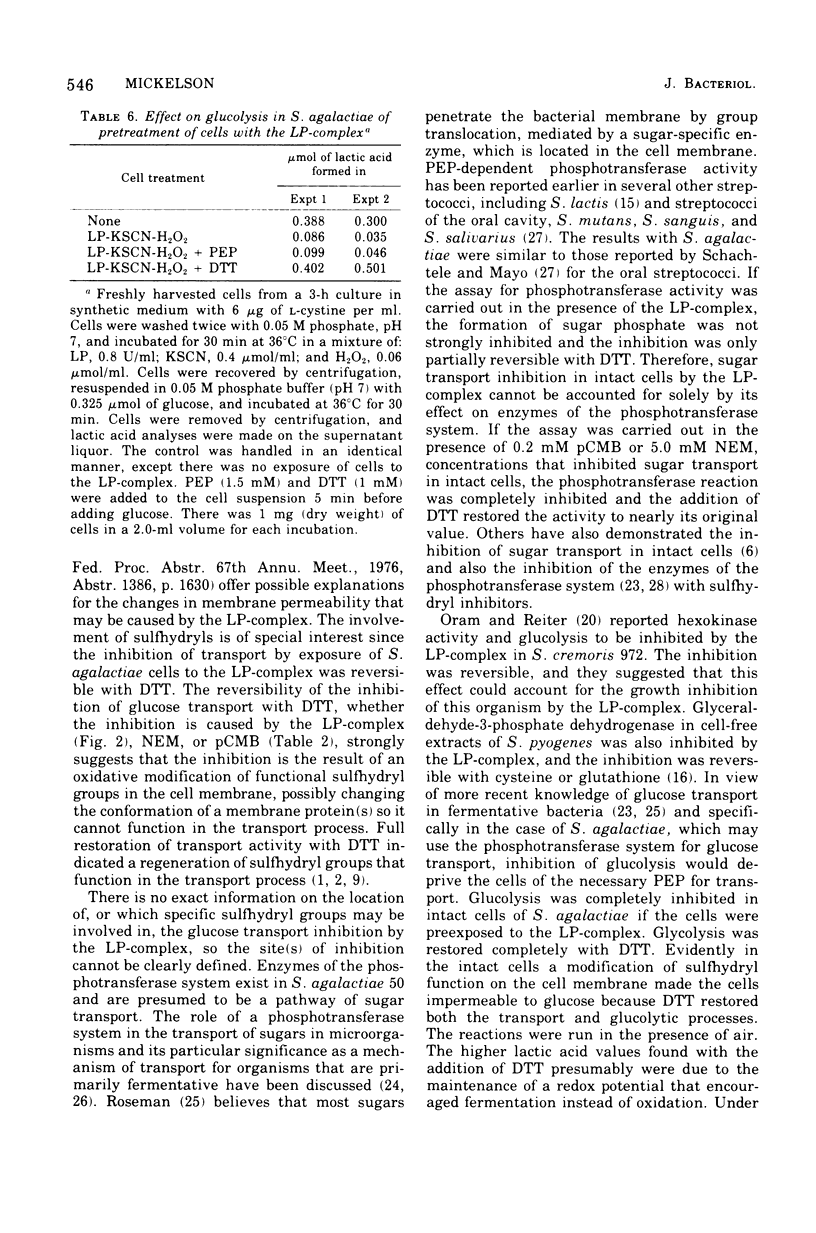
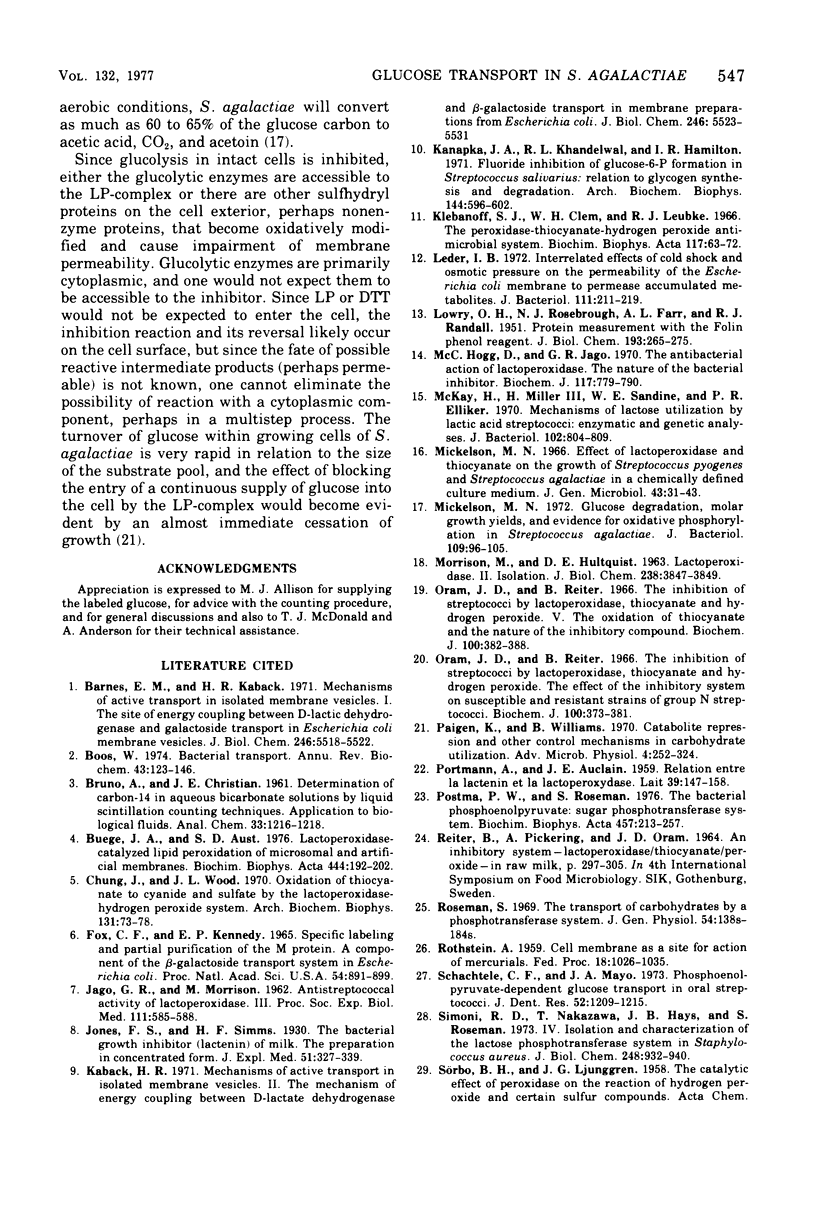
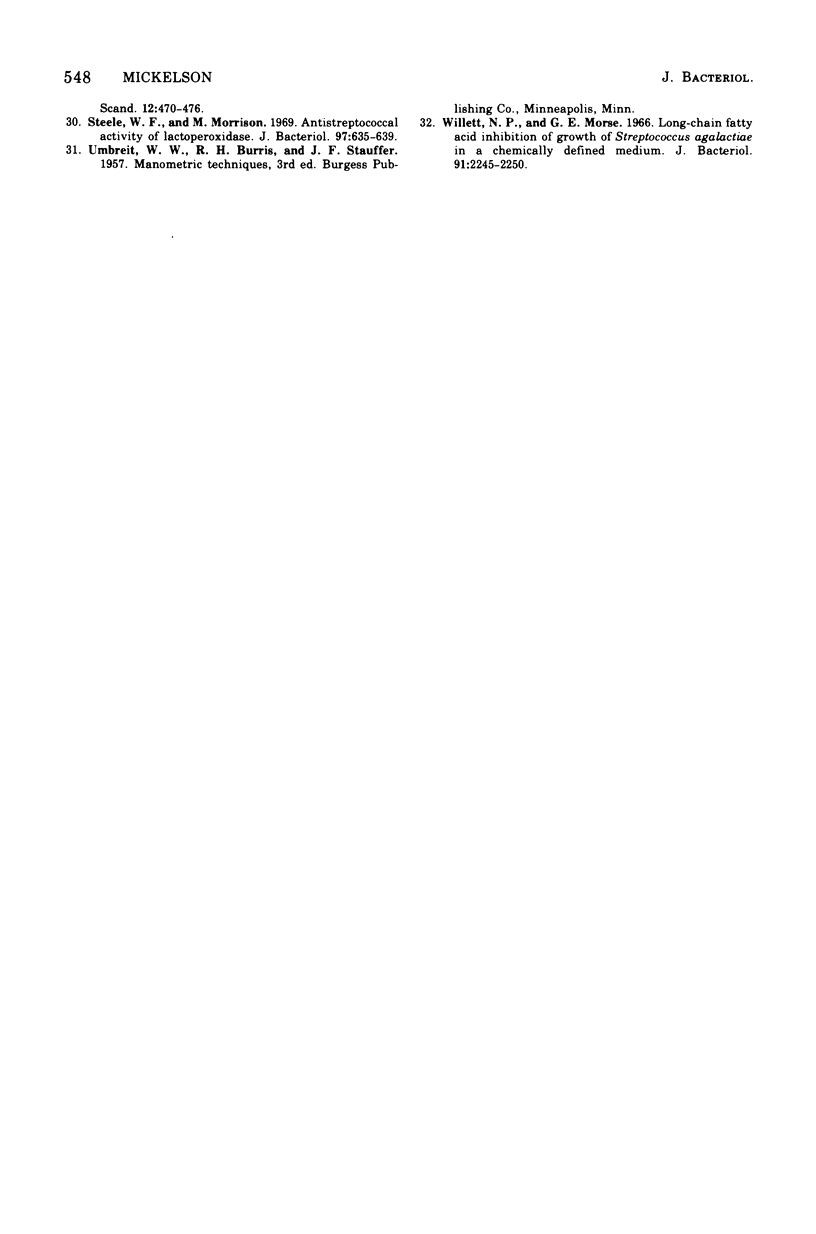
Selected References
These references are in PubMed. This may not be the complete list of references from this article.
- Barnes E. M., Jr, Kaback H. R. Mechanisms of active transport in isolated membrane vesicles. I. The site of energy coupling between D-lactic dehydrogenase and beta-galactoside transport in Escherichia coli membrane vesicles. J Biol Chem. 1971 Sep 10;246(17):5518–5522. [PubMed] [Google Scholar]
- Boos W. Bacterial transport. Annu Rev Biochem. 1974;43(0):123–146. doi: 10.1146/annurev.bi.43.070174.001011. [DOI] [PubMed] [Google Scholar]
- Buege J. A., Aust S. D. Lactoperoxidase-catalyzed lipid peroxidation of microsomal and artificial membranes. Biochim Biophys Acta. 1976 Aug 24;444(1):192–201. doi: 10.1016/0304-4165(76)90236-1. [DOI] [PubMed] [Google Scholar]
- Chung J., Wood J. L. Oxidation of thiocyanate to cyanide and sulfate by the lactoperoxidase-hydrogen peroxide system. Arch Biochem Biophys. 1970 Nov;141(1):73–78. doi: 10.1016/0003-9861(70)90108-6. [DOI] [PubMed] [Google Scholar]
- Fox C. F., Kennedy E. P. Specific labeling and partial purification of the M protein, a component of the beta-galactoside transport system of Escherichia coli. Proc Natl Acad Sci U S A. 1965 Sep;54(3):891–899. doi: 10.1073/pnas.54.3.891. [DOI] [PMC free article] [PubMed] [Google Scholar]
- Hogg D. M., Jago G. R. The antibacterial action of lactoperoxidase. The nature of the bacterial inhibitor. Biochem J. 1970 May;117(4):779–790. doi: 10.1042/bj1170779. [DOI] [PMC free article] [PubMed] [Google Scholar]
- JAGO G. R., MORRISON M. Anti-streptococcal activity of lactoperoxidase III. Proc Soc Exp Biol Med. 1962 Dec;111:585–588. doi: 10.3181/00379727-111-27862. [DOI] [PubMed] [Google Scholar]
- Kaback H. R., Barnes E. M., Jr Mechanisms of active transport in isolated membrane vesicles. II. The mechanism of energy coupling between D-lactic dehydrogenase and beta-galactoside transport in membrane preparations from Escherichia coli. J Biol Chem. 1971 Sep 10;246(17):5523–5531. [PubMed] [Google Scholar]
- Kanapka J. A., Khandelwal R. L., Hamilton I. R. Fluoride inhibition of glucose-6-P formation in Streptococcus salivarius: relation to glycogen synthesis and degradation. Arch Biochem Biophys. 1971 Jun;144(2):596–602. doi: 10.1016/0003-9861(71)90366-3. [DOI] [PubMed] [Google Scholar]
- Klebanoff S. J., Clem W. H., Luebke R. G. The peroxidase-thiocyanate-hydrogen peroxide antimicrobial system. Biochim Biophys Acta. 1966 Mar 28;117(1):63–72. doi: 10.1016/0304-4165(66)90152-8. [DOI] [PubMed] [Google Scholar]
- LOWRY O. H., ROSEBROUGH N. J., FARR A. L., RANDALL R. J. Protein measurement with the Folin phenol reagent. J Biol Chem. 1951 Nov;193(1):265–275. [PubMed] [Google Scholar]
- Leder I. G. Interrelated effects of cold shock and osmotic pressure on the permeability of the Escherichia coli membrane to permease accumulated substrates. J Bacteriol. 1972 Jul;111(1):211–219. doi: 10.1128/jb.111.1.211-219.1972. [DOI] [PMC free article] [PubMed] [Google Scholar]
- McKay L., Miller A., 3rd, Sandine W. E., Elliker P. R. Mechanisms of lactose utilization by lactic acid streptococci: enzymatic and genetic analyses. J Bacteriol. 1970 Jun;102(3):804–809. doi: 10.1128/jb.102.3.804-809.1970. [DOI] [PMC free article] [PubMed] [Google Scholar]
- Mickelson M. N. Effect of lactoperoxidase and thiocyanate on the growth of Streptococcus pyogenes and Streptococcus agalactiae in a chemically defined culture medium. J Gen Microbiol. 1966 Apr;43(1):31–43. doi: 10.1099/00221287-43-1-31. [DOI] [PubMed] [Google Scholar]
- Mickelson M. N. Glucose degradation, molar growth yields, and evidence for oxidative phosphorylation in Streptococcus agalactiae. J Bacteriol. 1972 Jan;109(1):96–105. doi: 10.1128/jb.109.1.96-105.1972. [DOI] [PMC free article] [PubMed] [Google Scholar]
- Oram J. D., Reiter B. The inhibition of streptococci by lactoperoxidase, thiocyanate and hydrogen peroxide. The effect of the inhibitory system on susceptible and resistant strains of group N streptococci. Biochem J. 1966 Aug;100(2):373–381. doi: 10.1042/bj1000373. [DOI] [PMC free article] [PubMed] [Google Scholar]
- Oram J. D., Reiter B. The inhibition of streptococci by lactoperoxidase, thiocyanate and hydrogen peroxide. The oxidation of thiocyanate and the nature of the inhibitory compound. Biochem J. 1966 Aug;100(2):382–388. doi: 10.1042/bj1000382. [DOI] [PMC free article] [PubMed] [Google Scholar]
- Postma P. W., Roseman S. The bacterial phosphoenolpyruvate: sugar phosphotransferase system. Biochim Biophys Acta. 1976 Dec 14;457(3-4):213–257. doi: 10.1016/0304-4157(76)90001-0. [DOI] [PubMed] [Google Scholar]
- ROTHSTEIN A. Cell membrane as site of action of heavy metals. Fed Proc. 1959 Dec;18:1026–1038. [PubMed] [Google Scholar]
- Schachtele C. F., Mayo J. A. Phosphoenolpyruvate-dependent glucose transport in oral streptococci. J Dent Res. 1973 Nov-Dec;52(6):1209–1215. doi: 10.1177/00220345730520060801. [DOI] [PubMed] [Google Scholar]
- Simoni R. D., Nakazawa T., Hays J. B., Roseman S. Sugar transport. IV. Isolation and characterization of the lactose phosphotransferase system in Staphylococcus aureus. J Biol Chem. 1973 Feb 10;248(3):932–940. [PubMed] [Google Scholar]
- Steele W. F., Morrison M. Antistreptococcal activity of lactoperoxidase. J Bacteriol. 1969 Feb;97(2):635–639. doi: 10.1128/jb.97.2.635-639.1969. [DOI] [PMC free article] [PubMed] [Google Scholar]
- Willett N. P., Morse G. E. Long-chain fatty acid inhibition of growth of Streptococcus agalactiae in a chemically defined medium. J Bacteriol. 1966 Jun;91(6):2245–2250. doi: 10.1128/jb.91.6.2245-2250.1966. [DOI] [PMC free article] [PubMed] [Google Scholar]