Abstract
Proton influx was measured after imposition of an electrochemical potential difference for protons (delta muH+) across the cell membrane of the anaerobe, Streptococcus lactis. As delta muH+ was increased, there was an approximately parallel increase in proton entry, until delta muH+ attained 175 to 200 mV. At this point, a new pathway became available for proton entry, allowing an abrupt increase in both the rate and extent of H+ influx. This gated response depended upon the value of delta muH+ itself, and not upon the value of either the membrane potential or the pH gradient. For delta muH+ above 175 to 200 mV, elevated proton entry occurred only in cells having a functional membrane-bound Ca2+-stimulated, Mg2+stimulated adenosine 5'-triphosphatase (EC 3.6.1.3). When present, elevated proton entry coincided with the appearance of net synthesis of adenosine 5'-triphosphate catalyzed by this adenosine 5'-triphosphatase. These observations demonstrate that membrane-bound adenosine 5'-triphosphatase catalyzes an obligatory coupling between the inward movement of protons and synthesis of adenosine 5'-triphosphate.
Full text
PDF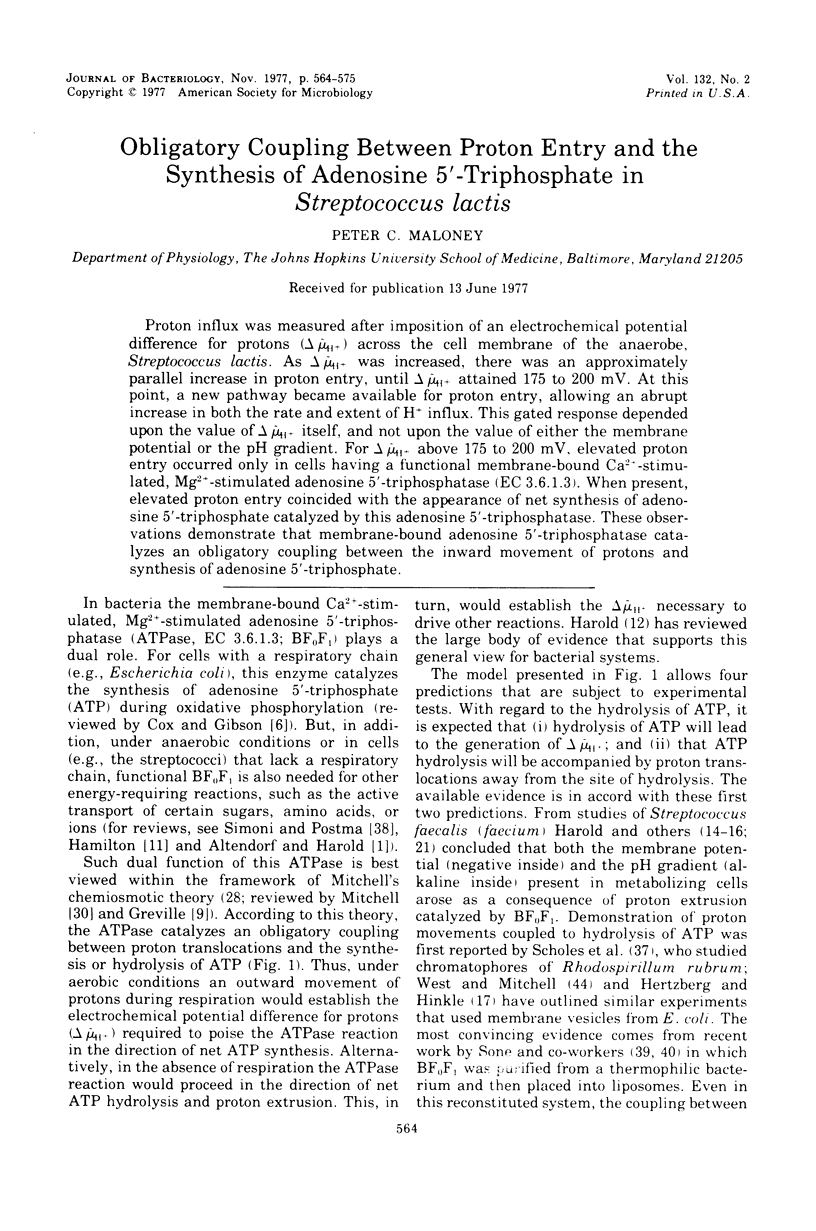
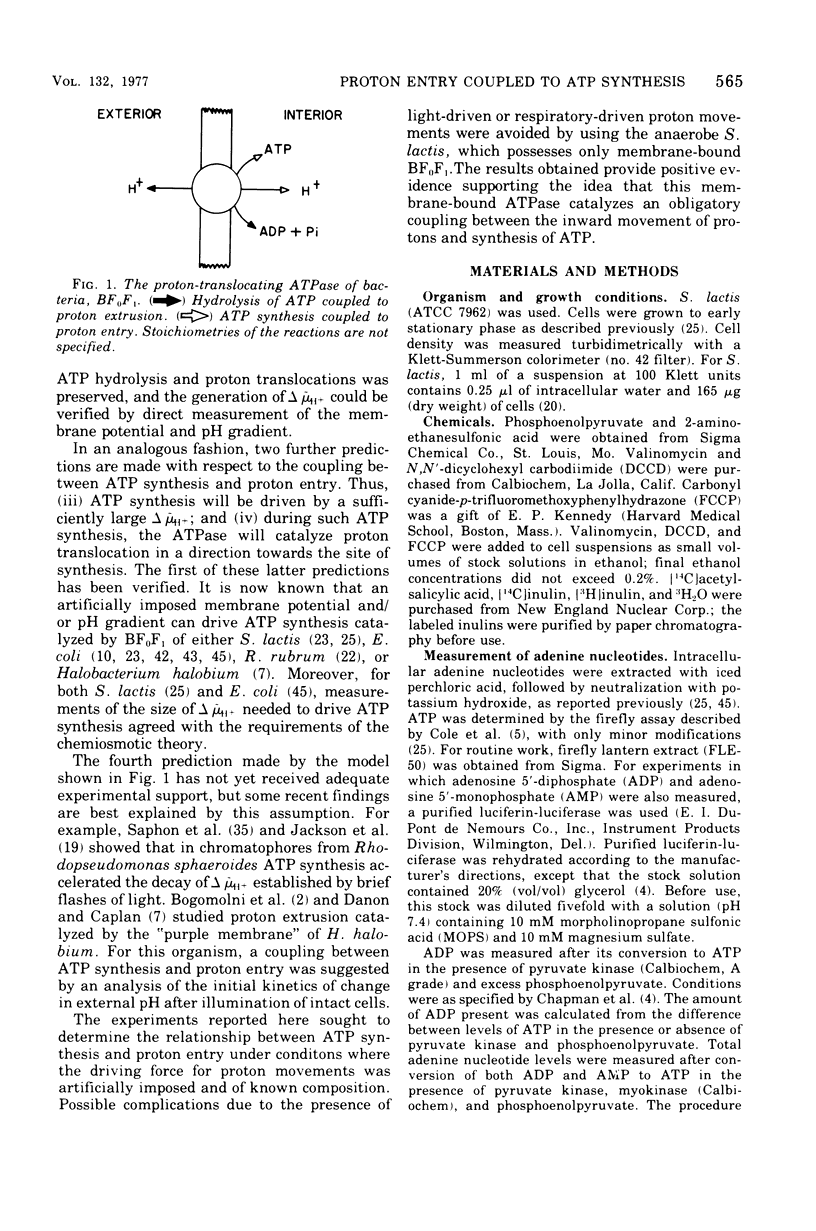
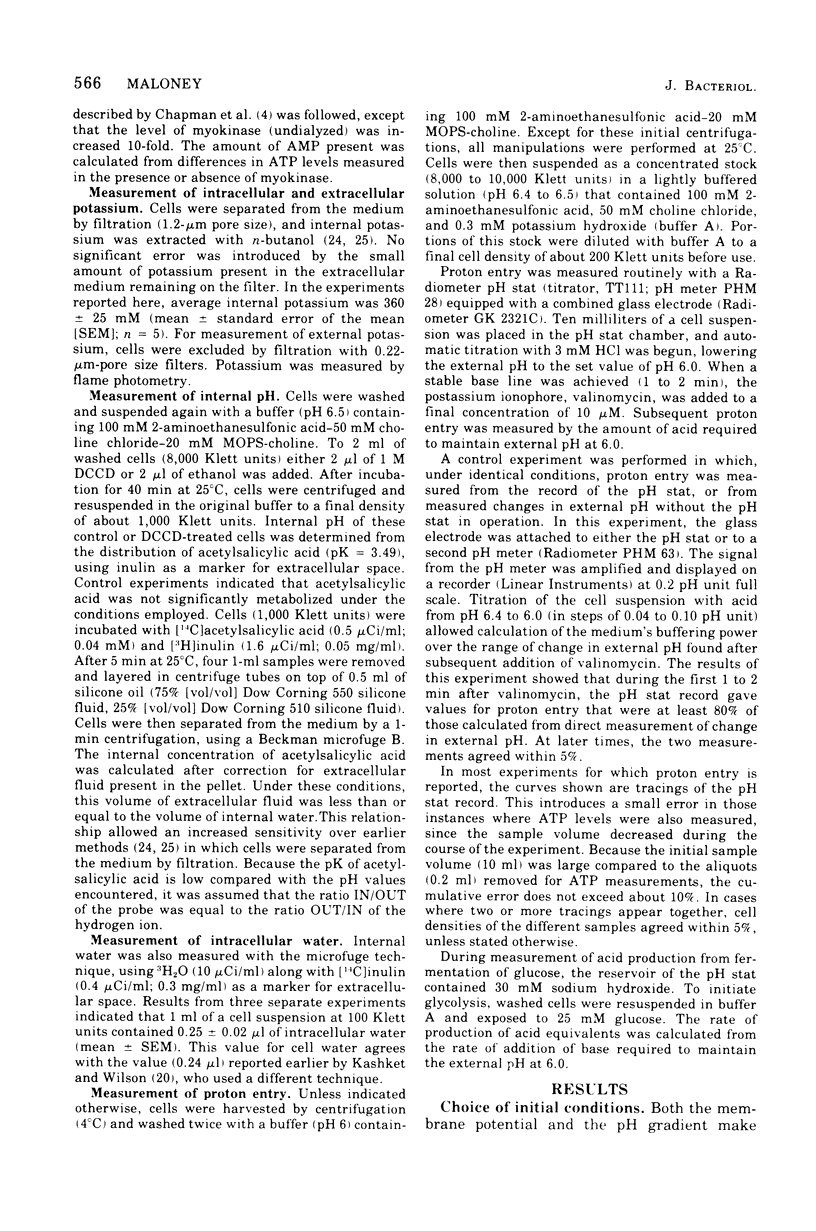
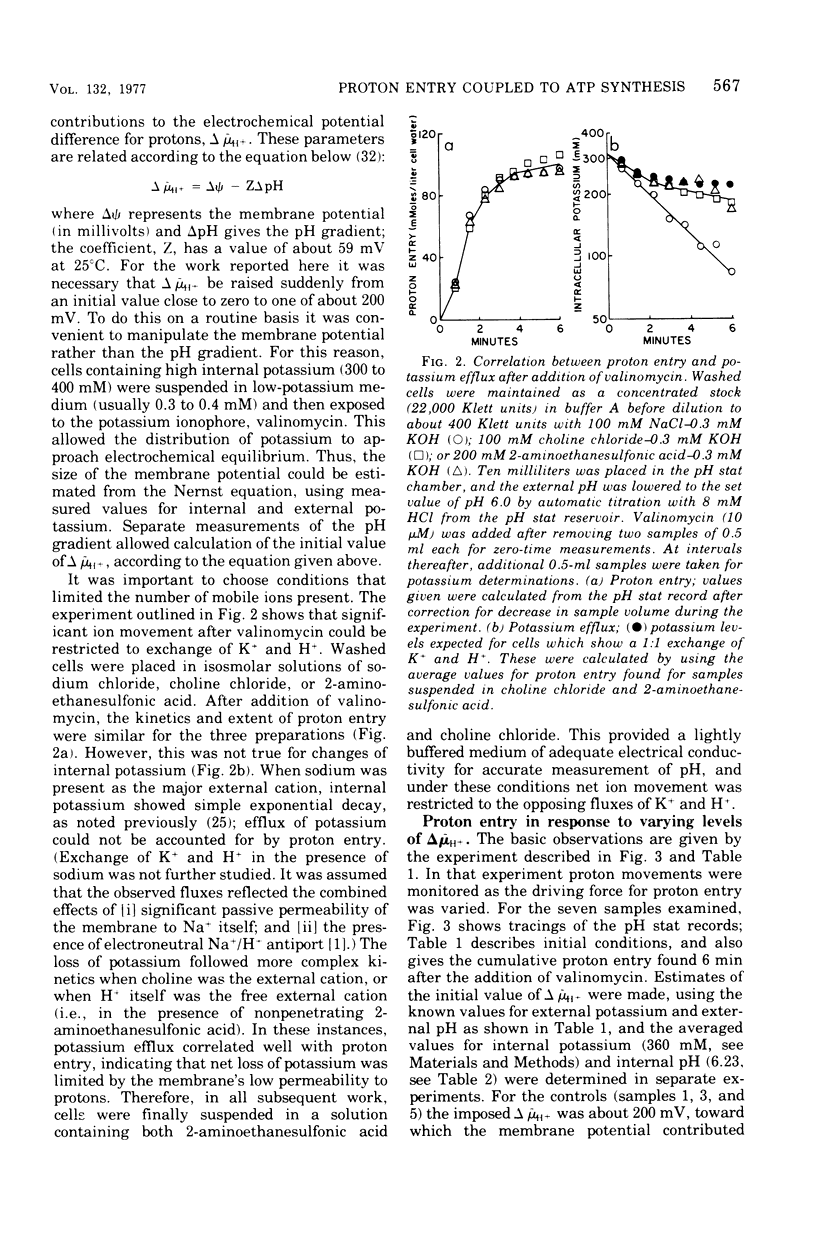
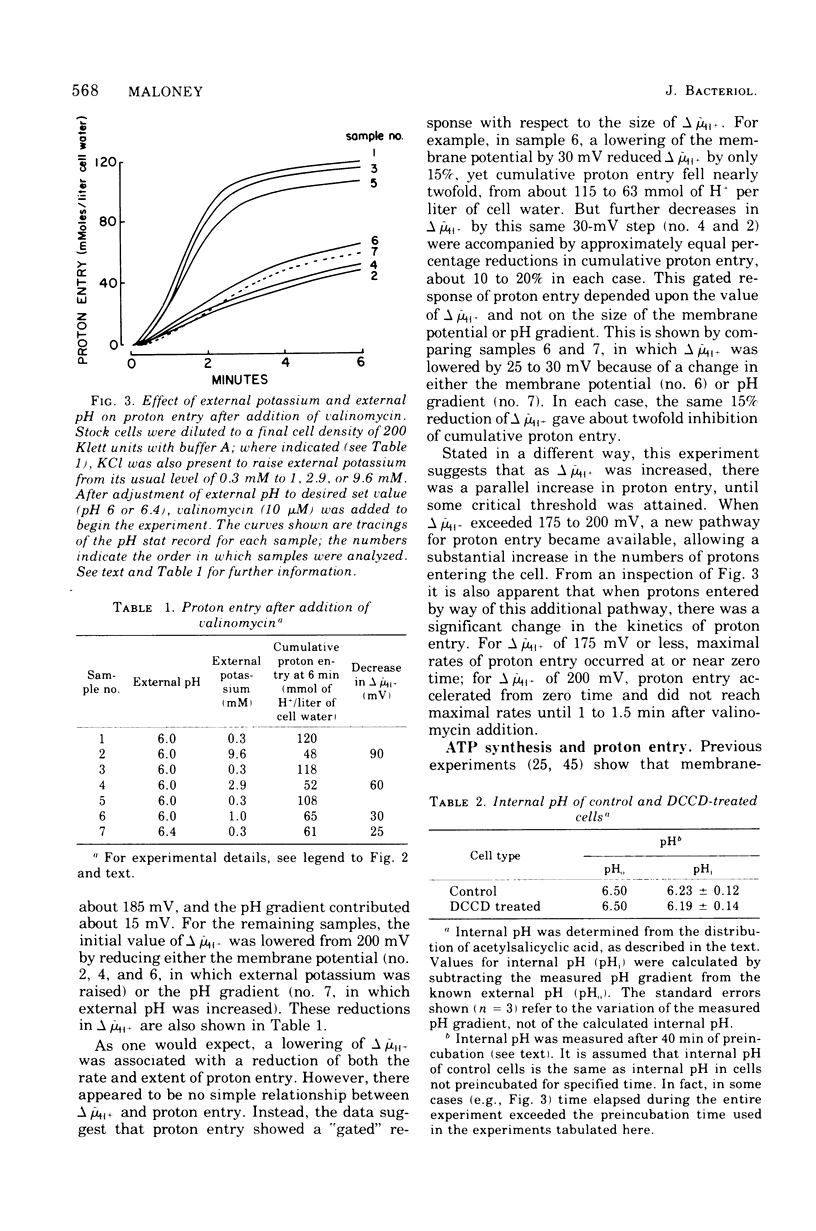
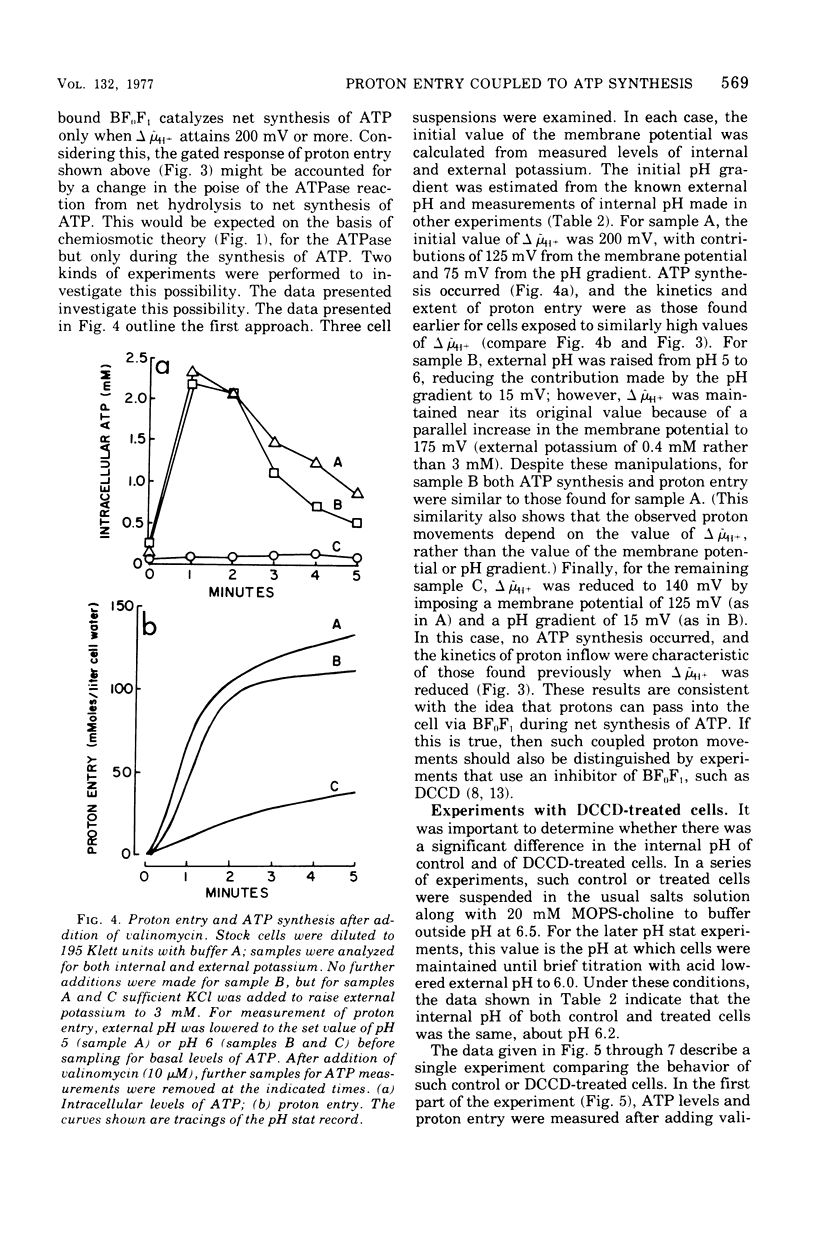
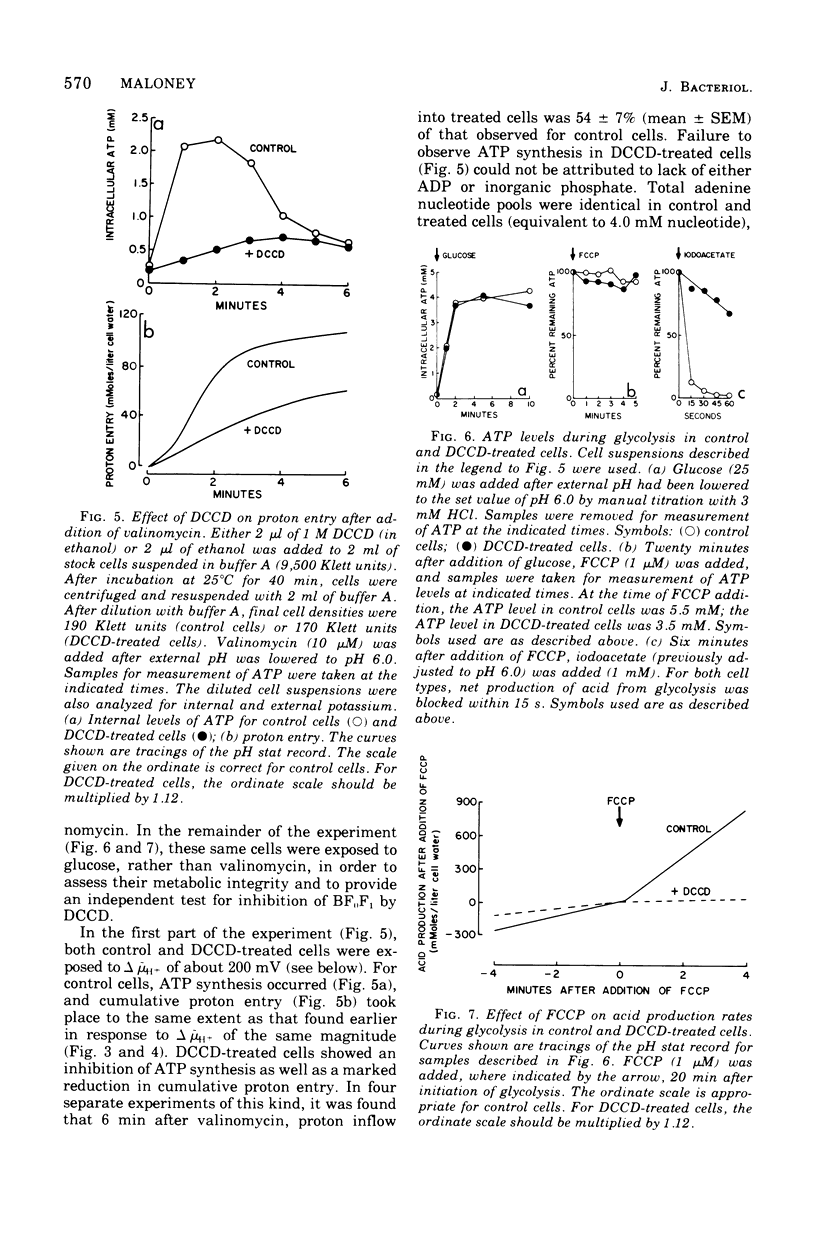
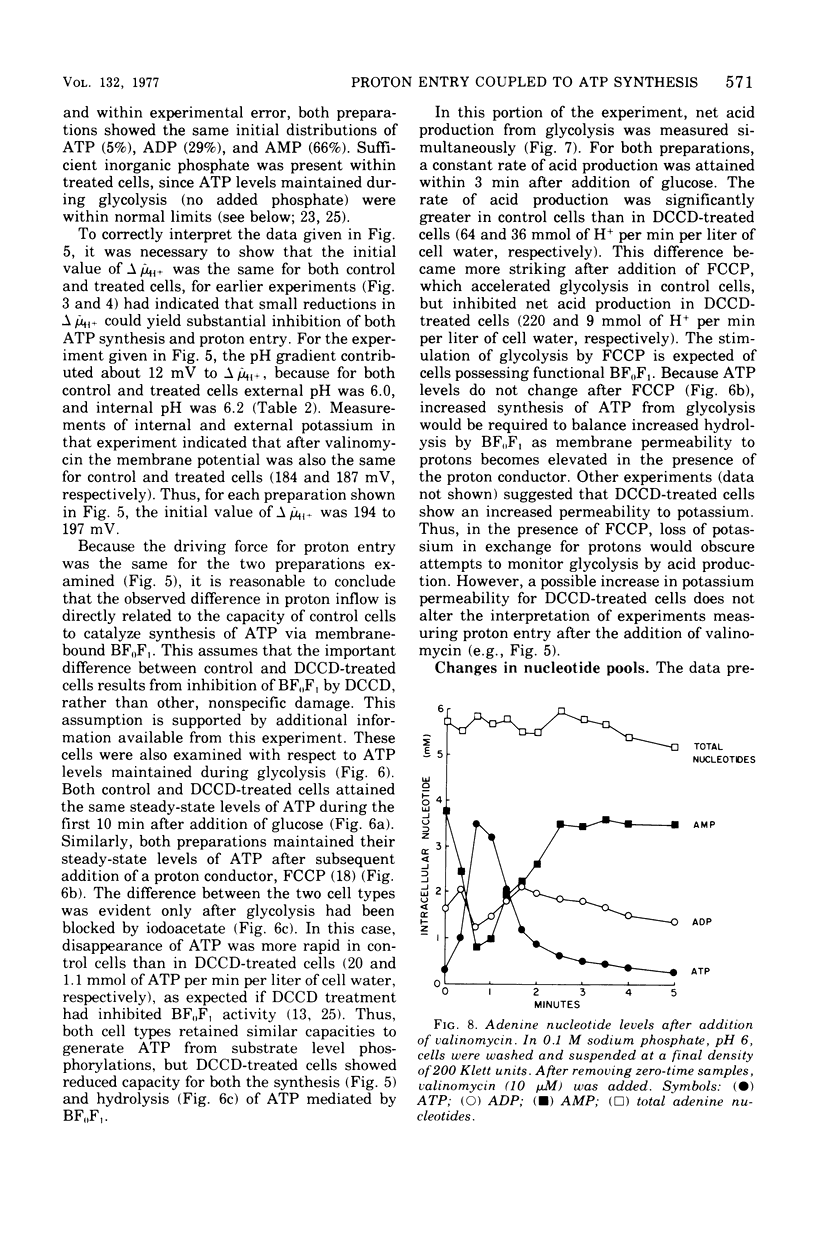
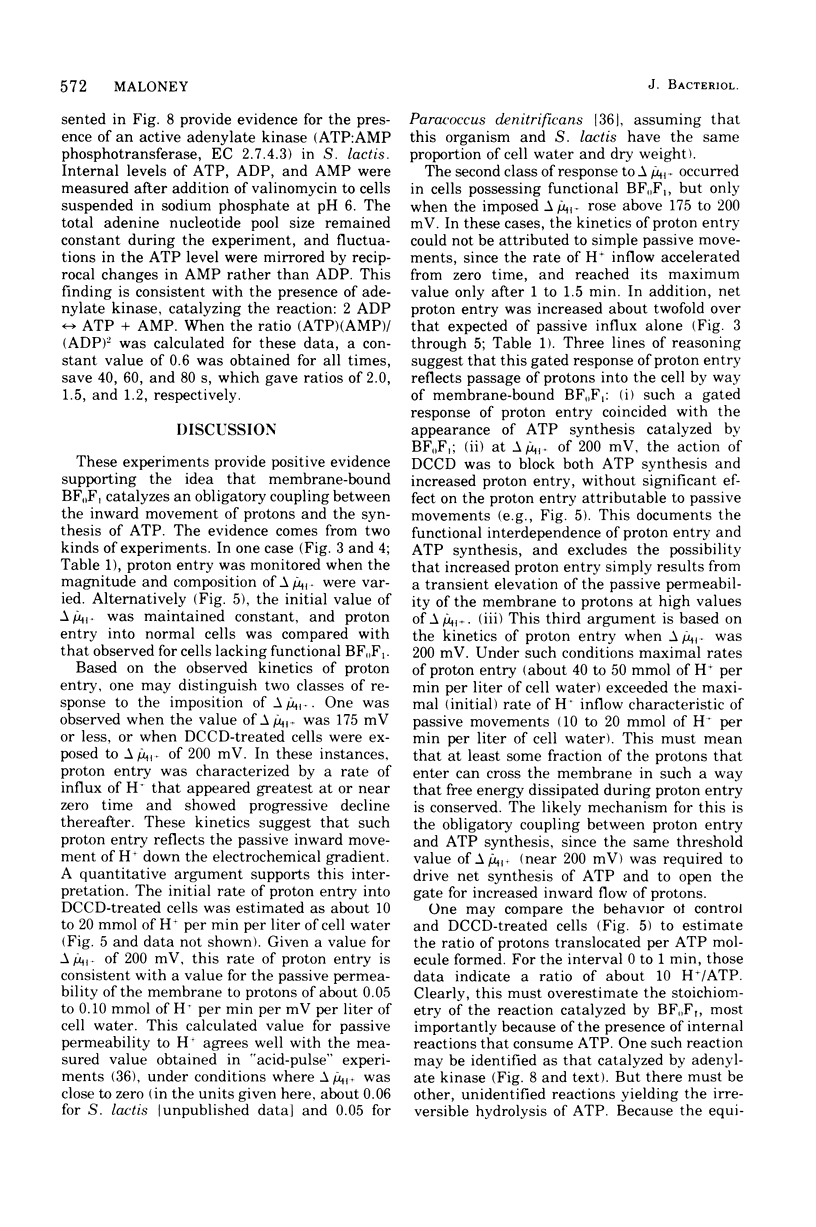
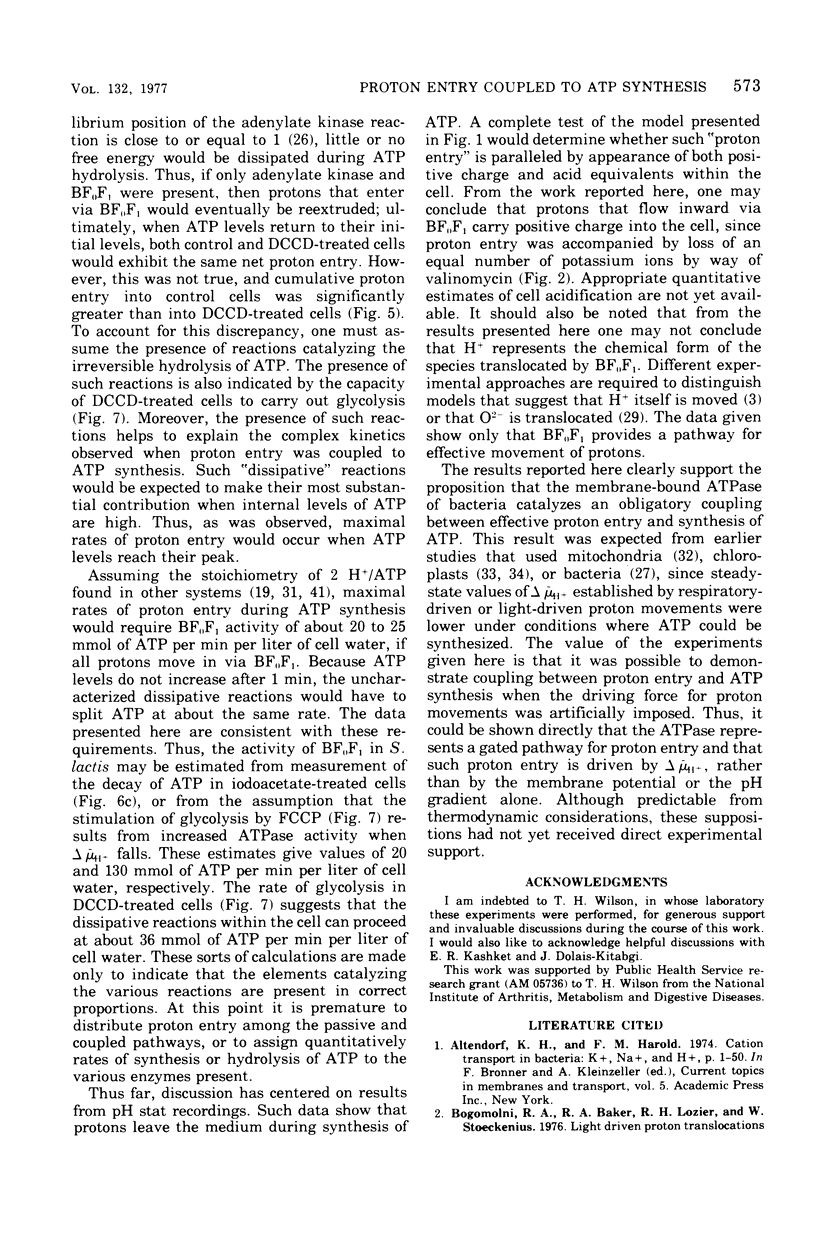
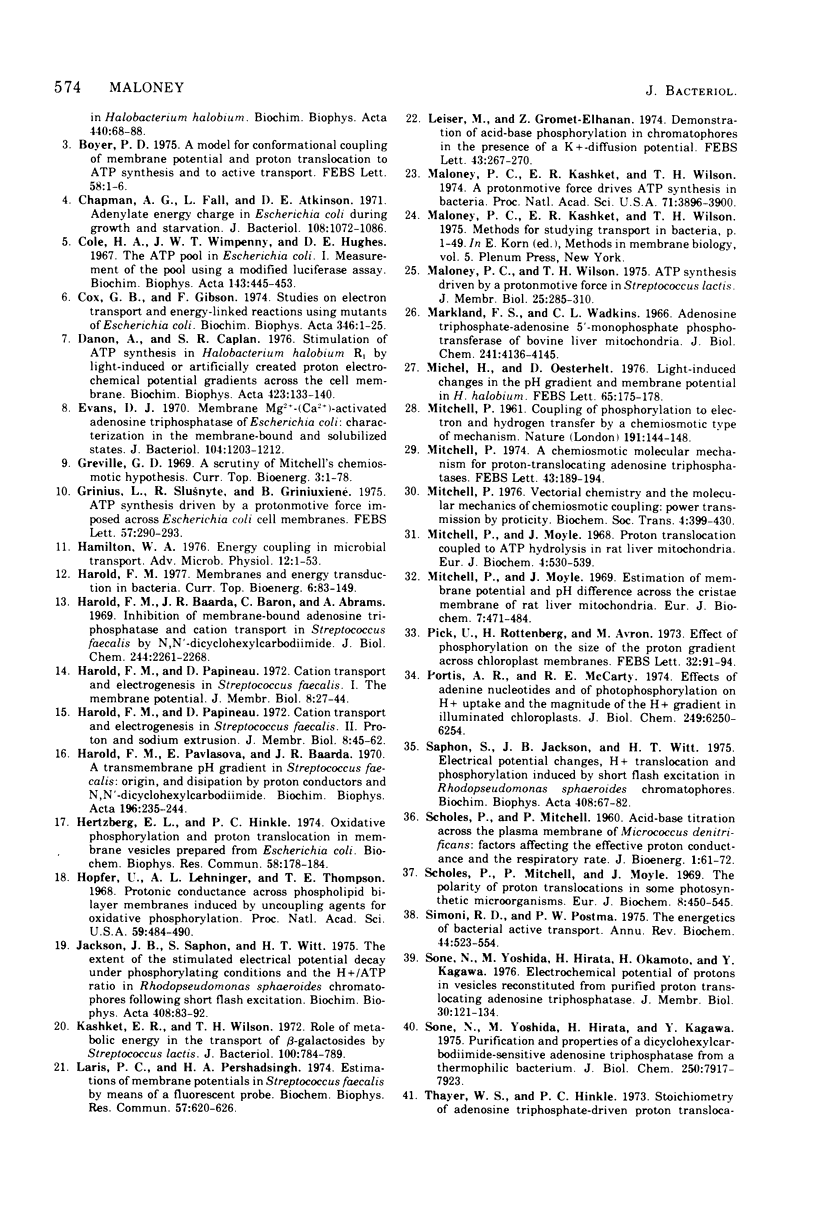
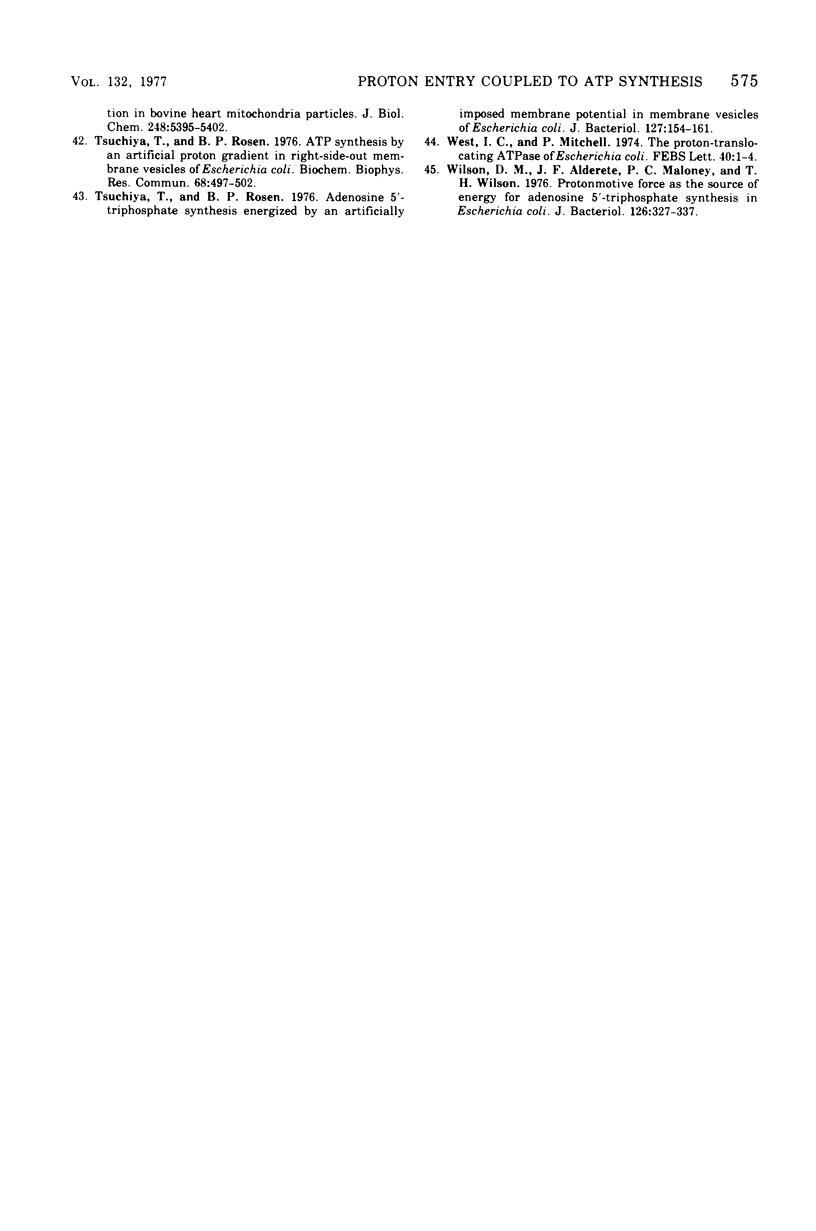
Selected References
These references are in PubMed. This may not be the complete list of references from this article.
- Bogomolni R. A., Baker R. A., Lozier R. H., Stoeckenius W. Light-driven proton translocations in Halobacterium halobium. Biochim Biophys Acta. 1976 Jul 9;440(1):68–88. doi: 10.1016/0005-2728(76)90114-6. [DOI] [PubMed] [Google Scholar]
- Boyer P. D. A model for conformational coupling of membrane potential and proton translocation to ATP synthesis and to active transport. FEBS Lett. 1975 Oct 15;58(1):1–6. doi: 10.1016/0014-5793(75)80212-2. [DOI] [PubMed] [Google Scholar]
- Chapman A. G., Fall L., Atkinson D. E. Adenylate energy charge in Escherichia coli during growth and starvation. J Bacteriol. 1971 Dec;108(3):1072–1086. doi: 10.1128/jb.108.3.1072-1086.1971. [DOI] [PMC free article] [PubMed] [Google Scholar]
- Cole H. A., Wimpenny J. W., Hughes D. E. The ATP pool in Escherichia coli. I. Measurement of the pool using modified luciferase assay. Biochim Biophys Acta. 1967;143(3):445–453. doi: 10.1016/0005-2728(67)90050-3. [DOI] [PubMed] [Google Scholar]
- Cox G. B., Gibson F. Studies on electron transport and energy-linked reactions using mutants of Escherichia coli. Biochim Biophys Acta. 1974 Apr 30;346(1):1–25. doi: 10.1016/0304-4173(74)90010-x. [DOI] [PubMed] [Google Scholar]
- Danon A., Caplan S. R. Stimulation of ATP synthesis in Halobacterium halobium R1 by light-induced or artifically created proton electrochemical potential gradients across the cell membrane. Biochim Biophys Acta. 1976 Jan 15;423(1):133–140. doi: 10.1016/0005-2728(76)90107-9. [DOI] [PubMed] [Google Scholar]
- Evans D. J. Membrane Mg-(Ca)-Activated Adenosine Triphosphatase of Escherichia coli: Characterization in the Membrane-Bound and Solubilized States. J Bacteriol. 1970 Dec;104(3):1203–1212. doi: 10.1128/jb.104.3.1203-1212.1970. [DOI] [PMC free article] [PubMed] [Google Scholar]
- Grinius L., Slusnyte R., Griniuviene B. ATP synthesis driven by protonmotive force imposed across Escherichia coli cell membranes. FEBS Lett. 1975 Oct 1;57(3):290–293. doi: 10.1016/0014-5793(75)80319-x. [DOI] [PubMed] [Google Scholar]
- Harold F. M., Baarda J. R., Baron C., Abrams A. Inhibition of membrane-bound adenosine triphosphatase and of cation transport in Streptococcus faecalis by N,N'-dicyclohexylcarbodiimide. J Biol Chem. 1969 May 10;244(9):2261–2268. [PubMed] [Google Scholar]
- Harold F. M., Papineau D. Cation transport and electrogenesis by Streptococcus faecalis. I. The membrane potential. J Membr Biol. 1972;8(1):27–44. doi: 10.1007/BF01868093. [DOI] [PubMed] [Google Scholar]
- Harold F. M., Papineau D. Cation transport and electrogenesis by Streptococcus faecalis. II. Proton and sodium extrusion. J Membr Biol. 1972;8(1):45–62. doi: 10.1007/BF01868094. [DOI] [PubMed] [Google Scholar]
- Harold F. M., Pavlasová E., Baarda J. R. A transmembrane pH gradient in Streptococcus faecalis: origin, and dissipation by proton conductors and N,N'-dicyclohexylcarbodimide. Biochim Biophys Acta. 1970;196(2):235–244. doi: 10.1016/0005-2736(70)90011-8. [DOI] [PubMed] [Google Scholar]
- Hertzberg E. L., Hinkle P. C. Oxidative phosphorylation and proton translocation in membrane vesicles prepared from Escherichia coli. Biochem Biophys Res Commun. 1974 May 7;58(1):178–184. doi: 10.1016/0006-291x(74)90908-5. [DOI] [PubMed] [Google Scholar]
- Hopfer U., Lehninger A. L., Thompson T. E. Protonic conductance across phospholipid bilayer membranes induced by uncoupling agents for oxidative phosphorylation. Proc Natl Acad Sci U S A. 1968 Feb;59(2):484–490. doi: 10.1073/pnas.59.2.484. [DOI] [PMC free article] [PubMed] [Google Scholar]
- Jackson J. B., Saphon S., Witt H. T. The extent of the stimulated electrical potential decay under phosphorylating conditions and the H+/ATP ratio in Rhodopseudomonas sphaeroides chromatophores following short flash excitation. Biochim Biophys Acta. 1975 Oct 10;408(1):83–92. doi: 10.1016/0005-2728(75)90160-7. [DOI] [PubMed] [Google Scholar]
- Kashket E. R., Wilson T. H. Role of metabolic energy in the transport of -galactosides by Streptococcus lactis. J Bacteriol. 1972 Feb;109(2):784–789. doi: 10.1128/jb.109.2.784-789.1972. [DOI] [PMC free article] [PubMed] [Google Scholar]
- Laris P. C., Pershadsingh H. A. Estimations of membrane potentials in Streptococcus faecalis by means of a fluorescent probe. Biochem Biophys Res Commun. 1974 Apr 8;57(3):620–626. doi: 10.1016/0006-291x(74)90591-9. [DOI] [PubMed] [Google Scholar]
- Leiser M., Gromet-Elhanan Z. Demonstration of acid-base phosphorylation in chromatophores in the presence of a K+ diffusion potential. FEBS Lett. 1974 Aug 1;43(3):267–270. doi: 10.1016/0014-5793(74)80658-7. [DOI] [PubMed] [Google Scholar]
- MITCHELL P. Coupling of phosphorylation to electron and hydrogen transfer by a chemi-osmotic type of mechanism. Nature. 1961 Jul 8;191:144–148. doi: 10.1038/191144a0. [DOI] [PubMed] [Google Scholar]
- Maloney P. C., Kashket E. R., Wilson T. H. A protonmotive force drives ATP synthesis in bacteria. Proc Natl Acad Sci U S A. 1974 Oct;71(10):3896–3900. doi: 10.1073/pnas.71.10.3896. [DOI] [PMC free article] [PubMed] [Google Scholar]
- Maloney P. C., Wilson T. H. ATP synthesis driven by a protonmotive force in Streptococcus lactis. J Membr Biol. 1975;25(3-4):285–310. doi: 10.1007/BF01868580. [DOI] [PubMed] [Google Scholar]
- Markland F. S., Wadkins C. L. Adenosine triphosphate-adenosine 5'-monophosphate phosphotransferase of bovine liver mitochondria. II. General kinetic and structural properties. J Biol Chem. 1966 Sep 25;241(18):4136–4145. [PubMed] [Google Scholar]
- Michel H., Oesterhelt D. Light-induced changes of the pH gradient and the membrane potential in H. halobium. FEBS Lett. 1976 Jun 1;65(2):175–178. doi: 10.1016/0014-5793(76)80473-5. [DOI] [PubMed] [Google Scholar]
- Mitchell P. A chemiosmotic molecular mechanism for proton-translocating adenosine triphosphatases. FEBS Lett. 1974 Jul 15;43(2):189–194. doi: 10.1016/0014-5793(74)80997-x. [DOI] [PubMed] [Google Scholar]
- Mitchell P., Moyle J. Estimation of membrane potential and pH difference across the cristae membrane of rat liver mitochondria. Eur J Biochem. 1969 Feb;7(4):471–484. doi: 10.1111/j.1432-1033.1969.tb19633.x. [DOI] [PubMed] [Google Scholar]
- Mitchell P., Moyle J. Proton translocation coupled to ATP hydrolysis in rat liver mitochondria. Eur J Biochem. 1968 May;4(4):530–539. doi: 10.1111/j.1432-1033.1968.tb00245.x. [DOI] [PubMed] [Google Scholar]
- Mitchell P. Vectorial chemistry and the molecular mechanics of chemiosmotic coupling: power transmission by proticity. Biochem Soc Trans. 1976;4(3):399–430. doi: 10.1042/bst0040399. [DOI] [PubMed] [Google Scholar]
- Pick U., Rottenberg H., Avron M. Effect of phosphorylation on the size of the proton gradient across chloroplast membranes. FEBS Lett. 1973 May 15;32(1):91–94. doi: 10.1016/0014-5793(73)80745-8. [DOI] [PubMed] [Google Scholar]
- Portis A. R., Jr, McCarty R. E. Effects of adenine nucleotides and of photophosphorylation on H+ uptake and the magnitude of the H+ gradient in illuminated chloroplasts. J Biol Chem. 1974 Oct 10;249(19):6250–6254. [PubMed] [Google Scholar]
- Saphon S., Jackson J. B., Witt H. T. Electrical potential changes, H+ translocation and phosphorylation induced by short flash excitation in Rhodopseudomonas sphaeroides chromatophores. Biochim Biophys Acta. 1975 Oct 10;408(1):67–82. doi: 10.1016/0005-2728(75)90159-0. [DOI] [PubMed] [Google Scholar]
- Scholes P., Mitchell P. Acid-base titration across the plasma membrane of Micrococcus denitrificans: factors affecting the effective proton conductance and the respiratory rate. J Bioenerg. 1970 Jun;1(1):61–72. doi: 10.1007/BF01516089. [DOI] [PubMed] [Google Scholar]
- Scholes P., Mitchell P., Moyle J. The polarity of proton translocation in some photosynthetic microorganisms. Eur J Biochem. 1969 Apr;8(3):450–454. doi: 10.1111/j.1432-1033.1969.tb00548.x. [DOI] [PubMed] [Google Scholar]
- Simoni R. D., Postma P. W. The energetics of bacterial active transport. Annu Rev Biochem. 1975;44:523–554. doi: 10.1146/annurev.bi.44.070175.002515. [DOI] [PubMed] [Google Scholar]
- Sone N., Yoshida M., Hirata H., Kagawa Y. Purification and properties of a dicyclohexylcarbodiimide-sensitive adenosine triphosphatase from a thermophilic bacterium. J Biol Chem. 1975 Oct 10;250(19):7917–7923. [PubMed] [Google Scholar]
- Sone N., Yoshida M., Hirata H., Okamoto H., Kagawa Y. Electrochemical potential of protons in vesicles reconstituted from purified, proton-translocating adenosine triphosphatase. J Membr Biol. 1976 Dec 28;30(2):121–134. doi: 10.1007/BF01869663. [DOI] [PubMed] [Google Scholar]
- Thayer W. S., Hinkle P. C. Stoichiometry of adenosine triphosphate-driven proton translocation in bovine heart submitochondrial particles. J Biol Chem. 1973 Aug 10;248(15):5395–5402. [PubMed] [Google Scholar]
- Tsuchiya T., Rosen B. P. ATP synthesis by an artificial proton gradient in right-side-out membrane vesicles of Escherichia coli. Biochem Biophys Res Commun. 1976 Jan 26;68(2):497–502. doi: 10.1016/0006-291x(76)91173-6. [DOI] [PubMed] [Google Scholar]
- Tsuchiya T., Rosen B. P. Adenosine 5'-triphosphate synthesis energized by an artificially imposed membrane potential in membrane vesicles of Escherichia coli. J Bacteriol. 1976 Jul;127(1):154–161. doi: 10.1128/jb.127.1.154-161.1976. [DOI] [PMC free article] [PubMed] [Google Scholar]
- West I. C., Mitchell P. The proton-translocating ATPase of Escherichia coli. FEBS Lett. 1974 Mar 15;40(1):1–4. doi: 10.1016/0014-5793(74)80880-x. [DOI] [PubMed] [Google Scholar]
- Wilson D. M., Alderette J. F., Maloney P. C., Wilson T. H. Protonmotive force as the source of energy for adenosine 5'-triphosphate synthesis in Escherichia coli. J Bacteriol. 1976 Apr;126(1):327–337. doi: 10.1128/jb.126.1.327-337.1976. [DOI] [PMC free article] [PubMed] [Google Scholar]