Abstract
Membrane vesicles of Escherichia coli prepared by osmotic lysis of lysozyme ethylenediaminetetracetate (EDTA) spheroplasts have approximately 60% of the total membrane-bound reduced nicotinamide adenine dinucleotide (NADH) dehydrogenase (ED 1.6.99.3) and Mg2+-adenosine triphosphatase (ATPase) (EC 3.6.1.3) activities exposed on the outer surface of the inner membrane. Absorption of these vesicles with antiserum prepared against the purified soluble Mg2+-ATPase resulted in agglutination of approximately 95% of the inner membrane vesicles, as determined by dehydrogenase activity, and about 50% of the total membrane protein. The unagglutinated vesicles lacked all dehydrogenase activity and may consist of outer membrane. Lysozyme-EDTA vesicles actively transported calcium ion, using either NADH or adenosine 5'-triphosphate (ATP) as energy source. However, neither D-lactate nor reduced phenazine methosulfate energized calcium uptake, suggesting that the observed calcium uptake was not due to a small population of everted vesicles. Transport of calcium driven by either NADH or ATP was inhibited by simultaneous addition of D-lactate or reduced phenazine methosulfate. Proline transport driven by D-lactate oxidation was inhibited by either NADH oxidation or ATP hydrolysis. These results suggest that the portion of the total population of vesicles capable of active transport, i.e., the inner membrane vesicles, are functionally a homogeneous population but cannot be categorized as either right-side-out or everted, since activities normally associated with only one side of the inner membrane can be found on both sides of the membrane of these vesicles. Moreover, the data indicate that oxidation of NADH or hydrolysis of ATP by externally localized NADH dehydrogenase or Mg2+-ATPase establishes a protonmotive force of the opposite polarity from that established through D-lactate oxidation.
Full text
PDF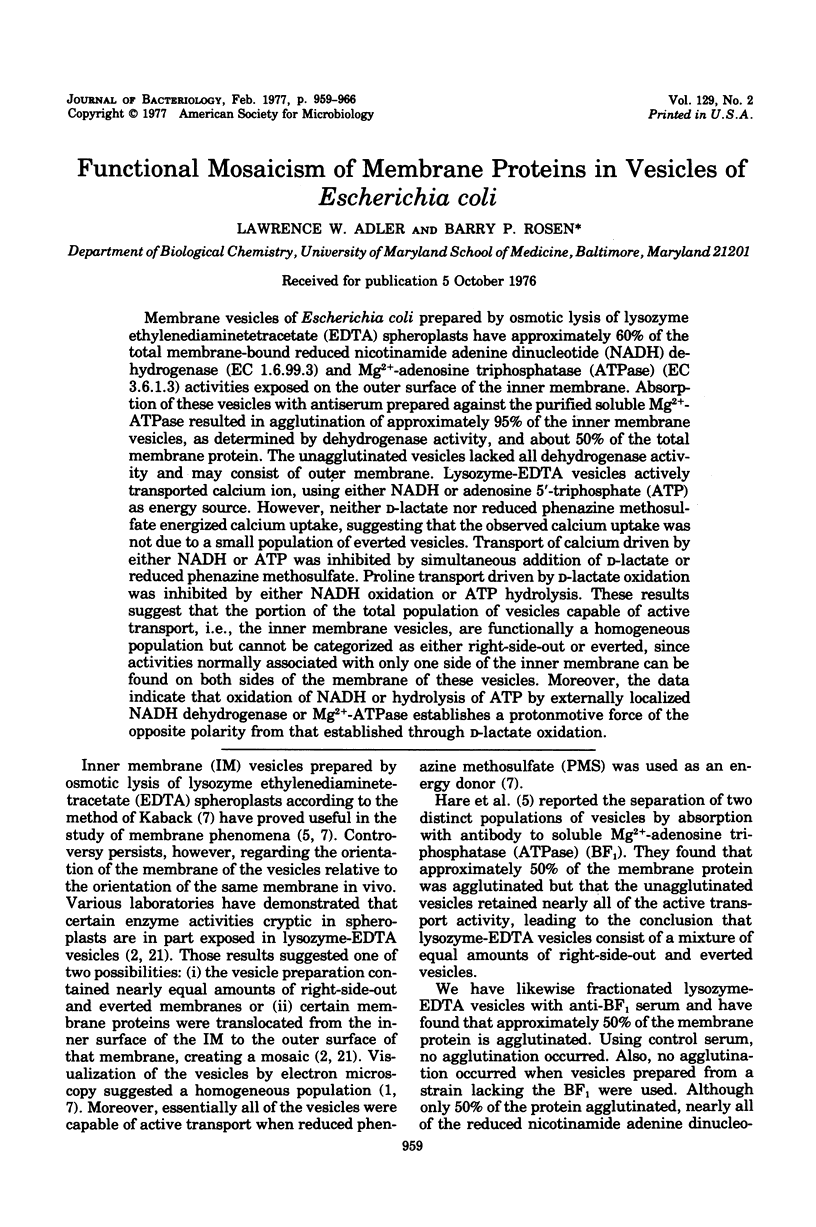
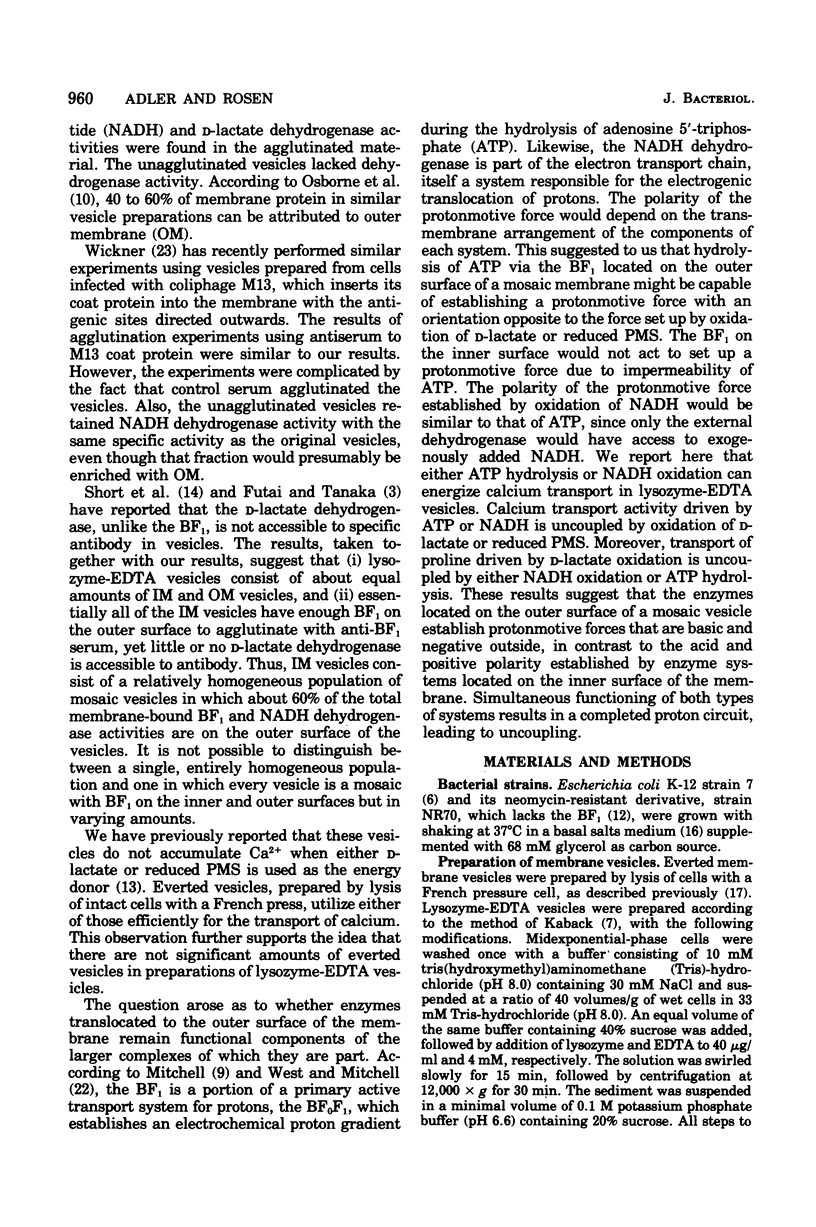
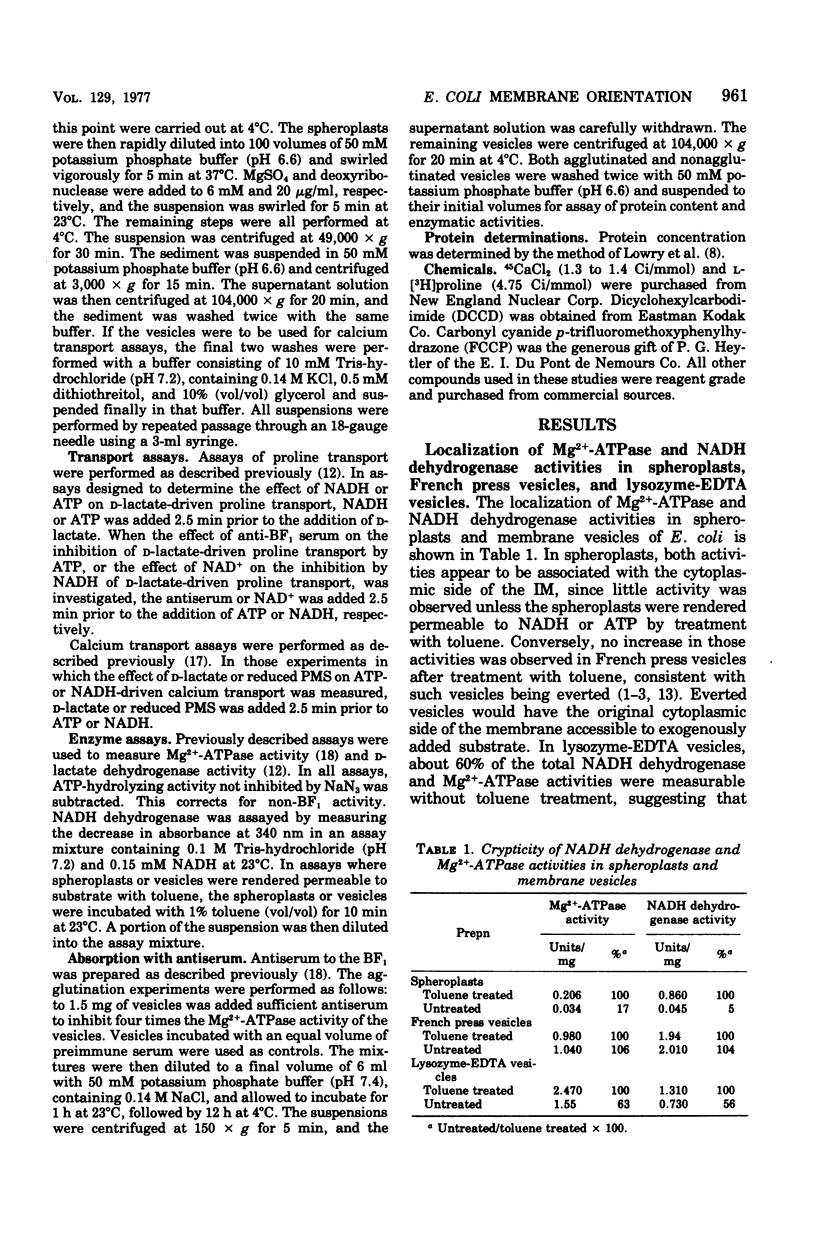
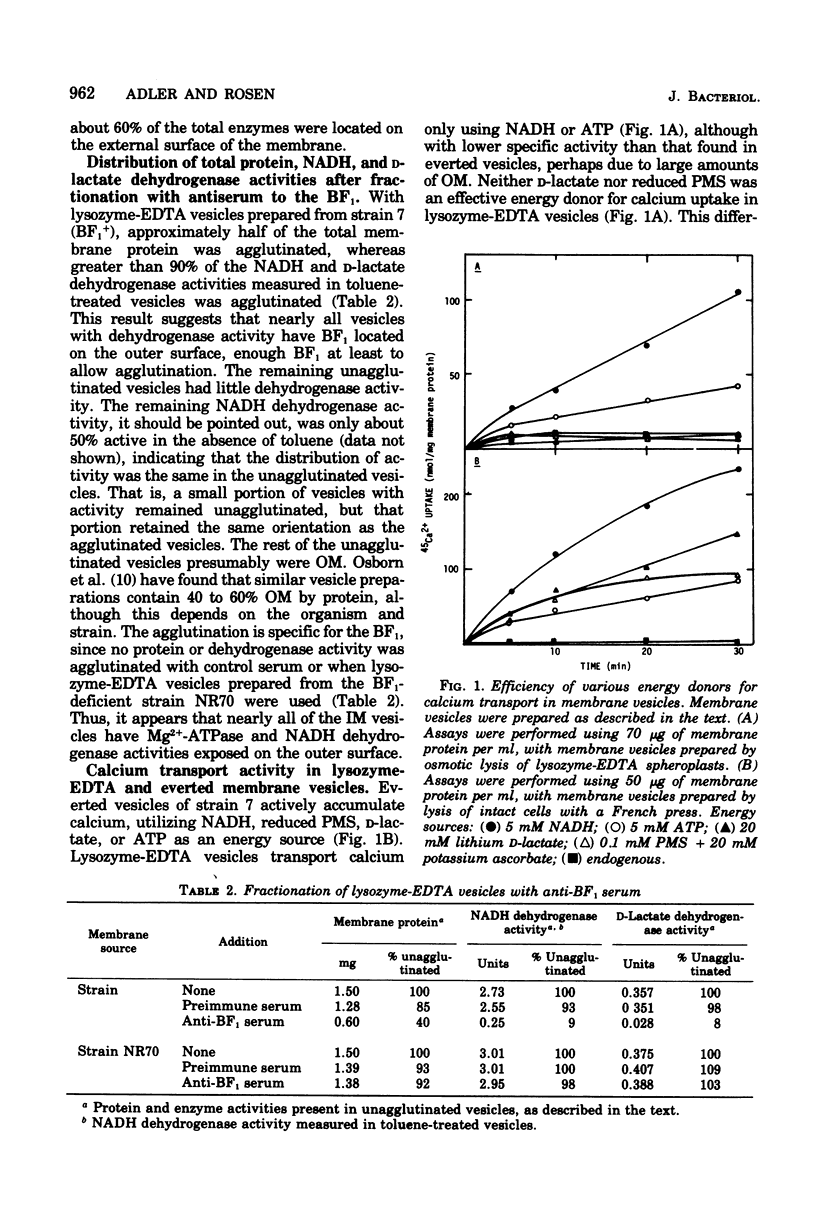
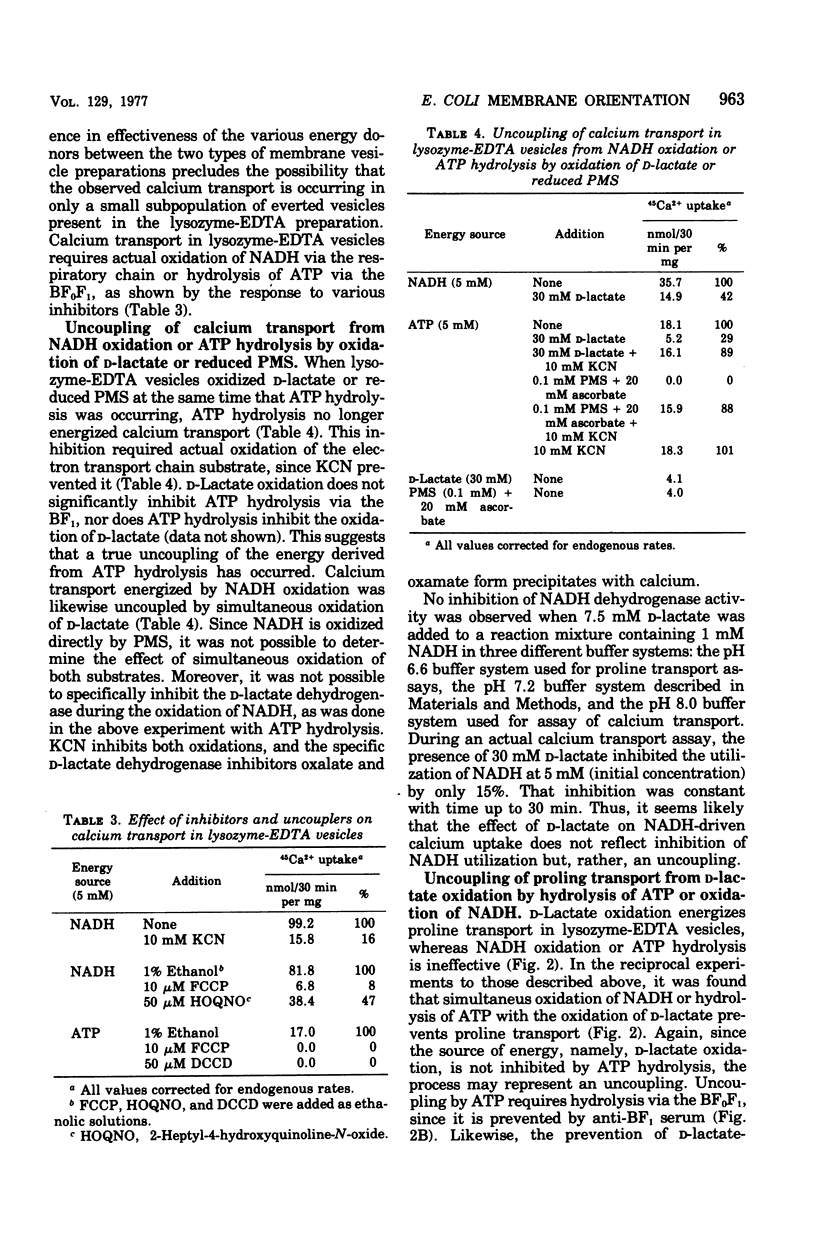
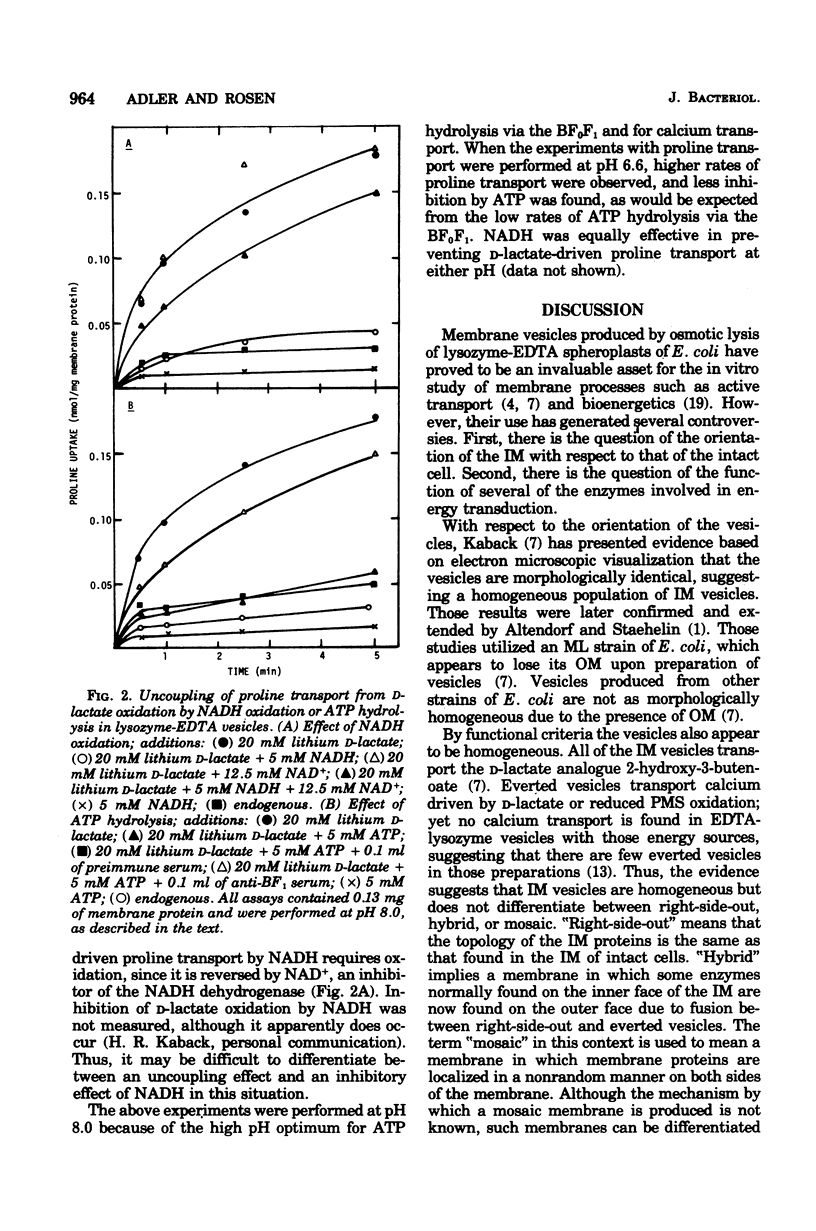
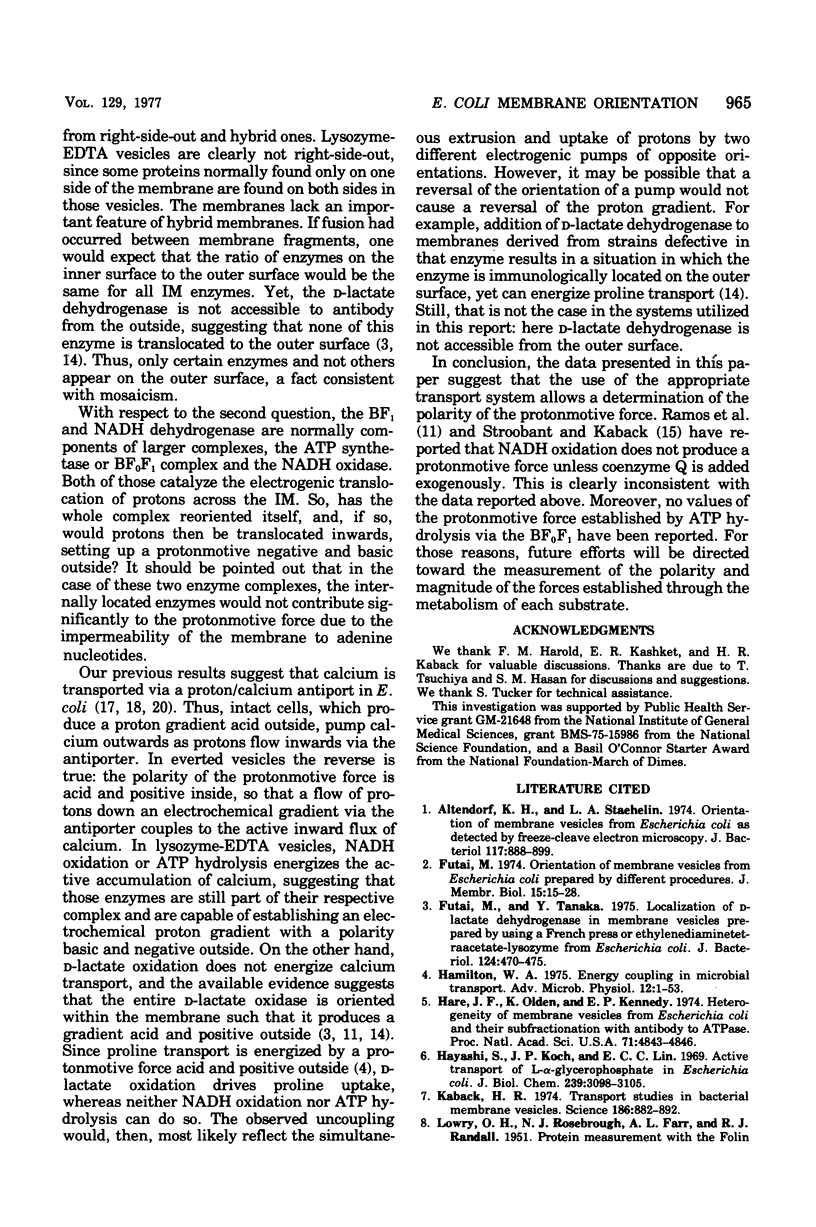
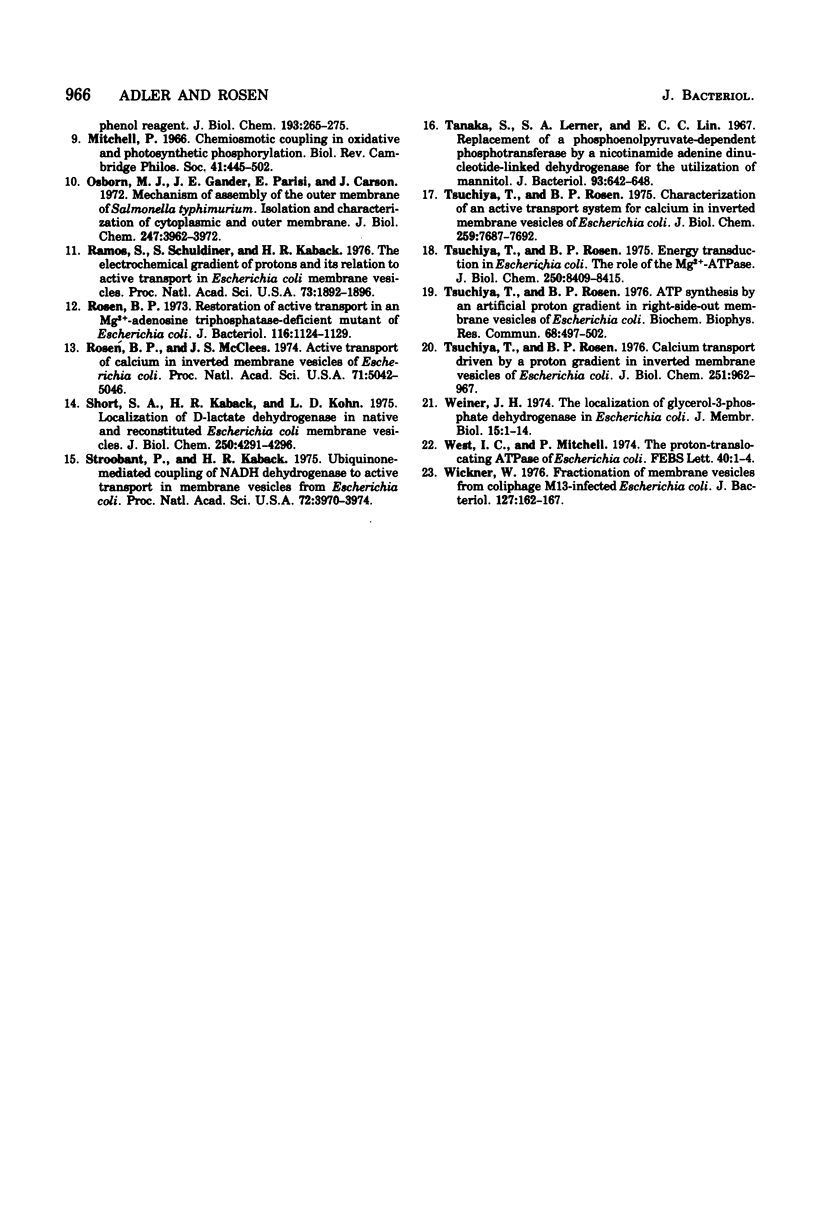
Selected References
These references are in PubMed. This may not be the complete list of references from this article.
- Altendorf K. H., Staehelin L. A. Orientation of membrane vesicles from Escherichia coli as detected by freeze-cleave electron microscopy. J Bacteriol. 1974 Feb;117(2):888–899. doi: 10.1128/jb.117.2.888-899.1974. [DOI] [PMC free article] [PubMed] [Google Scholar]
- Futai M. Orientation of membrane vesicles from Escherichia coli prepared by different procedures. J Membr Biol. 1974;15(1):15–28. doi: 10.1007/BF01870079. [DOI] [PubMed] [Google Scholar]
- Futai M., Tanaka Y. Localization of D-lactate dehydrogenase in membrane vesicles prepared by using a french press or ethylenediaminetetraacetate-lysozyme from Escherichia coli. J Bacteriol. 1975 Oct;124(1):470–475. doi: 10.1128/jb.124.1.470-475.1975. [DOI] [PMC free article] [PubMed] [Google Scholar]
- HAYASHI S., KOCH J. P., LIN E. C. ACTIVE TRANSPORT OF L-ALPHA-GLYCEROPHOSPHATE IN ESCHERICHIA COLI. J Biol Chem. 1964 Sep;239:3098–3105. [PubMed] [Google Scholar]
- Hare J. F., Olden K., Kennedy E. P. Heterogeneity of membrane vesicles from Escherichia coli and their subfractionation with antibody to ATPase. Proc Natl Acad Sci U S A. 1974 Dec;71(12):4843–4846. doi: 10.1073/pnas.71.12.4843. [DOI] [PMC free article] [PubMed] [Google Scholar]
- Kaback H. R. Transport studies in bacterial membrane vesicles. Science. 1974 Dec 6;186(4167):882–892. doi: 10.1126/science.186.4167.882. [DOI] [PubMed] [Google Scholar]
- LOWRY O. H., ROSEBROUGH N. J., FARR A. L., RANDALL R. J. Protein measurement with the Folin phenol reagent. J Biol Chem. 1951 Nov;193(1):265–275. [PubMed] [Google Scholar]
- Mitchell P. Chemiosmotic coupling in oxidative and photosynthetic phosphorylation. Biol Rev Camb Philos Soc. 1966 Aug;41(3):445–502. doi: 10.1111/j.1469-185x.1966.tb01501.x. [DOI] [PubMed] [Google Scholar]
- Osborn M. J., Gander J. E., Parisi E., Carson J. Mechanism of assembly of the outer membrane of Salmonella typhimurium. Isolation and characterization of cytoplasmic and outer membrane. J Biol Chem. 1972 Jun 25;247(12):3962–3972. [PubMed] [Google Scholar]
- Ramos S., Schuldiner S., Kaback H. R. The electrochemical gradient of protons and its relationship to active transport in Escherichia coli membrane vesicles. Proc Natl Acad Sci U S A. 1976 Jun;73(6):1892–1896. doi: 10.1073/pnas.73.6.1892. [DOI] [PMC free article] [PubMed] [Google Scholar]
- Rosen B. P., McClees J. S. Active transport of calcium in inverted membrane vesicles of Escherichia coli. Proc Natl Acad Sci U S A. 1974 Dec;71(12):5042–5046. doi: 10.1073/pnas.71.12.5042. [DOI] [PMC free article] [PubMed] [Google Scholar]
- Rosen B. P. Restoration of active transport in an Mg2+-adenosine triphosphatase-deficient mutant of Escherichia coli. J Bacteriol. 1973 Dec;116(3):1124–1129. doi: 10.1128/jb.116.3.1124-1129.1973. [DOI] [PMC free article] [PubMed] [Google Scholar]
- Short S. A., Kaback H. R., Kohn L. D. Localization of D-lactate dehydrogenase in native and reconstituted Escherichia coli membrane vesicles. J Biol Chem. 1975 Jun 10;250(11):4291–4296. [PubMed] [Google Scholar]
- Stroobant P., Kaback H. R. Ubiquinone-mediated coupling of NADH dehydrogenase to active transport in membrane vesicles from Escherichia coli. Proc Natl Acad Sci U S A. 1975 Oct;72(10):3970–3974. doi: 10.1073/pnas.72.10.3970. [DOI] [PMC free article] [PubMed] [Google Scholar]
- Tanaka S., Lerner S. A., Lin E. C. Replacement of a phosphoenolpyruvate-dependent phosphotransferase by a nicotinamide adenine dinucleotide-linked dehydrogenase for the utilization of mannitol. J Bacteriol. 1967 Feb;93(2):642–648. doi: 10.1128/jb.93.2.642-648.1967. [DOI] [PMC free article] [PubMed] [Google Scholar]
- Tsuchiya T., Rosen B. P. ATP synthesis by an artificial proton gradient in right-side-out membrane vesicles of Escherichia coli. Biochem Biophys Res Commun. 1976 Jan 26;68(2):497–502. doi: 10.1016/0006-291x(76)91173-6. [DOI] [PubMed] [Google Scholar]
- Tsuchiya T., Rosen B. P. Calcium transport driven by a proton gradient and inverted membrane vesicles of Escherichia coli. J Biol Chem. 1976 Feb 25;251(4):962–967. [PubMed] [Google Scholar]
- Tsuchiya T., Rosen B. P. Characterization of an active transport system for calcium in inverted membrane vesicles of Escherichia coli. J Biol Chem. 1975 Oct 10;250(19):7687–7692. [PubMed] [Google Scholar]
- Tsuchiya T., Rosen B. P. Energy transduction in Escherichia coli. The role of the Mg2+ATPase. J Biol Chem. 1975 Nov 10;250(21):8409–8415. [PubMed] [Google Scholar]
- Weiner J. H. The localization of glycerol-3-phosphate dehydrogenase in Escherichia coli. J Membr Biol. 1974;15(1):1–14. doi: 10.1007/BF01870078. [DOI] [PubMed] [Google Scholar]
- West I. C., Mitchell P. The proton-translocating ATPase of Escherichia coli. FEBS Lett. 1974 Mar 15;40(1):1–4. doi: 10.1016/0014-5793(74)80880-x. [DOI] [PubMed] [Google Scholar]
- Wickner W. Fractionation of membrane vesicles from coliphage M13-infected Escherichia coli. J Bacteriol. 1976 Jul;127(1):162–167. doi: 10.1128/jb.127.1.162-167.1976. [DOI] [PMC free article] [PubMed] [Google Scholar]