Abstract
Polymers synthesized by heterotrophically growing (glucose as carbon source) cultures of Aphanocapsa 6714 were compared with polymers synthesized in photosynthetically grown cultures. Loss of photosystem II by dark incubation, or inhibition of light-grown cells with the photosystem II-specific inhibitor dichlorophenylmethylurea, caused an 80 to 90% reduction in the rate of lipid and total ribonucleic acid synthesis, and more than a 90% reduction in the rate of protein synthesis. In contrast, glycogen synthesis was reduced only about 50% in dark cells and less than 30% in dichlorphenylmethylurea-inhibited cells. After longer heterotrophic growth, glycogen became the major component, whereas in photosynthetically grown cultures protein was the major constituent. 14C (from 14CO2 and/or [14C]glucose) assimilated into protein by heterotrophically grown cells was found in amino acids in nearly the same proportions as in photosynthetically grown cells. Thus, routes of biosynthesis available to autotropic cells were also available to heterotrophic cultures, but the supply of carbon precursors to those pathways was greatly reduced. The limited biosynthesis in heterotrophic cells was not due to a limitation for cellular energy. The adenylates were maintained at nearly the same concentrations (and hence the energy charge also) as in photosynthetic cells. The concentration of reduced nicotinamide adenine dinucleotide phosphate was higher in heterotrophic (dark) cells than in photosynthetic cells. From rates of CO2 fixation and/or glycogen biosynthesis it was determined that stationary-phase cells expended approximately 835, 165, and less than 42 nmol of adenosine 5'-triphosphate per mg (dry weight) of algae per 30 min during photosynthetic, photoheterotrophic, and chemoheterotrophic metabolism, respectively. Analysis of the soluble metabolite pools in dark heterotrophic cultures by double-labeling experiments revealed rapid equilibration of 14C through the monophosphate pools, but much slower movement of label into the diphosphate pools of fructose-1,6-diphosphate and sedoheptulose-1,7-diphosphate. Carbon did flow into 3-phosphoglycerate in the dark; however, the initial rate was low and the concentration of this metabolite soon fell to an undetectable level. In photosynthetic cells, 14C quickly equilibrated throughout all the intermediates of the reductive pentose cycle, in particular, into 3-phosphoglycerate. Analysis of glucose-6-phosphate dehydrogenase in cell extracts showed that the enzyme was very sensitive to product inhibition by reduced nicotinamide adenine dinucleotide.
Full text
PDF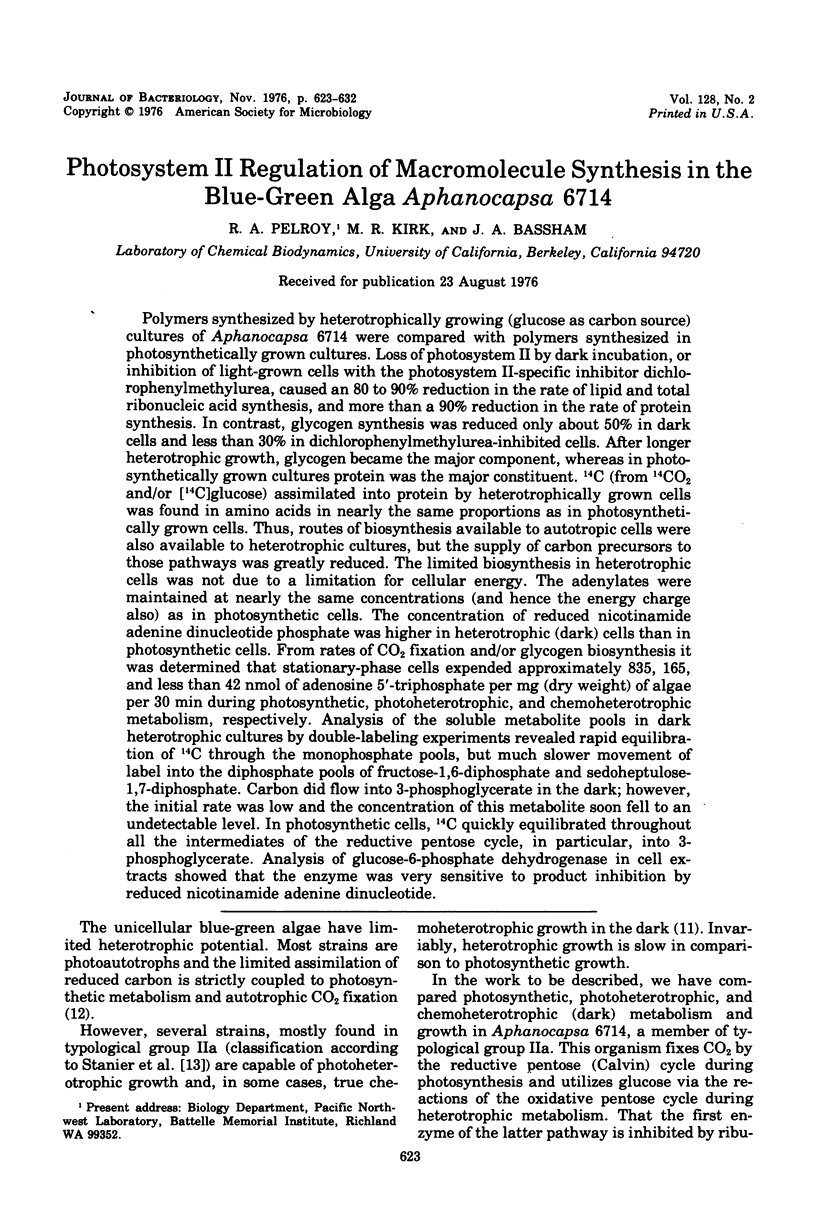
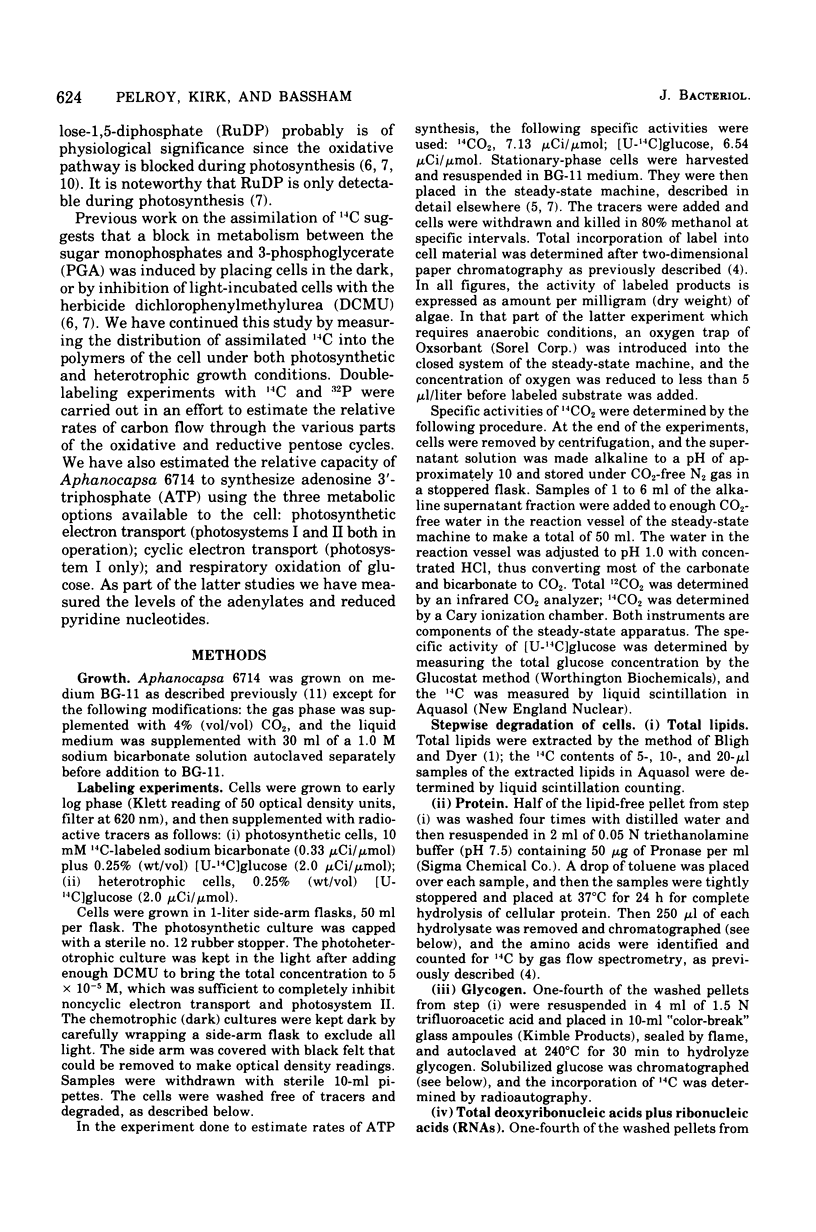
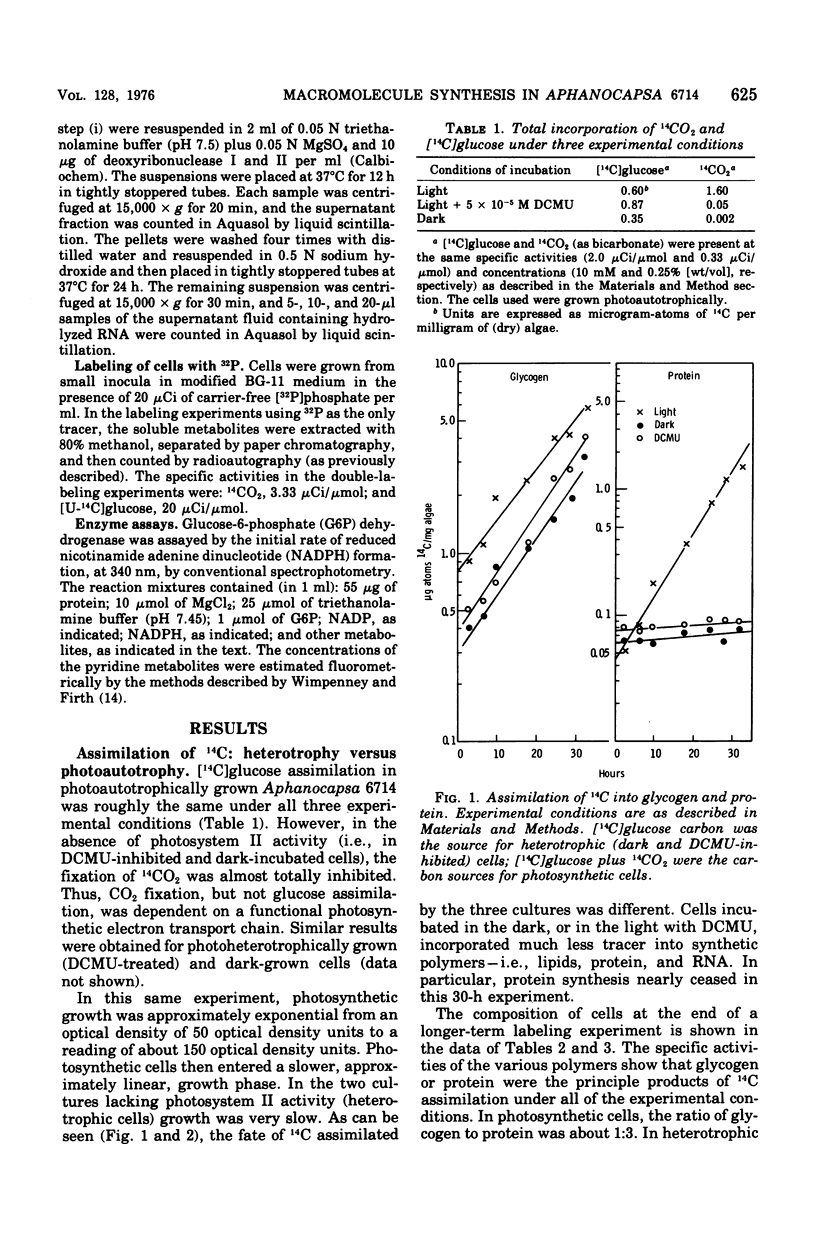
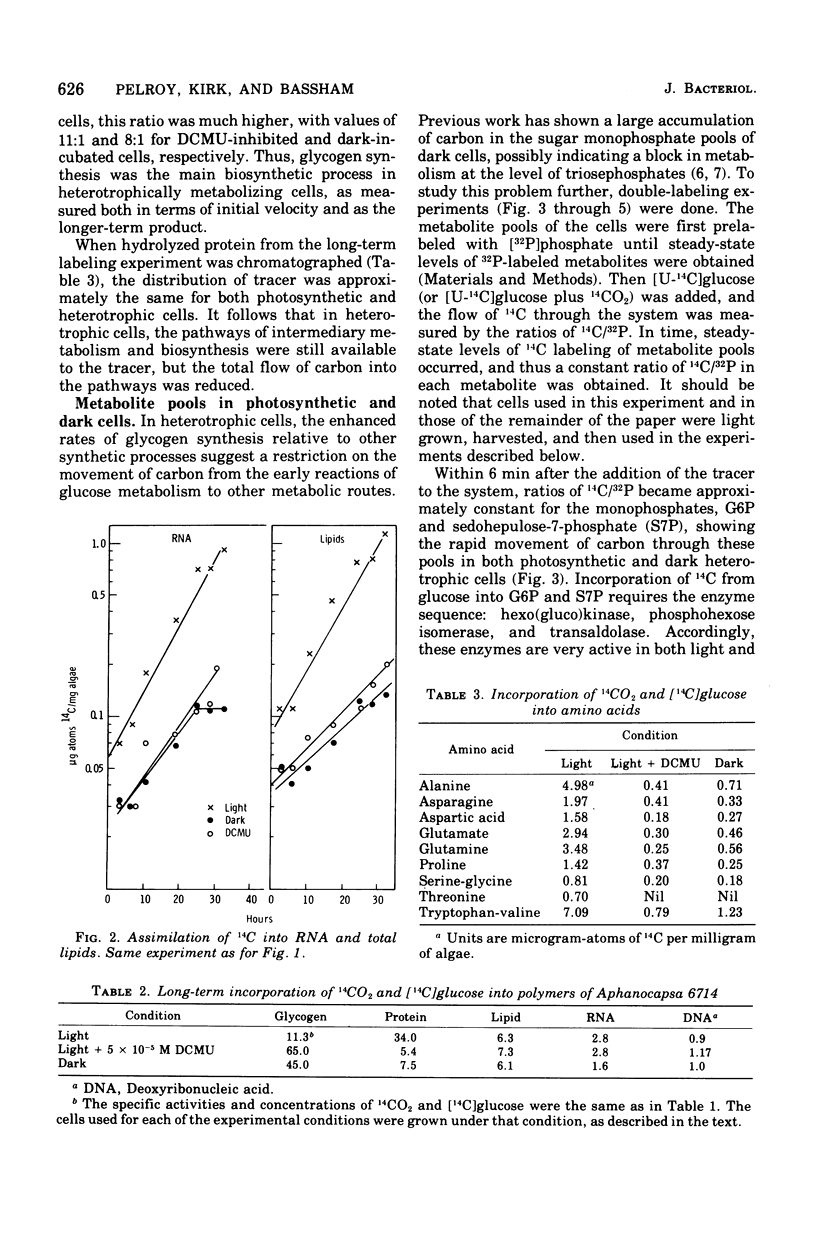
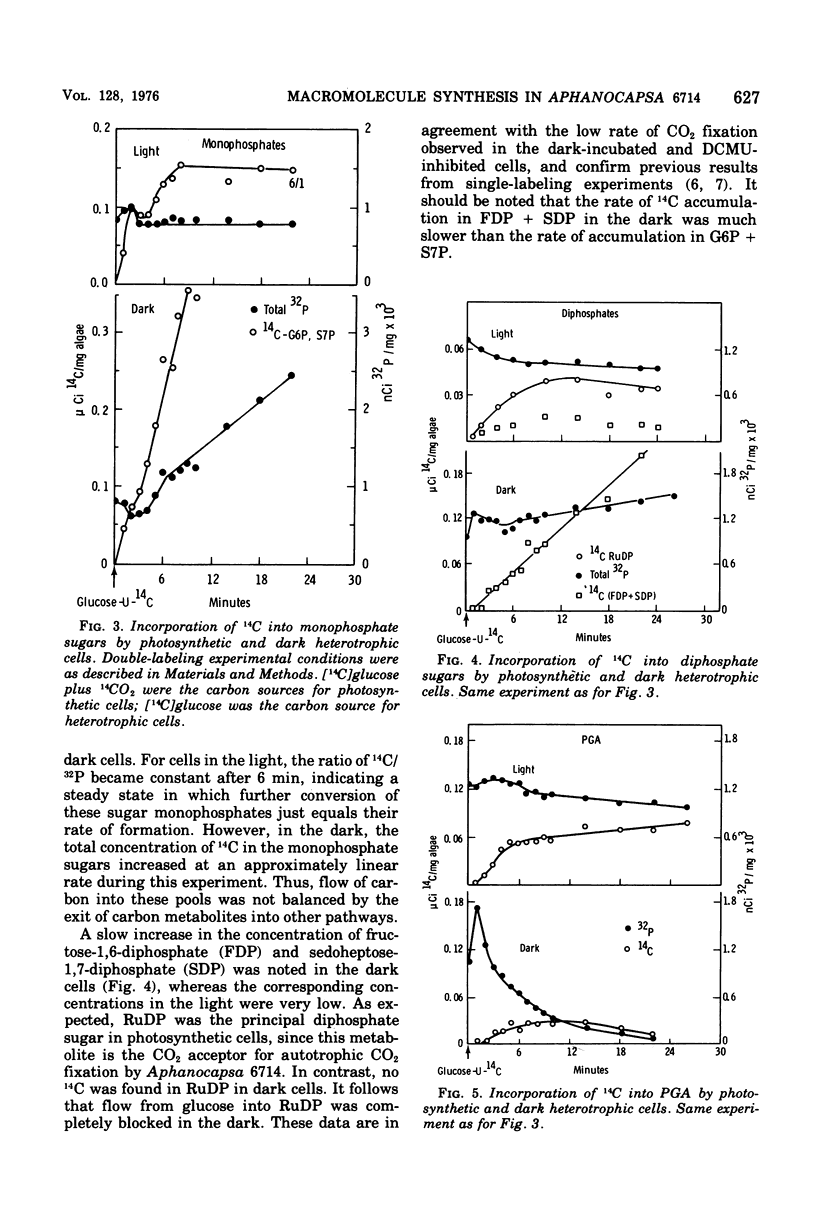
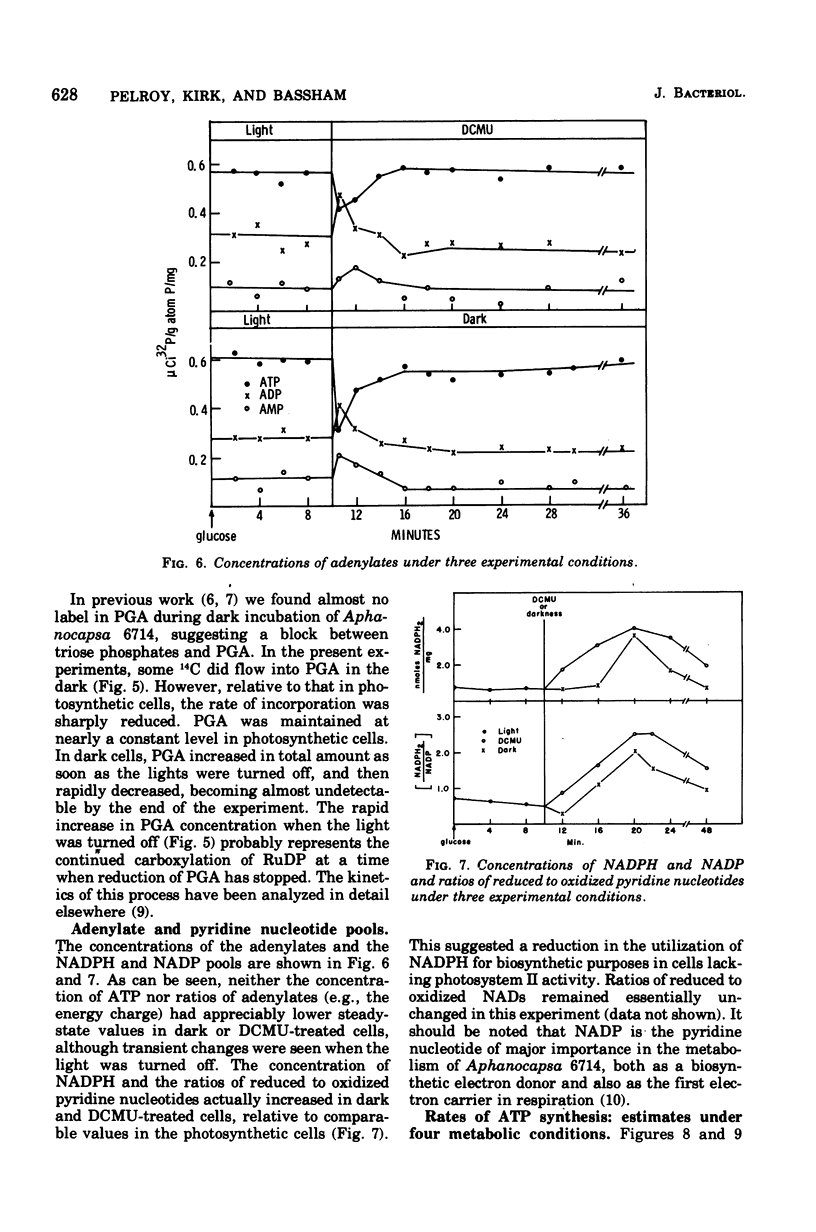
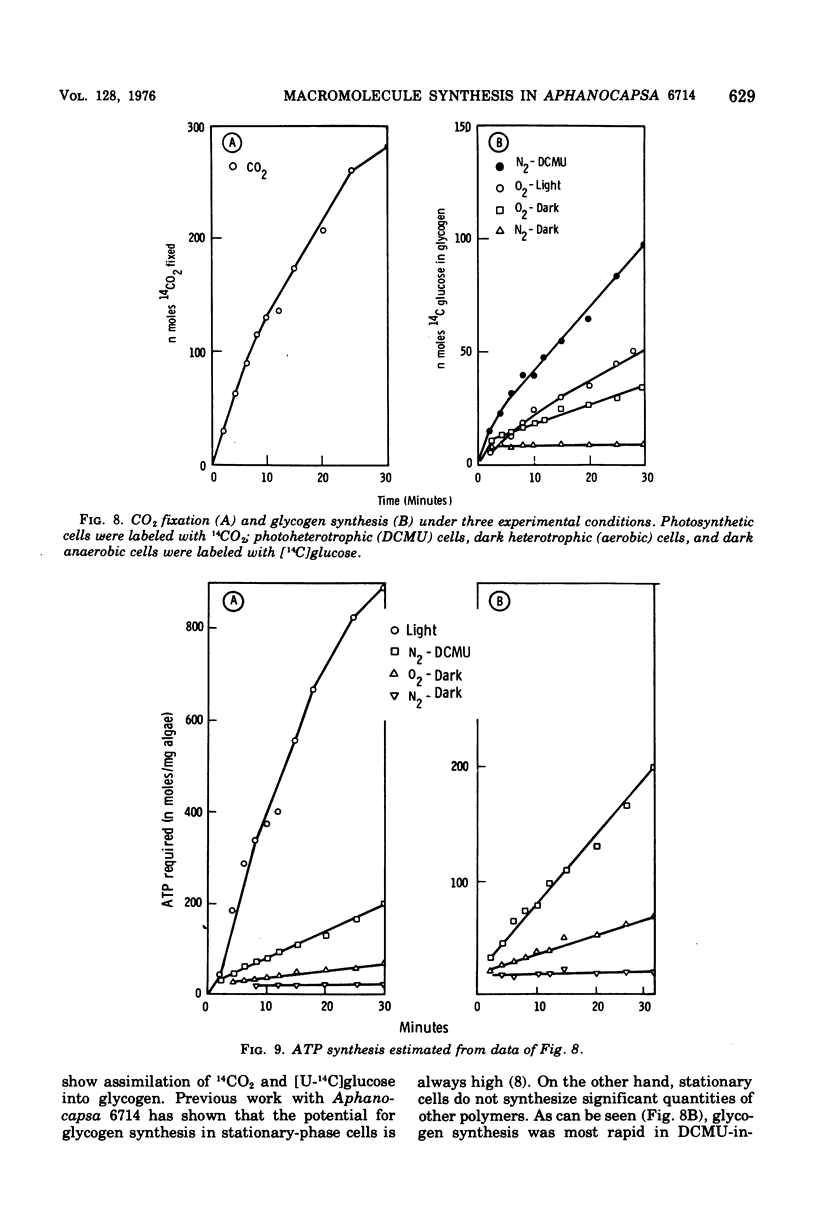
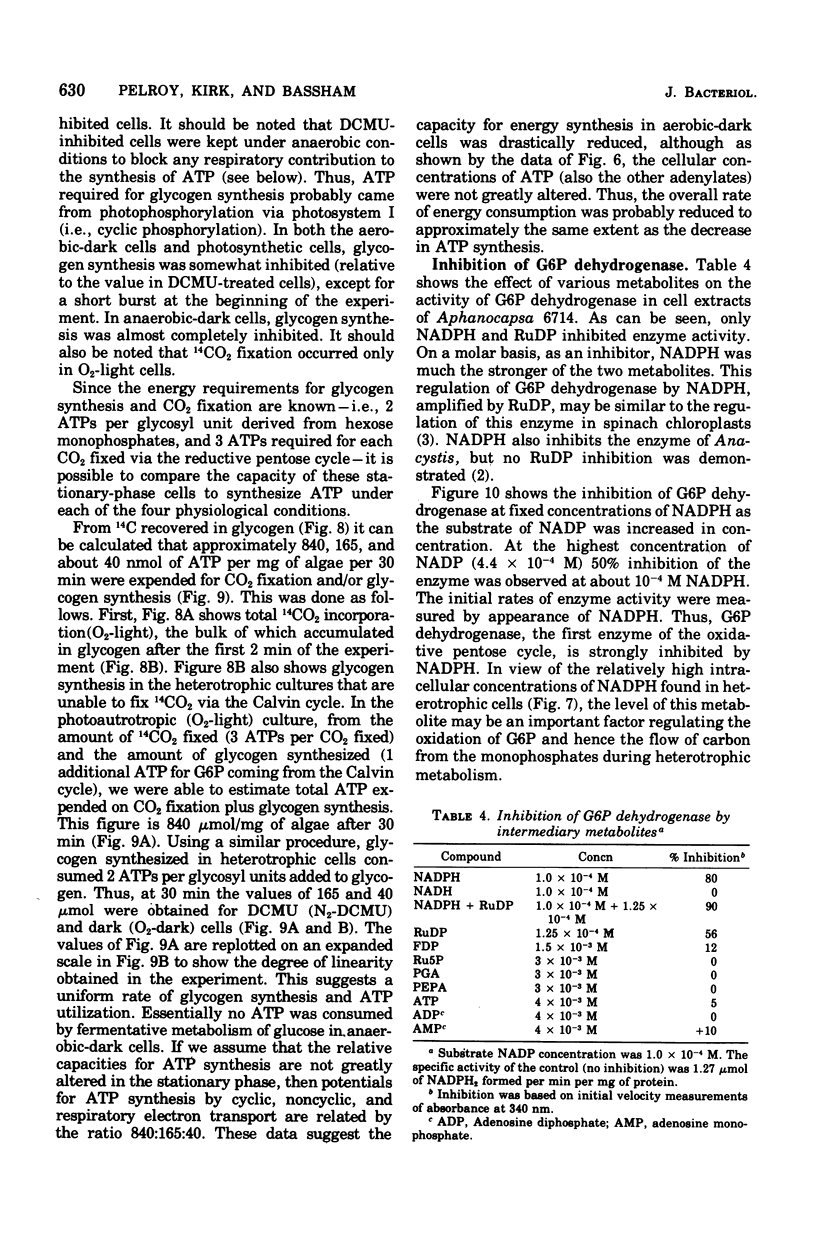
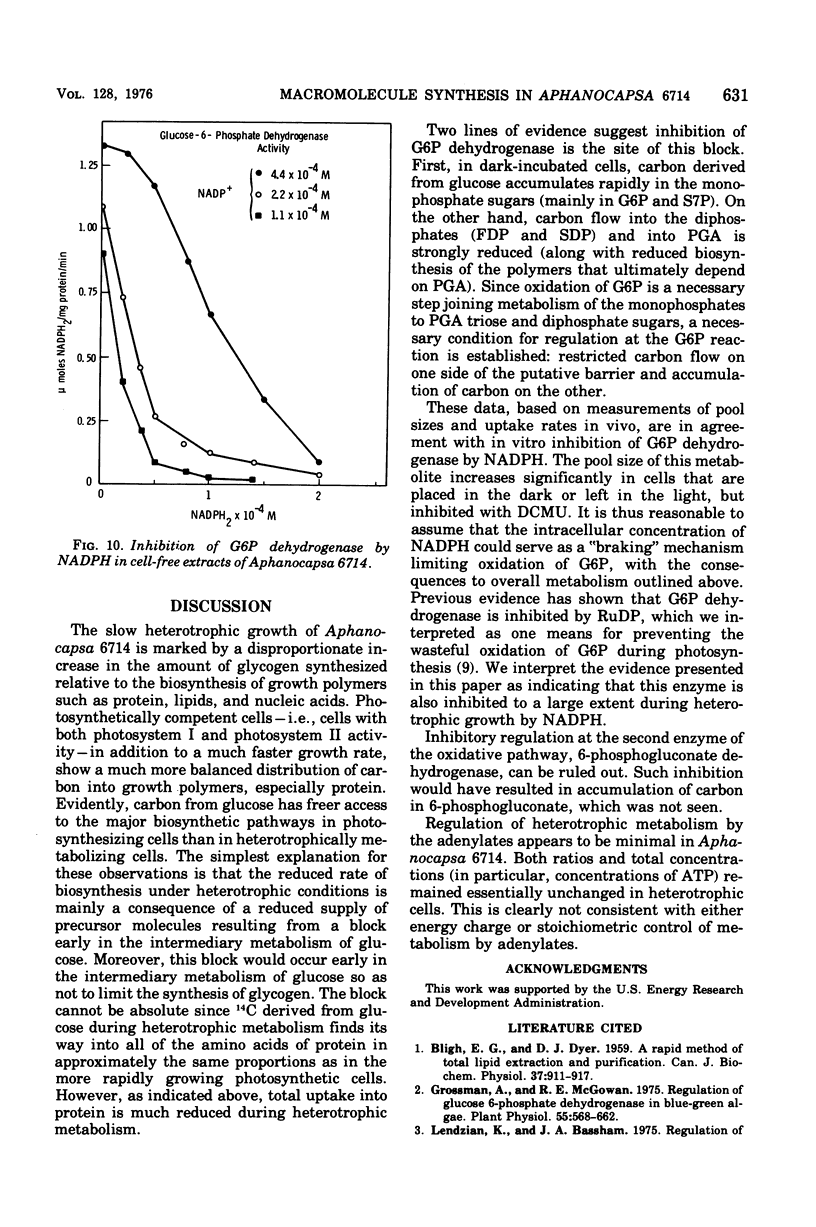
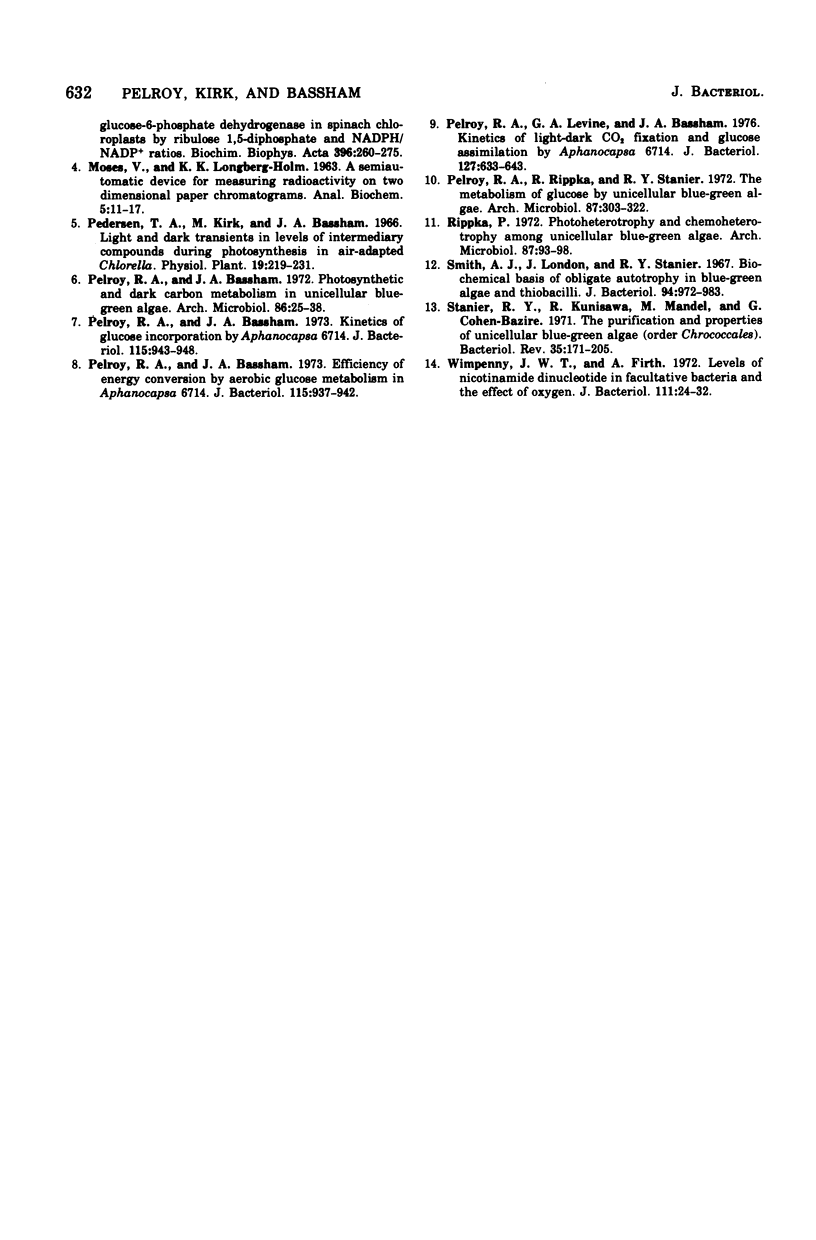
Selected References
These references are in PubMed. This may not be the complete list of references from this article.
- BLIGH E. G., DYER W. J. A rapid method of total lipid extraction and purification. Can J Biochem Physiol. 1959 Aug;37(8):911–917. doi: 10.1139/o59-099. [DOI] [PubMed] [Google Scholar]
- Grossman A., McGowan R. E. Regulation of glucose 6-phosphate dehydrogenase in blue-green algae. Plant Physiol. 1975 Apr;55(4):658–662. doi: 10.1104/pp.55.4.658. [DOI] [PMC free article] [PubMed] [Google Scholar]
- MOSES V., LONBERG-HOLM K. K. A semiautomatic device for measuring radioactivity on two-dimensional paper chromatograms. Anal Biochem. 1963 Jan;5:11–27. doi: 10.1016/0003-2697(63)90053-8. [DOI] [PubMed] [Google Scholar]
- Pelroy R. A., Bassham J. A. Efficiency of energy conversion by aerobic glucose metabolism in Aphancapsa 6714. J Bacteriol. 1973 Sep;115(3):937–942. doi: 10.1128/jb.115.3.937-942.1973. [DOI] [PMC free article] [PubMed] [Google Scholar]
- Pelroy R. A., Bassham J. A. Kinetics of glucose incorporation by Aphanocapsa 6714. J Bacteriol. 1973 Sep;115(3):943–948. doi: 10.1128/jb.115.3.943-948.1973. [DOI] [PMC free article] [PubMed] [Google Scholar]
- Pelroy R. A., Bassham J. A. Photosynthetic and dark carbon metabolism in unicellular blue-green algae. Arch Mikrobiol. 1972;86(1):25–38. doi: 10.1007/BF00412397. [DOI] [PubMed] [Google Scholar]
- Pelroy R. A., Levine G. A., Bassham J. A. Kinetics of light-dark CO2 fixation and glucose assimilation by Aphanocapsa 6714. J Bacteriol. 1976 Nov;128(2):633–643. doi: 10.1128/jb.128.2.633-643.1976. [DOI] [PMC free article] [PubMed] [Google Scholar]
- Pelroy R. A., Rippka R., Stanier R. Y. Metabolism of glucose by unicellular blue-green algae. Arch Mikrobiol. 1972;87(4):303–322. doi: 10.1007/BF00409131. [DOI] [PubMed] [Google Scholar]
- Smith A. J., London J., Stanier R. Y. Biochemical basis of obligate autotrophy in blue-green algae and thiobacilli. J Bacteriol. 1967 Oct;94(4):972–983. doi: 10.1128/jb.94.4.972-983.1967. [DOI] [PMC free article] [PubMed] [Google Scholar]
- Stanier R. Y., Kunisawa R., Mandel M., Cohen-Bazire G. Purification and properties of unicellular blue-green algae (order Chroococcales). Bacteriol Rev. 1971 Jun;35(2):171–205. doi: 10.1128/br.35.2.171-205.1971. [DOI] [PMC free article] [PubMed] [Google Scholar]
- Wimpenny J. W., Firth A. Levels of nicotinamide adenine dinucleotide and reduced nicotinamide adenine dinucleotide in facultative bacteria and the effect of oxygen. J Bacteriol. 1972 Jul;111(1):24–32. doi: 10.1128/jb.111.1.24-32.1972. [DOI] [PMC free article] [PubMed] [Google Scholar]