Abstract
BACKGROUND: Using fluorescently labeled superoxide dismutase (SOD) and flow cytometry, we have shown previously that the enzyme CuZn SOD (EC 1.15.1.1) from bovine erythrocytes binds rapidly to the cell surface with slow uptake into the cell during the following hours. The degree of labeling was most important for monocytes in comparison to other blood cells (erythrocytes, lymphocytes, and neutrophils) and fibroblasts. In agreement with the flow-cytometric findings, the inhibition of superoxide production was more important for SOD-pretreated monocytes than for neutrophils, as demonstrated with the cytochrome c reduction assay. It was thus of interest to confirm the observed differences between monocytes and neutrophils with confocal laser microscopy, study in greater detail the kinetics of binding, penetration, and intracellular localization of the enzyme, and compare the results obtained with bovine CuZn SOD with those from SODs of other origins and carrying different active sites. MATERIALS AND METHODS: Recombinant human (rh), bovine, and equine CuZn SODs, as well as rh and E. coli Mn SODs, were studied before use with respect to specific activity and purity (HPLC, SDS-PAGE electrophoresis). Fluorescein isothiocyanate was covalently conjugated to the various SODs for study with high-resolution confocal scanning laser microscopy. Superoxide production by monocytes and neutrophils was measured with the cytochrome c assay. RESULTS: As expected from our experiments with flow cytometry, only rare neutrophils were labeled with FITC-SOD, even with the longest incubation time of 3 hr and the highest dose of 1500 units/ml. In addition, they showed a localized fluorescence pattern that was quite different from the diffuse punctate fluorescence pattern of monocytes. Lymphocytes were not labeled at all. The rapid binding to the cellular surface of monocytes was confirmed, and even after 5 min of preincubation, FITC-SOD was found on a small percentage of monocytes. This was correlated with a reduction in superoxide release after phorbolmyristate acetate (PMA) stimulation by 40%. An interesting finding was the perinuclear accumulation of the penetrated SOD after the longest pretreatment of 3 hr, suggesting a barrier against further progression. Indeed, through confocal microscopy we were able to exclude any fluorescence at the nuclear level. While the fluorescence labeling patterns and the kinetics of penetration were quite similar for bovine, equine, and rh CuZn SOD, the Mn SODs showed poor labeling, correlated with a weak inhibitory effect on cytochrome c reduction, which was not statistically significant. CONCLUSIONS: The rapid binding of native CuZn SODs on the surface of monocytes, leading to reduced superoxide release by these cells, explains the observation that beneficial effects of injected SOD lasted for months despite rapid clearance of the enzyme from the bloodstream, according to pharmacodynamic studies. The preferential binding to monocytes, in contrast to neutrophils, may play a role in chronic inflammatory diseases in which the monocytes are in an activated state. The differences in binding capacity between CuZn SODs and Mn SODs, correlated with different inhibitory effects of superoxide production by monocytes, may also have therapeutic significance.
Full text
PDF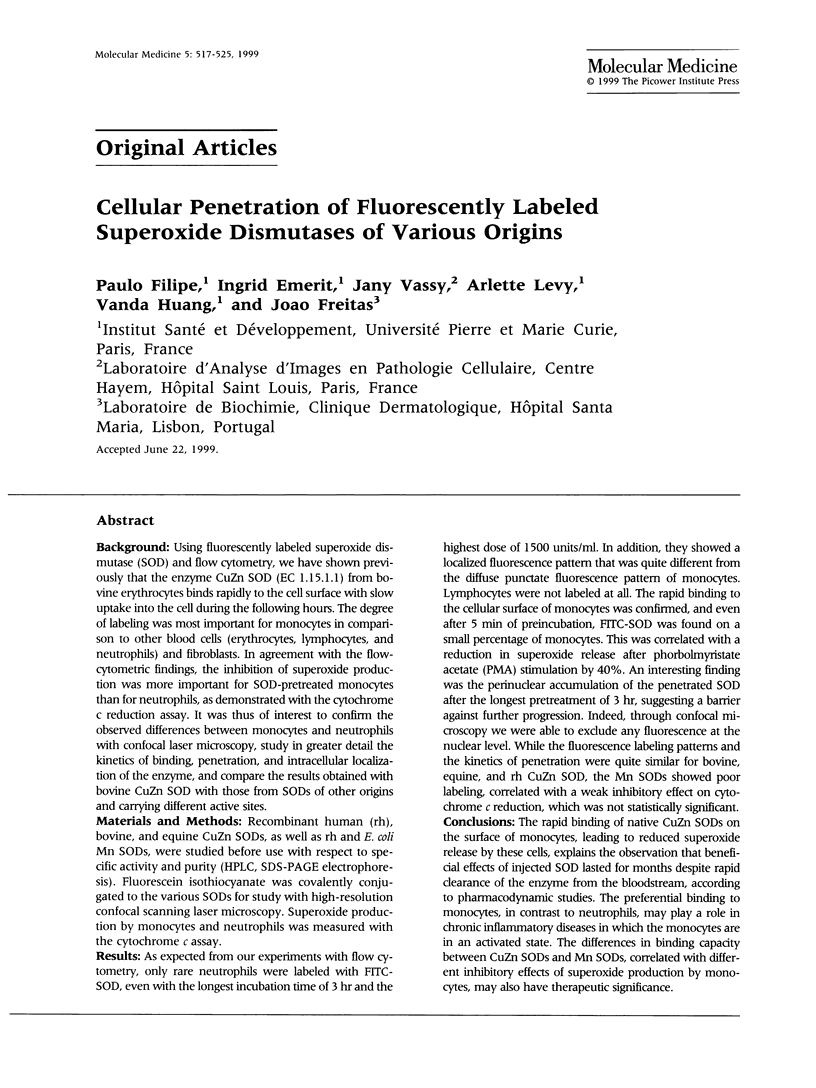
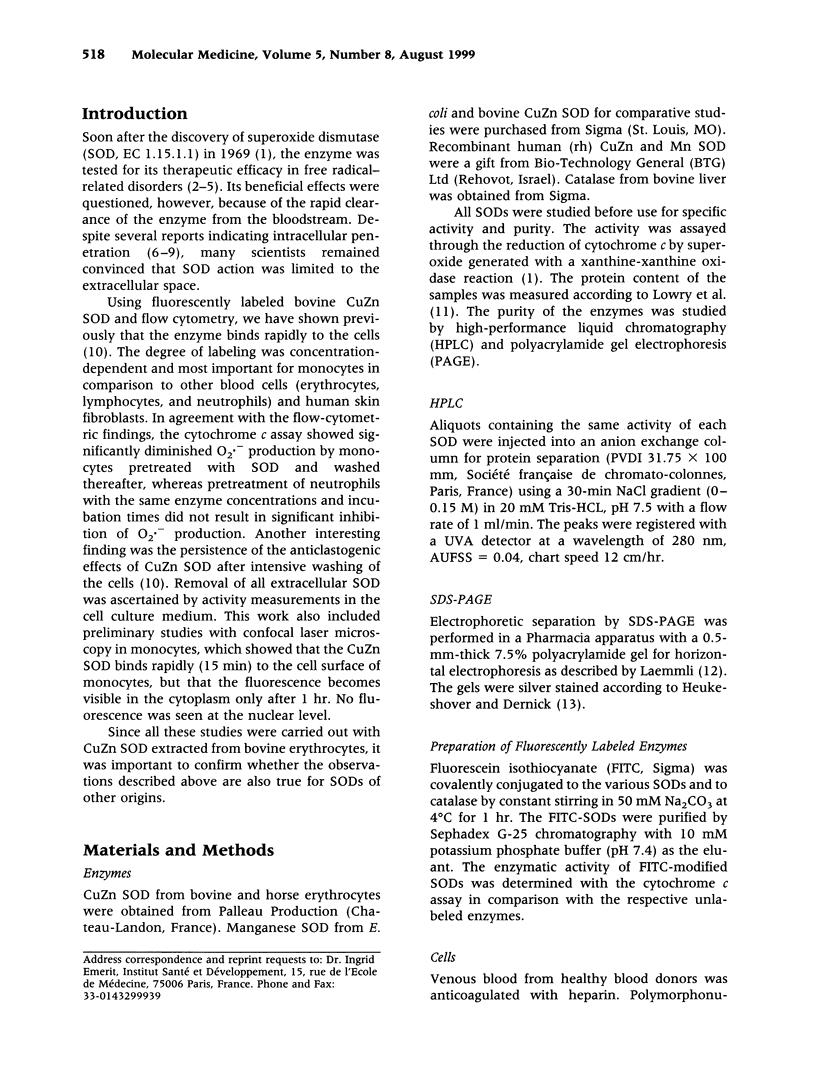
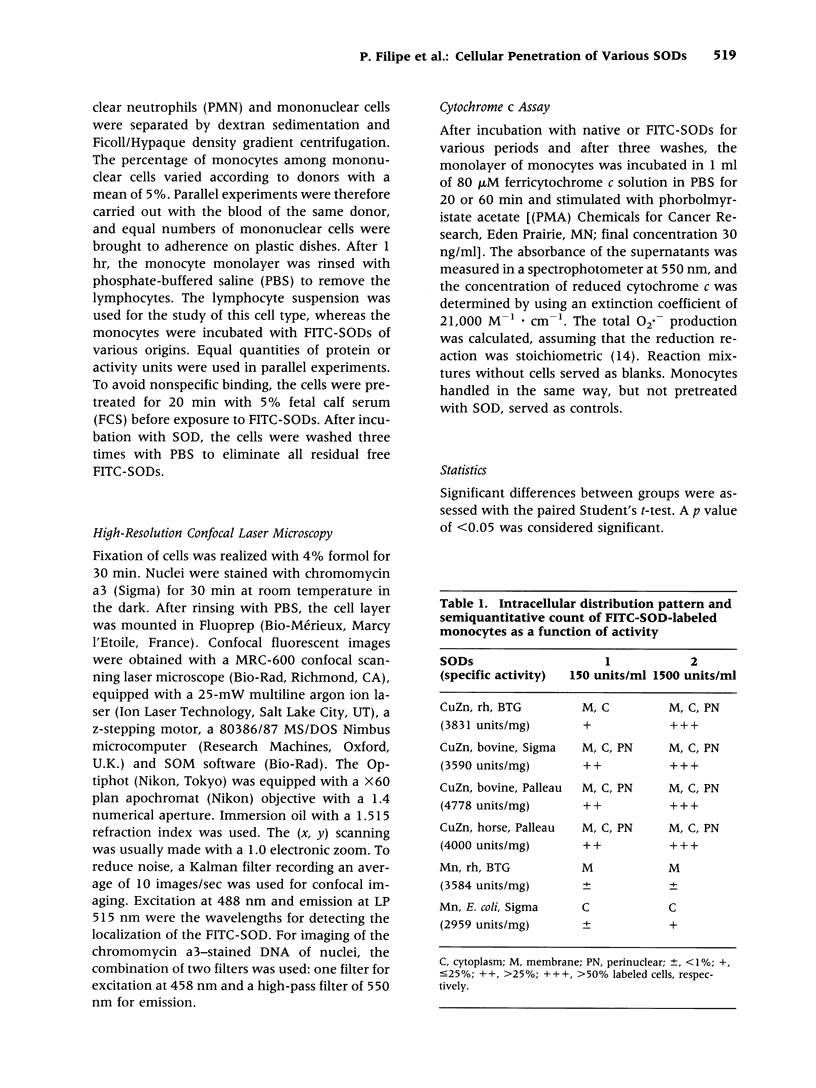
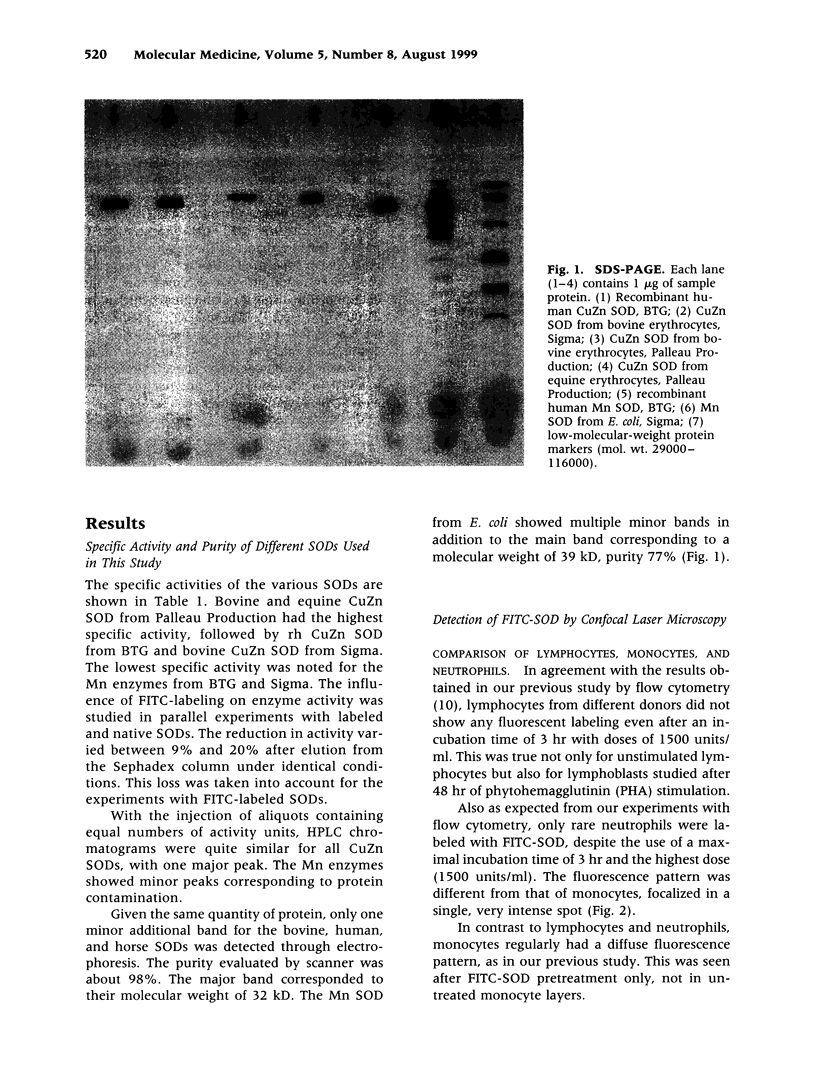
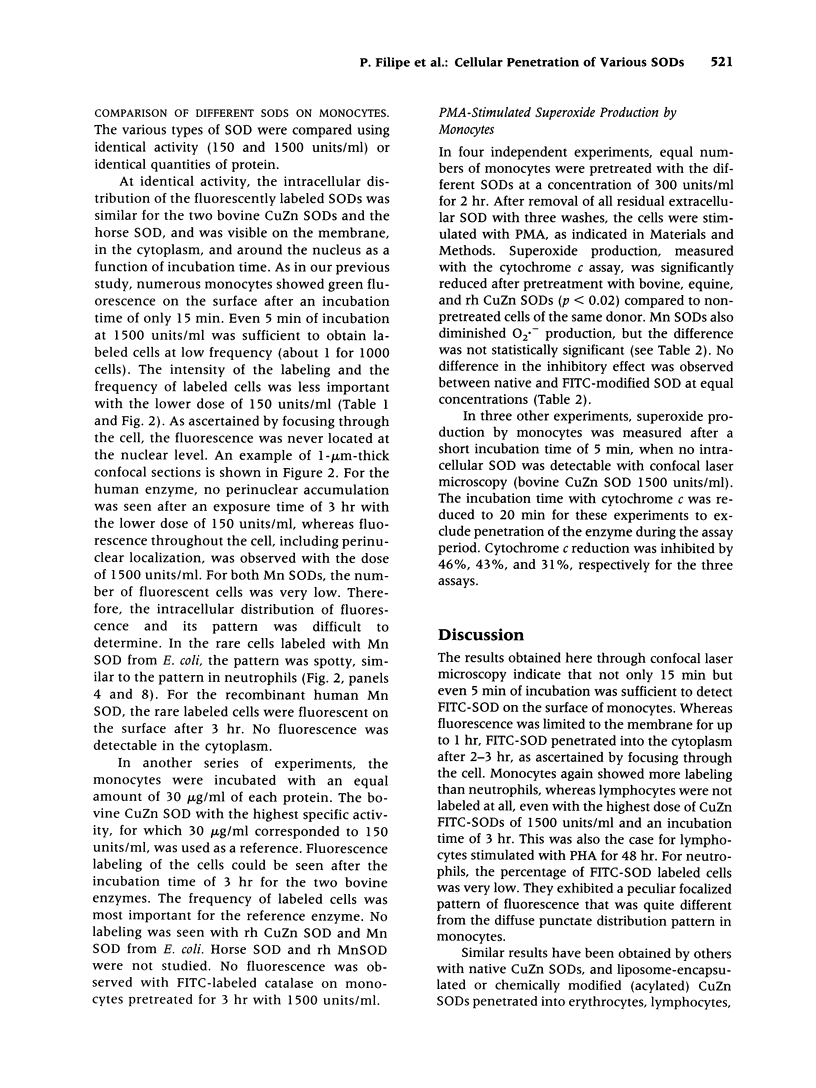
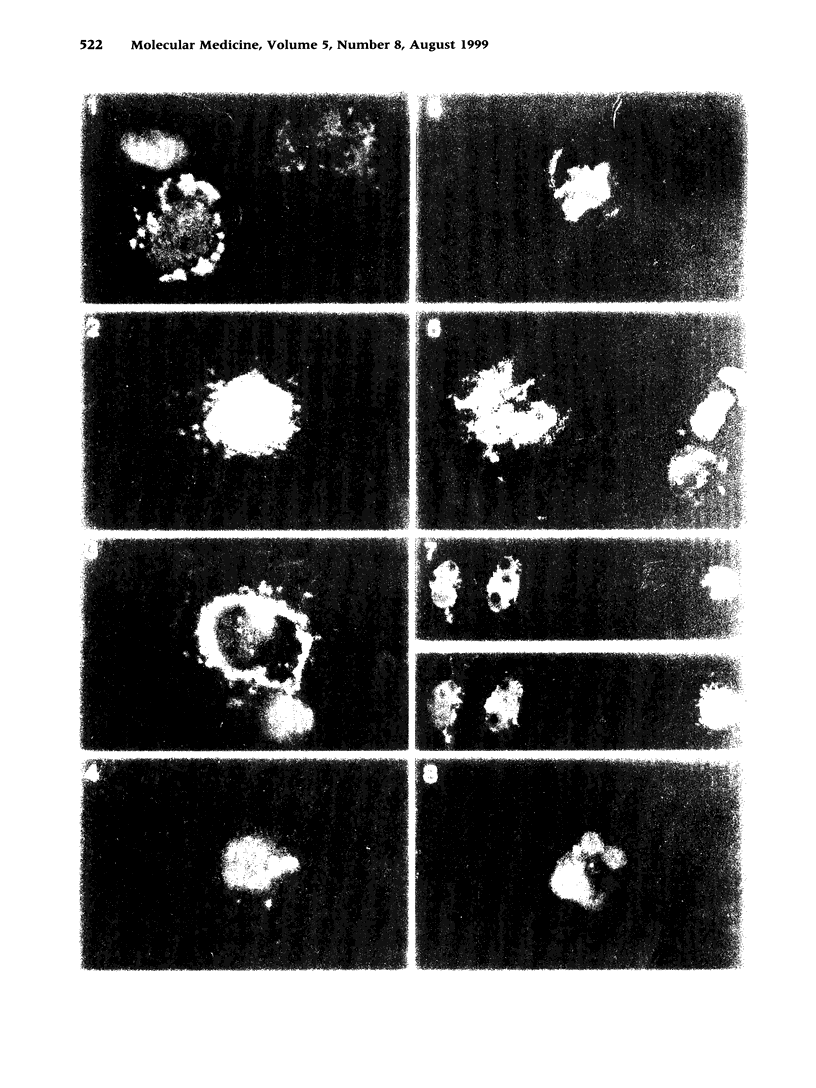
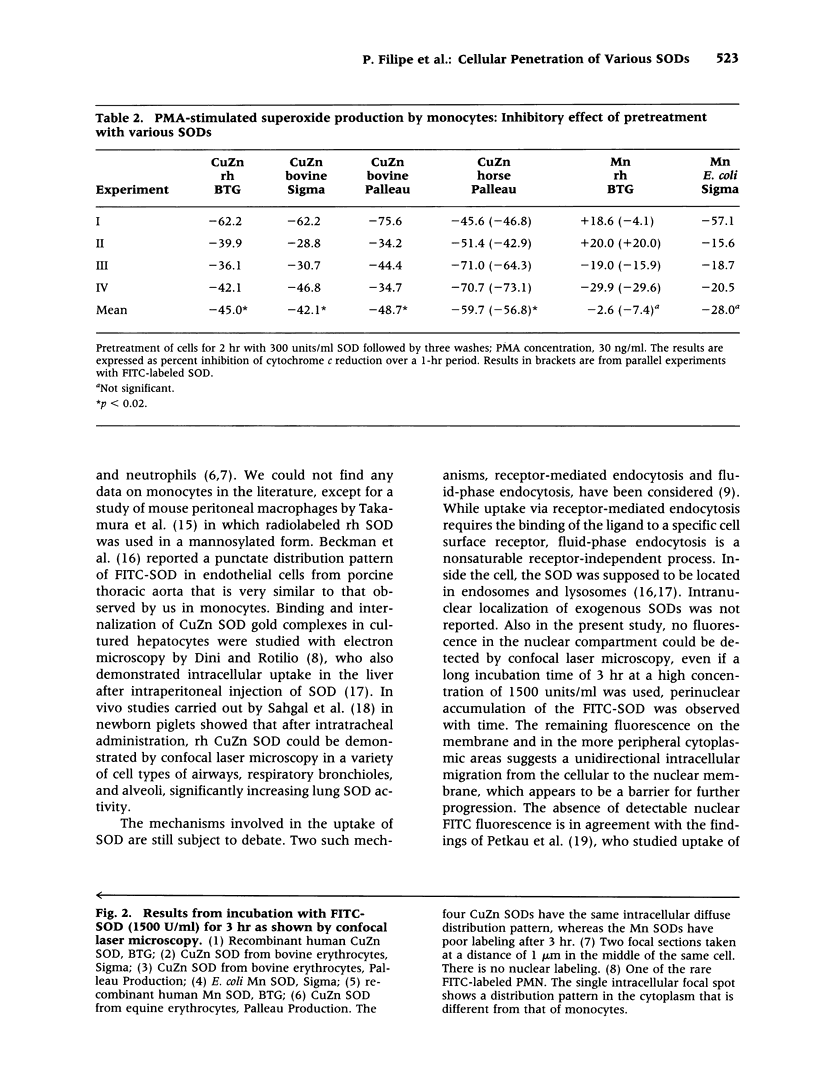
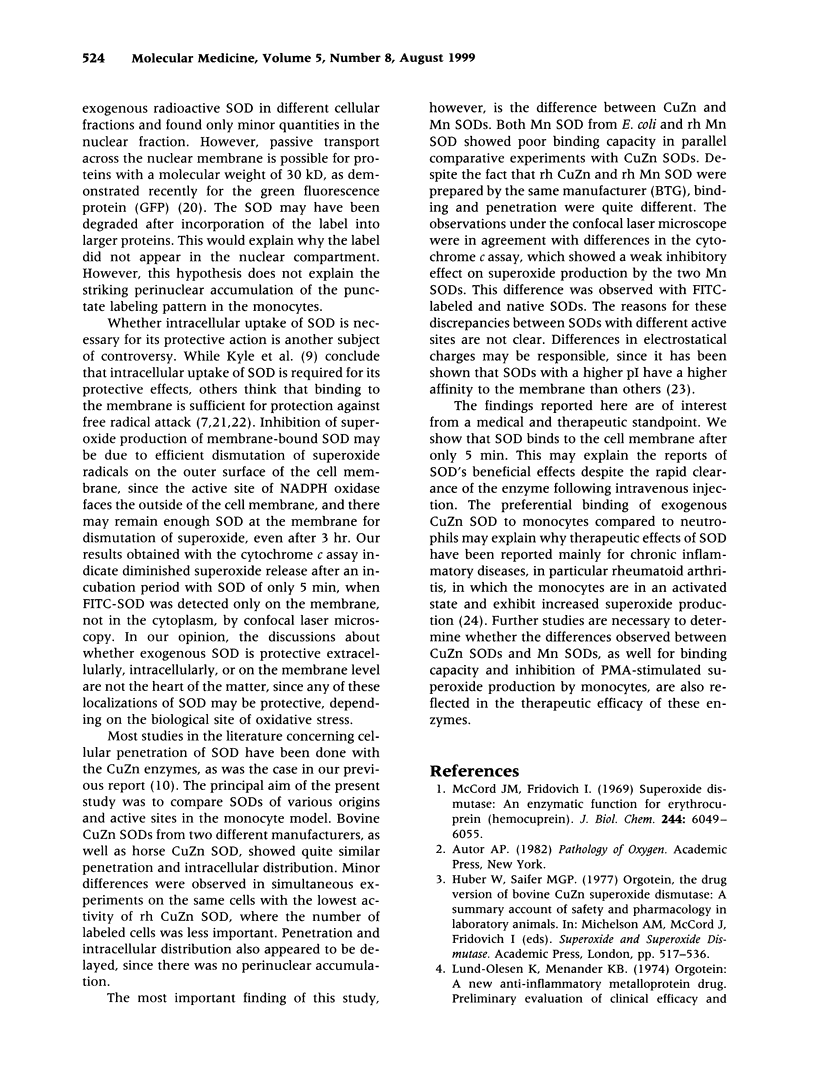
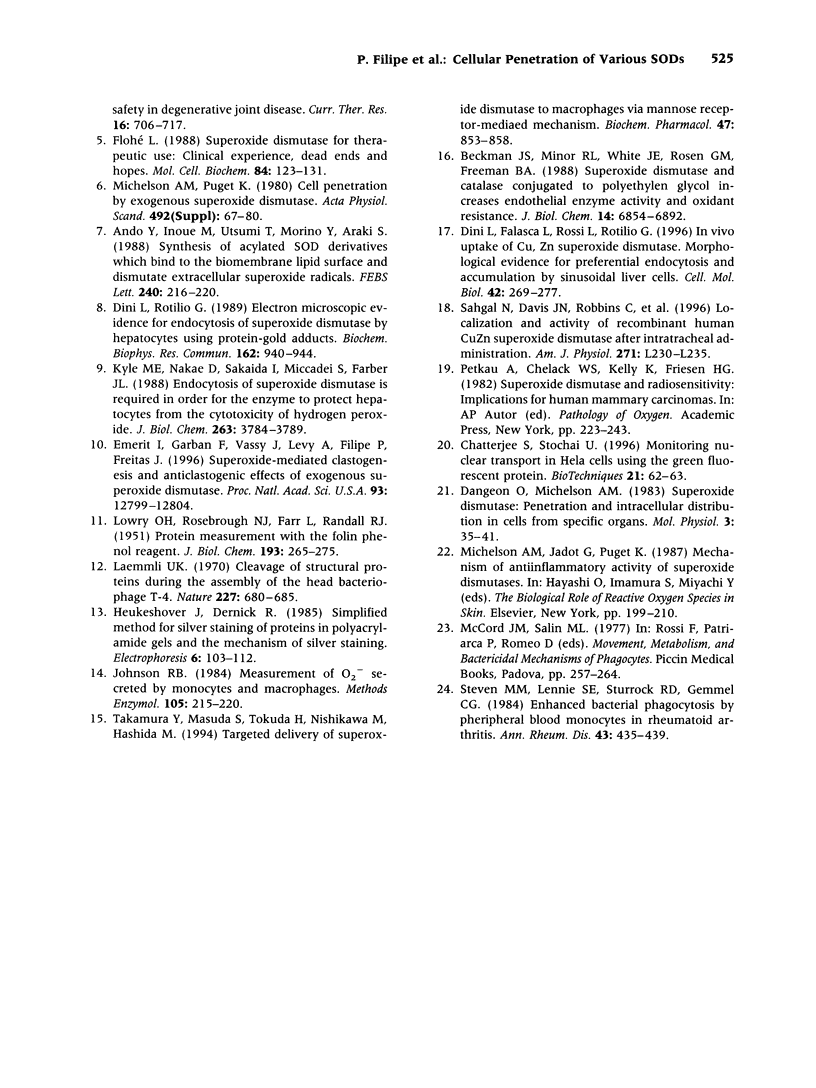
Images in this article
Selected References
These references are in PubMed. This may not be the complete list of references from this article.
- Ando Y., Inoue M., Utsumi T., Morino Y., Araki S. Synthesis of acylated SOD derivatives which bind to the biomembrane lipid surface and dismutate extracellular superoxide radicals. FEBS Lett. 1988 Nov 21;240(1-2):216–220. doi: 10.1016/0014-5793(88)80371-5. [DOI] [PubMed] [Google Scholar]
- Beckman J. S., Minor R. L., Jr, White C. W., Repine J. E., Rosen G. M., Freeman B. A. Superoxide dismutase and catalase conjugated to polyethylene glycol increases endothelial enzyme activity and oxidant resistance. J Biol Chem. 1988 May 15;263(14):6884–6892. [PubMed] [Google Scholar]
- Chatterjee S., Stochaj U. Monitoring nuclear transport in HeLa cells using the green fluorescent protein. Biotechniques. 1996 Jul;21(1):62–63. doi: 10.2144/96211bm13. [DOI] [PubMed] [Google Scholar]
- Dini L., Falasca L., Rossi L., Rotilio G. In vivo uptake of Cu, Zn superoxide dismutase. Morphological evidence for preferential endocytosis and accumulation by sinusoidal liver cells. Cell Mol Biol (Noisy-le-grand) 1996 Mar;42(2):269–277. [PubMed] [Google Scholar]
- Dini L., Rotilio G. Electron microscopic evidence for endocytosis of superoxide dismutase by hepatocytes using protein-gold adducts. Biochem Biophys Res Commun. 1989 Aug 15;162(3):940–944. doi: 10.1016/0006-291x(89)90763-8. [DOI] [PubMed] [Google Scholar]
- Emerit I., Garban F., Vassy J., Levy A., Filipe P., Freitas J. Superoxide-mediated clastogenesis and anticlastogenic effects of exogenous superoxide dismutase. Proc Natl Acad Sci U S A. 1996 Nov 12;93(23):12799–12804. doi: 10.1073/pnas.93.23.12799. [DOI] [PMC free article] [PubMed] [Google Scholar]
- Flohé L. Superoxide dismutase for therapeutic use: clinical experience, dead ends and hopes. Mol Cell Biochem. 1988 Dec;84(2):123–131. doi: 10.1007/BF00421046. [DOI] [PubMed] [Google Scholar]
- Kyle M. E., Nakae D., Sakaida I., Miccadei S., Farber J. L. Endocytosis of superoxide dismutase is required in order for the enzyme to protect hepatocytes from the cytotoxicity of hydrogen peroxide. J Biol Chem. 1988 Mar 15;263(8):3784–3789. [PubMed] [Google Scholar]
- LOWRY O. H., ROSEBROUGH N. J., FARR A. L., RANDALL R. J. Protein measurement with the Folin phenol reagent. J Biol Chem. 1951 Nov;193(1):265–275. [PubMed] [Google Scholar]
- Laemmli U. K. Cleavage of structural proteins during the assembly of the head of bacteriophage T4. Nature. 1970 Aug 15;227(5259):680–685. doi: 10.1038/227680a0. [DOI] [PubMed] [Google Scholar]
- McCord J. M., Fridovich I. Superoxide dismutase. An enzymic function for erythrocuprein (hemocuprein). J Biol Chem. 1969 Nov 25;244(22):6049–6055. [PubMed] [Google Scholar]
- Michelson A. M., Puget K. Cell penetration by exogenous superoxide dismutase. Acta Physiol Scand Suppl. 1980;492:67–80. [PubMed] [Google Scholar]
- Sahgal N., Davis J. M., Robbins C., Horowitz S., Langenback E. G., Perry R. H., Colflesh D., Tierney J., Simon S. R. Localization and activity of recombinant human CuZn superoxide dismutase after intratracheal administration. Am J Physiol. 1996 Aug;271(2 Pt 1):L230–L235. doi: 10.1152/ajplung.1996.271.2.L230. [DOI] [PubMed] [Google Scholar]
- Steven M. M., Lennie S. E., Sturrock R. D., Gemmell C. G. Enhanced bacterial phagocytosis by peripheral blood monocytes in rheumatoid arthritis. Ann Rheum Dis. 1984 Jun;43(3):435–439. doi: 10.1136/ard.43.3.435. [DOI] [PMC free article] [PubMed] [Google Scholar]
- Takakura Y., Masuda S., Tokuda H., Nishikawa M., Hashida M. Targeted delivery of superoxide dismutase to macrophages via mannose receptor-mediated mechanism. Biochem Pharmacol. 1994 Mar 2;47(5):853–858. doi: 10.1016/0006-2952(94)90485-5. [DOI] [PubMed] [Google Scholar]