Abstract
It was confirmed that 2,3-dihydroxy-p-cumate is a substrate for ring cleavage in Pseudomonas putida PL-W after growth with p-cymene or p-cumate. This compound was oxidized to pyruvate, acetaldehyde, isobutyrate, and carbon dioxide by extracts of cells, and these products appear in equimolar amounts. The transient appearance of compounds and 2,3-dihydroxy-p-cumate to a yellow intermediate (lambda max, 345 nm) without decarboxylation. Extracts of the benzene nucleus; this is followed by decarboxylation to give the 393-nm species, which gives rise to isobutyrate, acetaldehyde, and pyruvate by the hydrolytic route of meta cleavage of catechols, via 4-hydroxy-2-oxovalerate. This was confirmed with a mutant of P. putida PL-RF-1 that was unable to grow with p-cymene (or p-cumate) but was able to oxidize both compounds AND 2,3-DIHYDROXY-P-CUMATE TO A YELLOW INTERMEDIATE (LAMBDA MAX, 345 NM) WITHOUT DECARBOXYLATION. Extrats of P. putida PL-W (wild type) or a revertant of the mutant PL-RF-1 catalyzed the decarboxlation of the 345-nm intermediate with transient formation of the compound that absorbed at 393 nm. The substrate specificities of the 3,4-dioxygenative ring cleavage enzyme, and the decarboxylase were determined in crude extracts of P. putida PL-W and Pseudomonas fluorescens 007. It was conclude that 3,4-dioxygenative cleavage and decarboxylation are sequential enzyme-catalyzed reactions common to both P. putida and P. fluorescens for the oxidation of 2,3-dihydroxybenzoates. Unlike P. putida PL-W, which exclusively use the hydrolase branch, P. fluorescens 007 uses the dehydrogenase branch of the meta pathways that diverge after ring cleavage and later converge at oxoenate intermediates.
Full text
PDF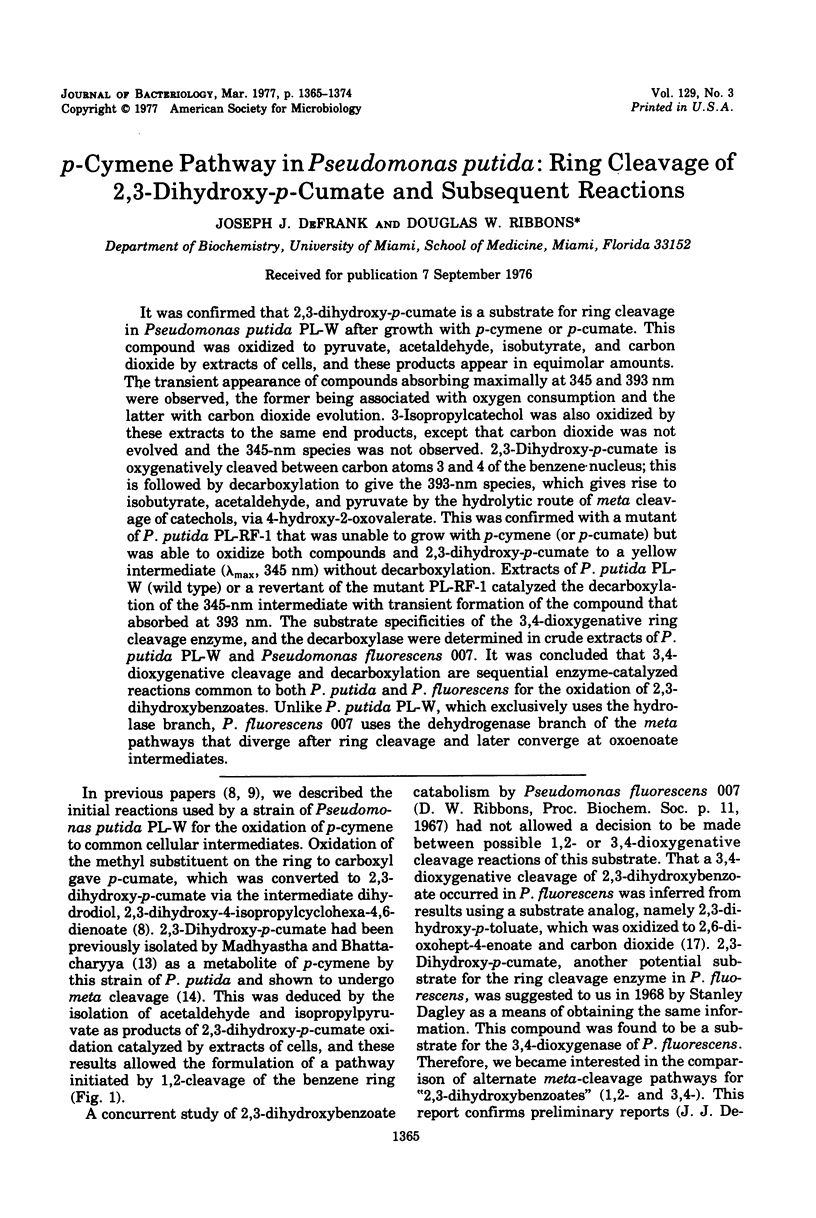
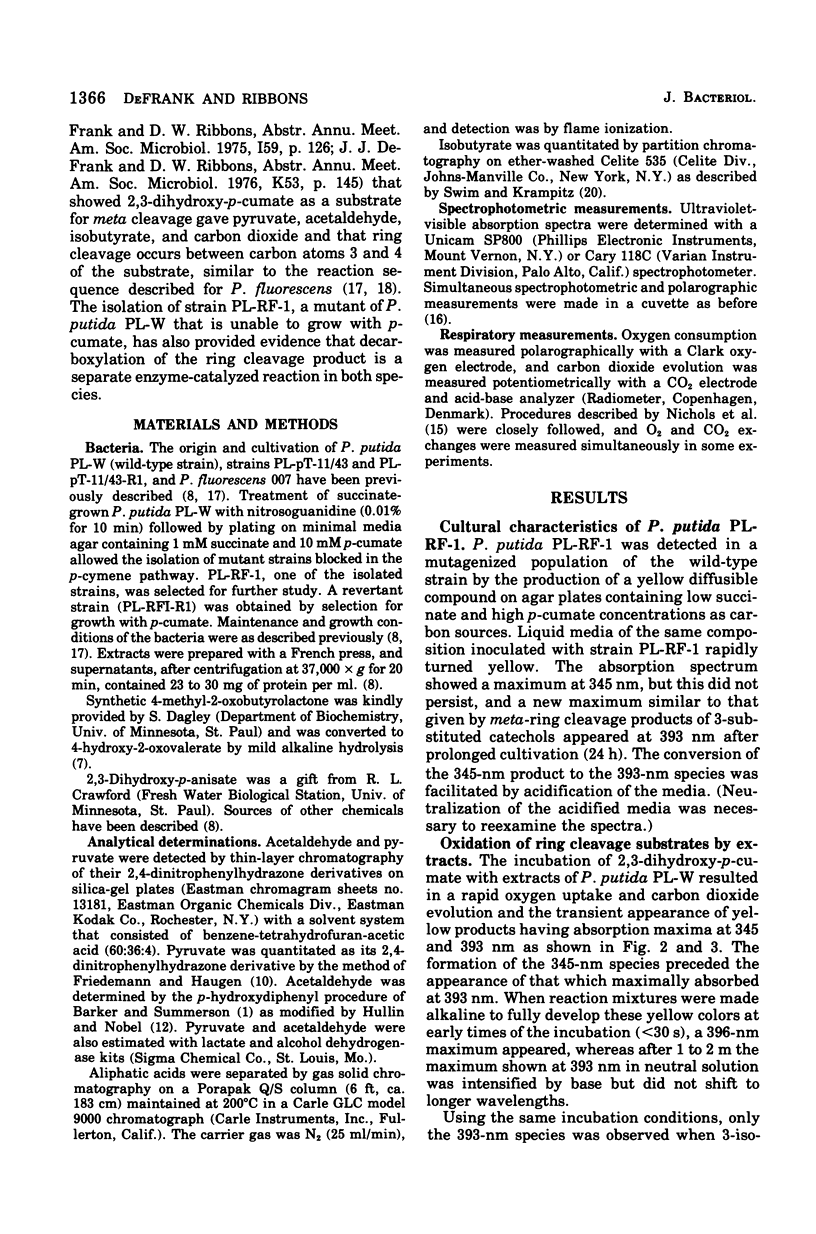
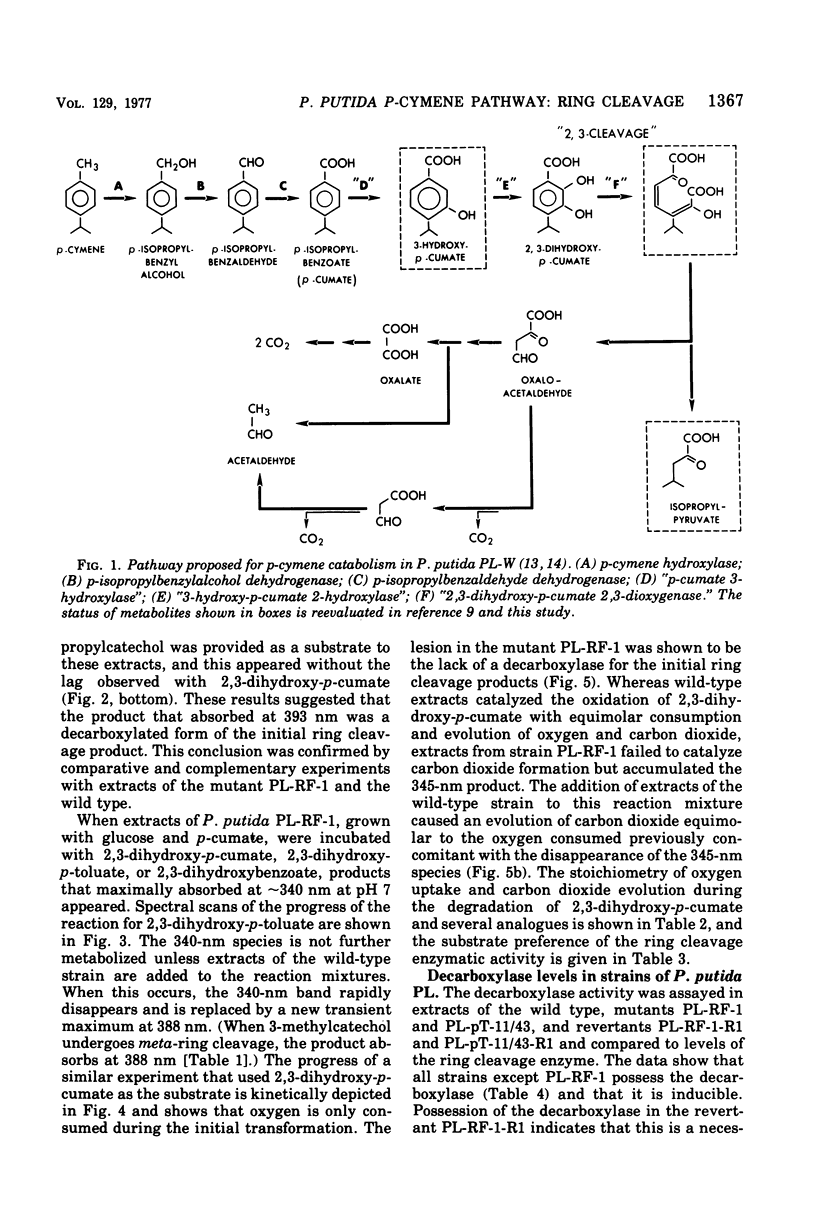
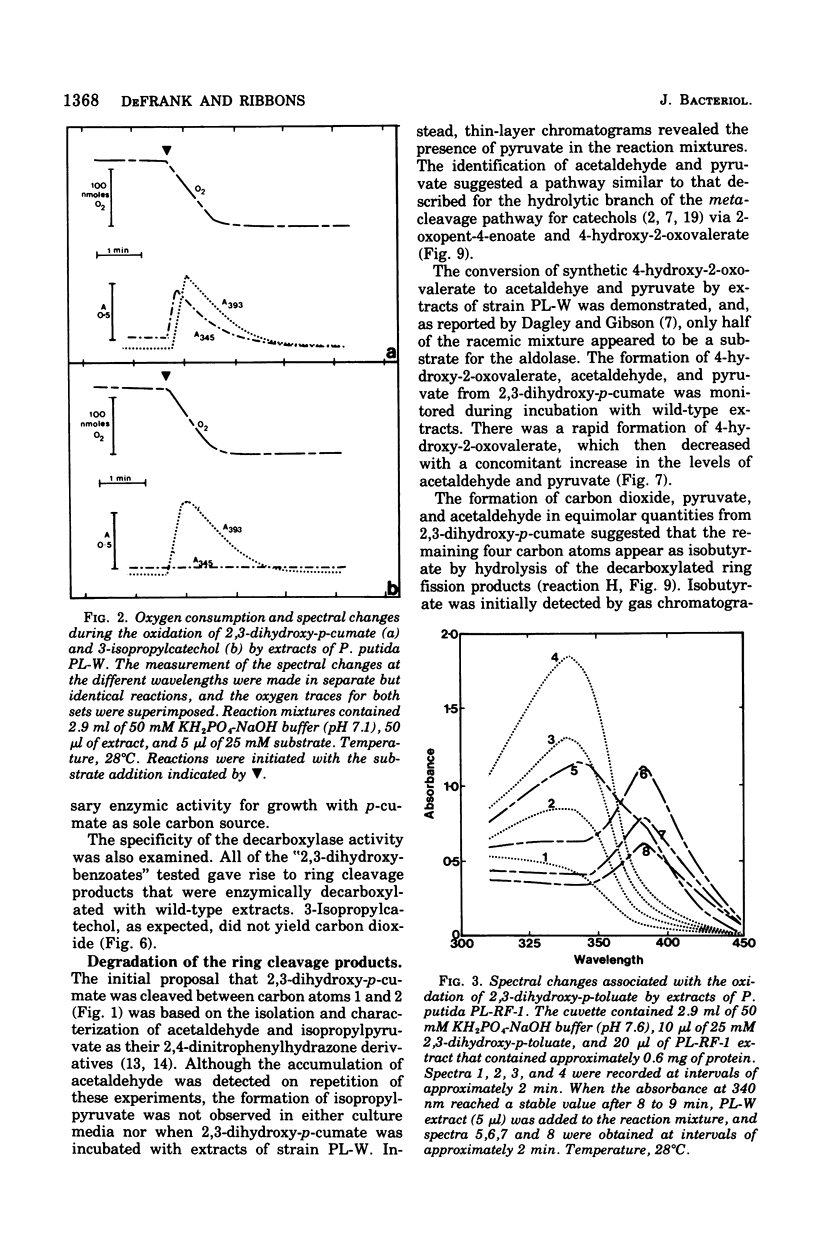
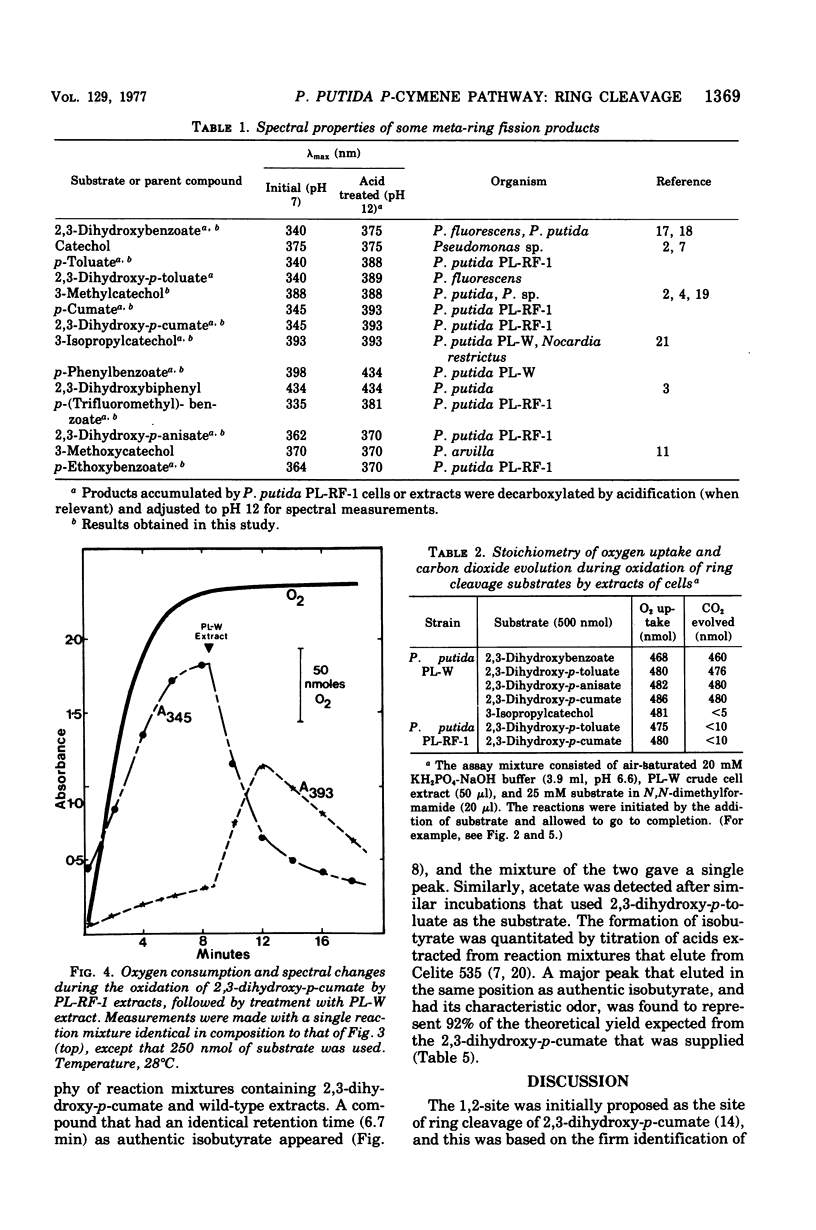
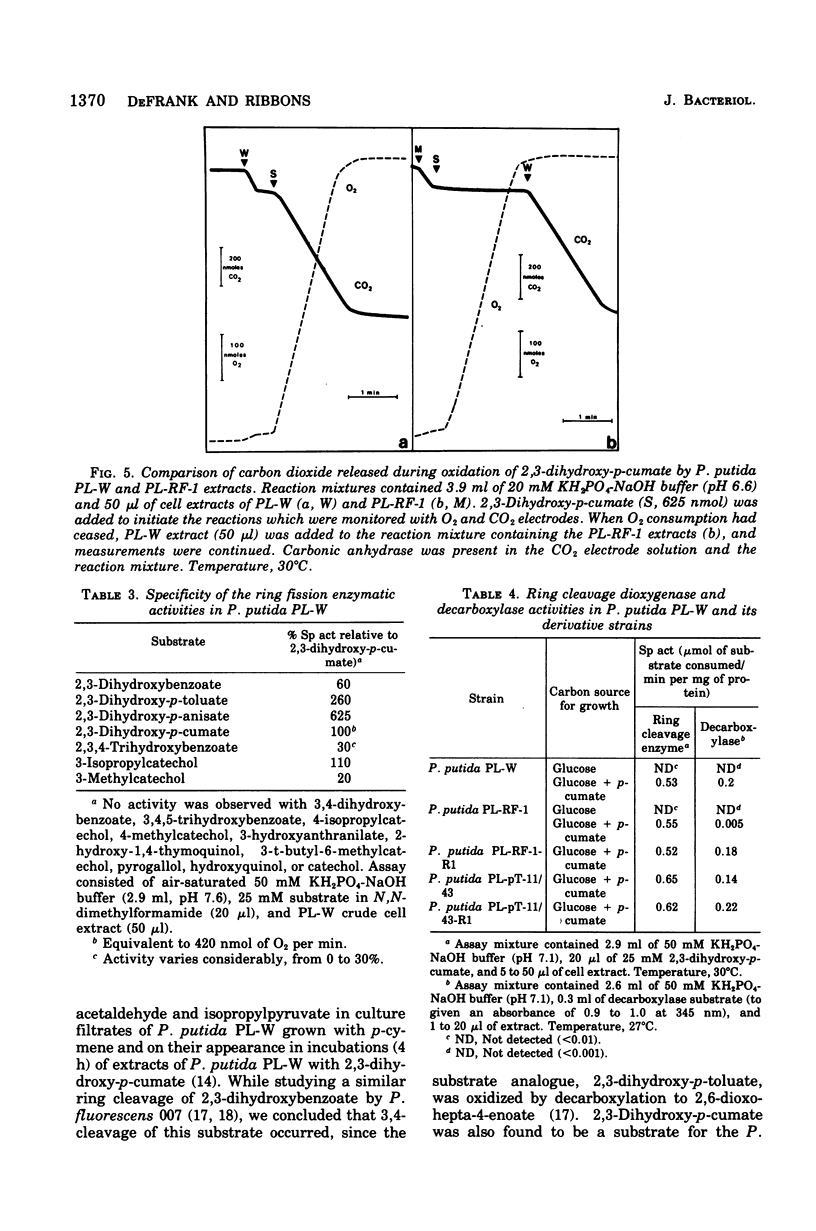
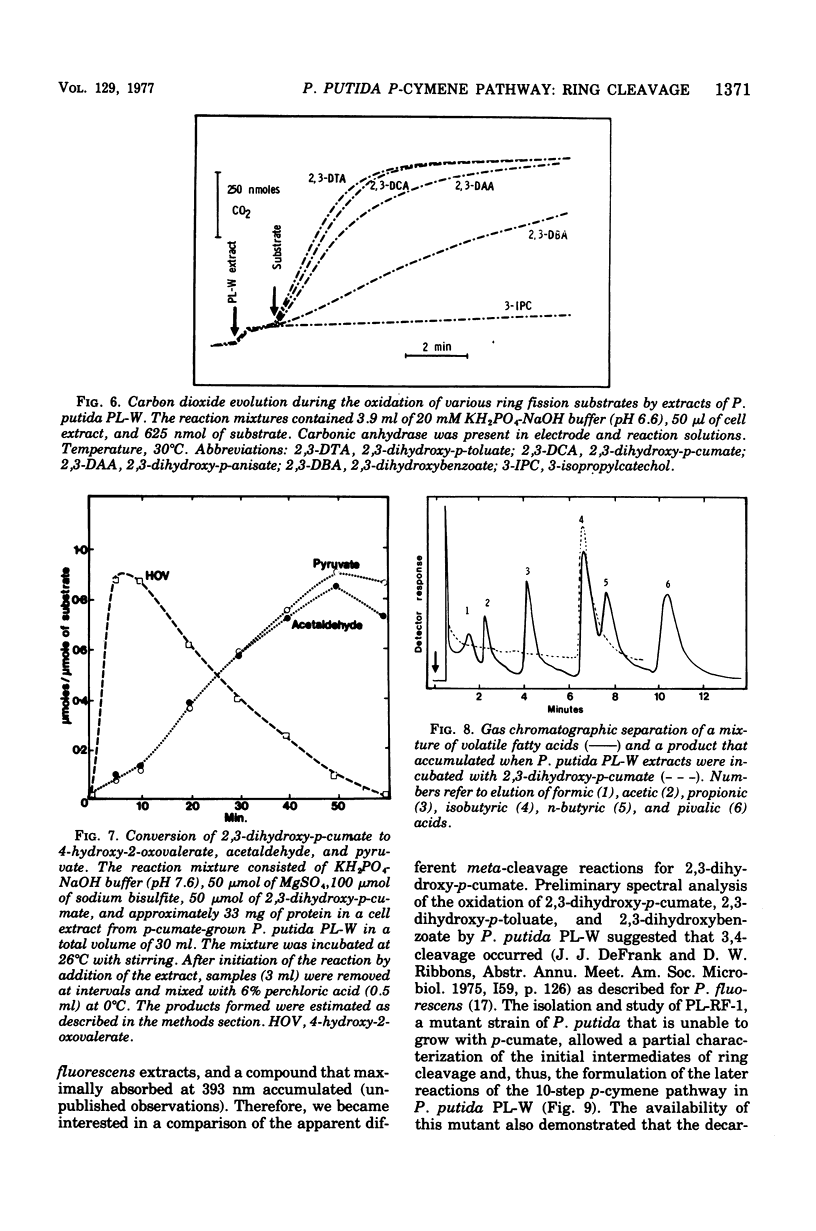
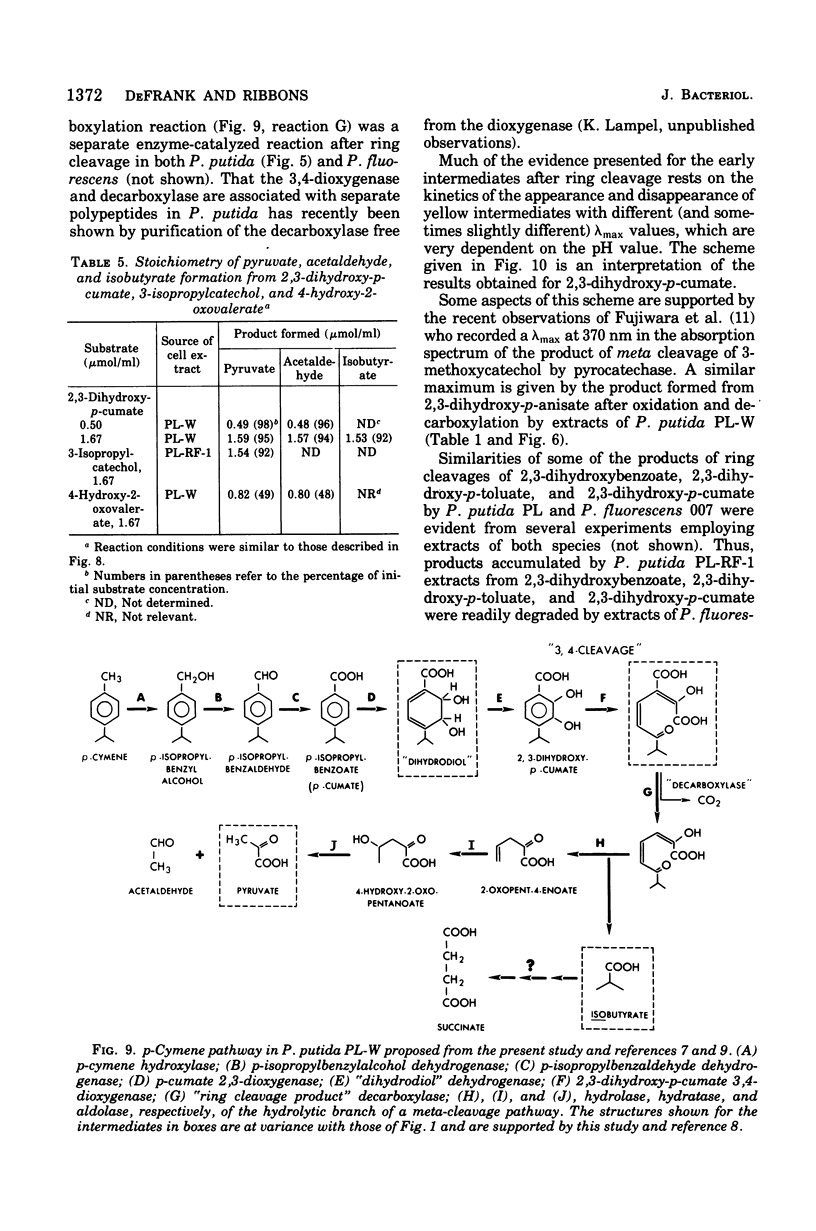
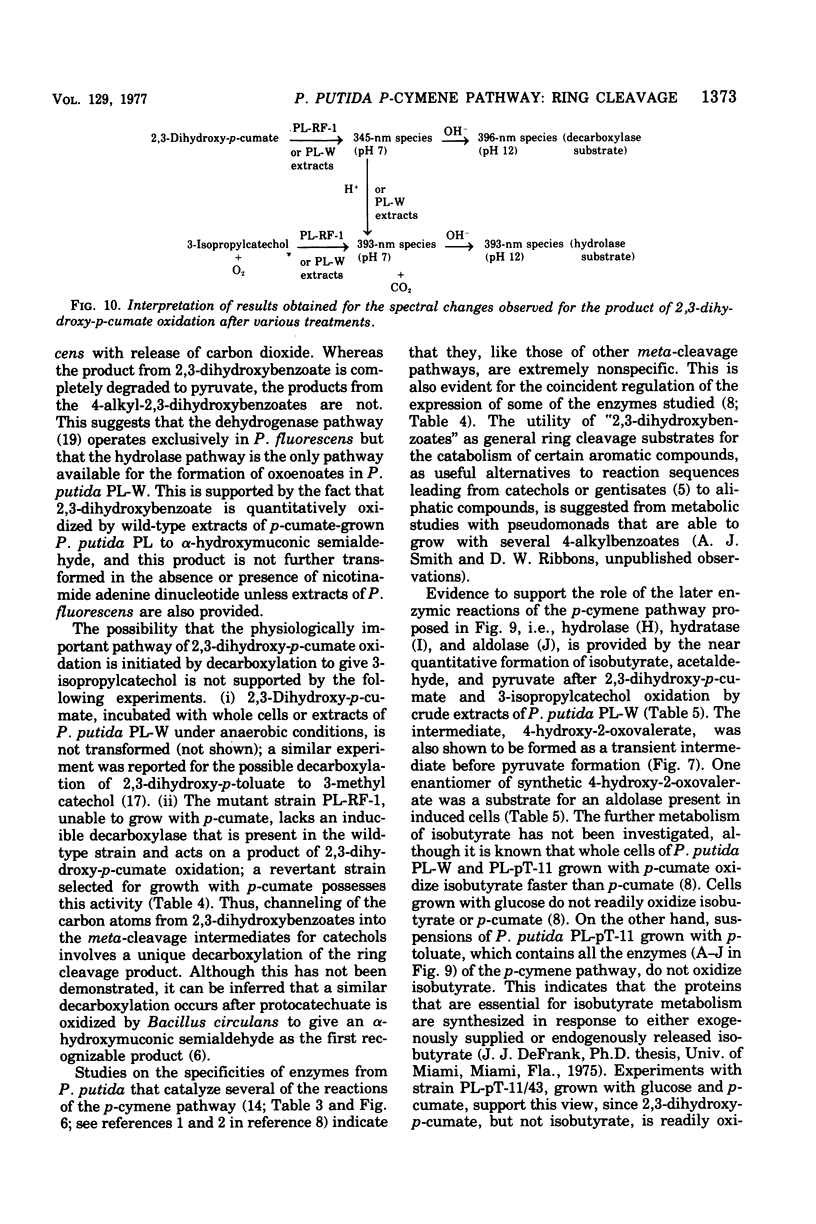
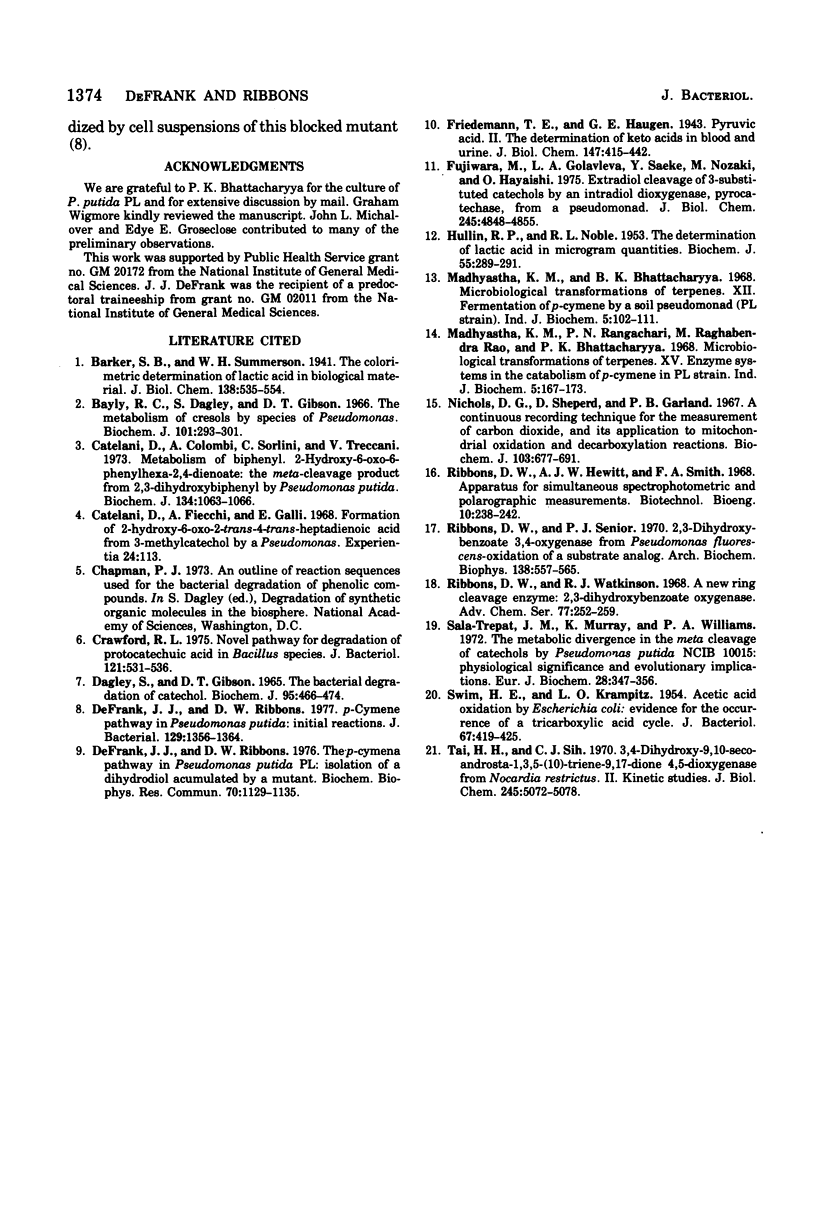
Selected References
These references are in PubMed. This may not be the complete list of references from this article.
- Bayly R. C., Dagley S., Gibson D. T. The metabolism of cresols by species of Pseudomonas. Biochem J. 1966 Nov;101(2):293–301. doi: 10.1042/bj1010293. [DOI] [PMC free article] [PubMed] [Google Scholar]
- Catelani D., Colombi A., Sorlini C., Treccani V. Metabolism of biphenyl. 2-Hydroxy-6-oxo-6-phenylhexa-2,4-dienoate: the meta-cleavage product from 2,3-dihydroxybiphenyl by Pseudomonas putida. Biochem J. 1973 Aug;134(4):1063–1066. doi: 10.1042/bj1341063. [DOI] [PMC free article] [PubMed] [Google Scholar]
- Catelani D., Fiecchi A., Galli E. Formation of 2-hydroxy-6-oxo-2, trans-4, trans-heptad-ienoic acid from 3-methylcatechol by a Pseudomonas. Experientia. 1968 Feb 15;24(2):113–113. doi: 10.1007/BF02146927. [DOI] [PubMed] [Google Scholar]
- Crawford R. L. Novel pathway for degradation of protocatechuic acid in Bacillus species. J Bacteriol. 1975 Feb;121(2):531–536. doi: 10.1128/jb.121.2.531-536.1975. [DOI] [PMC free article] [PubMed] [Google Scholar]
- DAGLEY S., GIBSON D. T. THE BACTERIAL DEGRADATION OF CATECHOL. Biochem J. 1965 May;95:466–474. doi: 10.1042/bj0950466. [DOI] [PMC free article] [PubMed] [Google Scholar]
- DeFrank J. J., Ribbons D. W. p-cymene pathway in Pseudomonas putida: initial reactions. J Bacteriol. 1977 Mar;129(3):1356–1364. doi: 10.1128/jb.129.3.1356-1364.1977. [DOI] [PMC free article] [PubMed] [Google Scholar]
- Defrank J. J., Ribbons D. W. The p-cymene pathway in Pseudomonas putida PL: isolation of a dihydrodiol accumulated by a mutant. Biochem Biophys Res Commun. 1976 Jun 21;70(4):1129–1135. doi: 10.1016/0006-291x(76)91020-2. [DOI] [PubMed] [Google Scholar]
- Fujiwara M., Golovleva L. A., Saeki Y., Nozaki M., Hayaishi O. Extradiol cleavage of 3-substituted catechols by an intradiol dioxygenase, pyrocatechase, from a Pseudomonad. J Biol Chem. 1975 Jul 10;250(13):4848–4855. [PubMed] [Google Scholar]
- HULLIN R. P., NOBLE R. L. The determination of lacic acid in microgram quantities. Biochem J. 1953 Sep;55(2):289–291. doi: 10.1042/bj0550289. [DOI] [PMC free article] [PubMed] [Google Scholar]
- Madhyastha K. M., Rangachari P. N., Raghabendra Rao M., Bhattacharyya P. K. Microbiological transformations of terpenes. XV. Enzyme systems in the catabolism of p-cymene in PL-strain. Indian J Biochem. 1968 Dec;5(4):167–173. [PubMed] [Google Scholar]
- Nicholls D. G., Shepherd D., Garland P. B. A continuous recording technique for the measurement of carbon dioxide, and its application to mitochondrial oxidation and decarboxylation reactions. Biochem J. 1967 Jun;103(3):677–691. doi: 10.1042/bj1030677. [DOI] [PMC free article] [PubMed] [Google Scholar]
- Ribbons D. W., Senior P. J. 2,3-dihydroxybenzoate 3,4-oxygenase from Pseudomonas fluorescens--oxidation of a substrate analog. Arch Biochem Biophys. 1970 Jun;138(2):557–565. doi: 10.1016/0003-9861(70)90381-4. [DOI] [PubMed] [Google Scholar]
- SWIM H. E., KRAMPITZ L. O. Acetic acid oxidation by Escherichia coli; evidence for the occurrence of a tricarboxylic acid cycle. J Bacteriol. 1954 Apr;67(4):419–425. doi: 10.1128/jb.67.4.419-425.1954. [DOI] [PMC free article] [PubMed] [Google Scholar]
- Sala-Trepat J. M., Murray K., Williams P. A. The metabolic divergence in the meta cleavage of catechols by Pseudomonas putida NCIB 10015. Physiological significance and evolutionary implications. Eur J Biochem. 1972 Jul 24;28(3):347–356. doi: 10.1111/j.1432-1033.1972.tb01920.x. [DOI] [PubMed] [Google Scholar]
- Tai H. H., Sih C. J. 3,4-dihydroxy-9,10-secoandrosta-1,3-5(10)-triene-9,17-dione 4,-5-dioxygenase from Nocardia restrictus. II. Kinetic studies. J Biol Chem. 1970 Oct 10;245(19):5072–5078. [PubMed] [Google Scholar]