Abstract
The effects of selected metabolic and respiratory inhibitors on the gliding motility of Flexibacter polymorphus were examined. Motility and oxygen consumption were quantitatively inhibited in a reversible manner by specific respiratory poisons, suggesting that gliding velocity was linked to electron transport activity. Arsenate had little influence on the number or rate of gliding filaments, despite a 95% decrease in the concentration of intracellular adenosine 5′-triphosphate (ATP). At concentrations of cyanide or azide that abolished gliding movement, cells possessed a level of ATP that should have been sufficient to allow motility. Proton-conducting uncouplers of oxidative phosphorylation, such as carbonylcyanide m-chlorophenylhydrazone (CCCP) and tetrachlorosalicylanilide, strongly inhibited locomotion yet did not suppress respiratory activity or intracellular ATP sufficiently to account for their effect on movement. Inhibition of motility by CCCP (but not by tetrachlorosalicylanilide) was partially reversed by sulfhydryl compounds. However, unlike CCCP, inhibition of motility by p-chloromercuribenzoate, a known sulfhydryl-blocking reagent, was associated with a corresponding reduction in respiratory activity and ATP content of cells. Protein synthesis was not blocked by concentrations of CCCP inhibitory for motility, indicating that utilization of existing ATP in this energy-requiring process was not impaired. These data suggest (but do not unequivocally prove) that ATP may not function as the sole energy donor for the gliding mechanism, but that some additional product of electron transport is required (e.g., the intermediate of oxidative phosphorylation).
Full text
PDF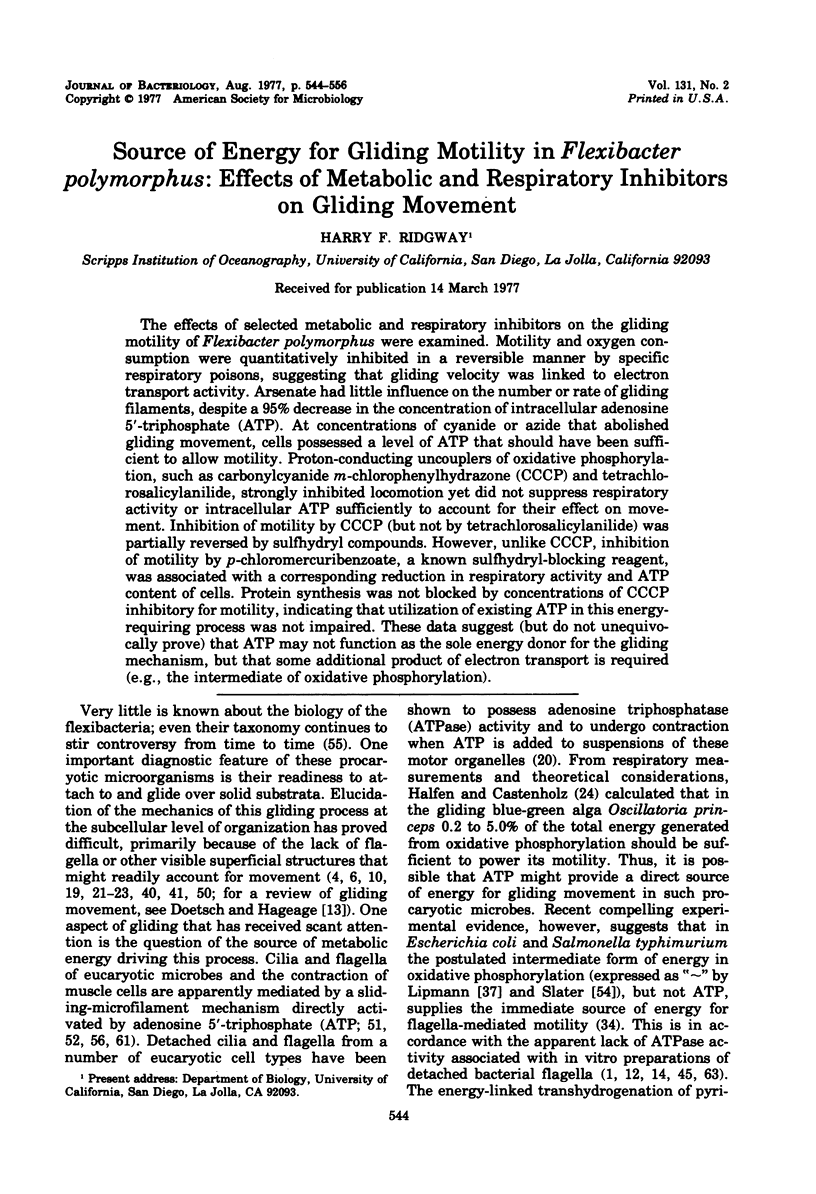
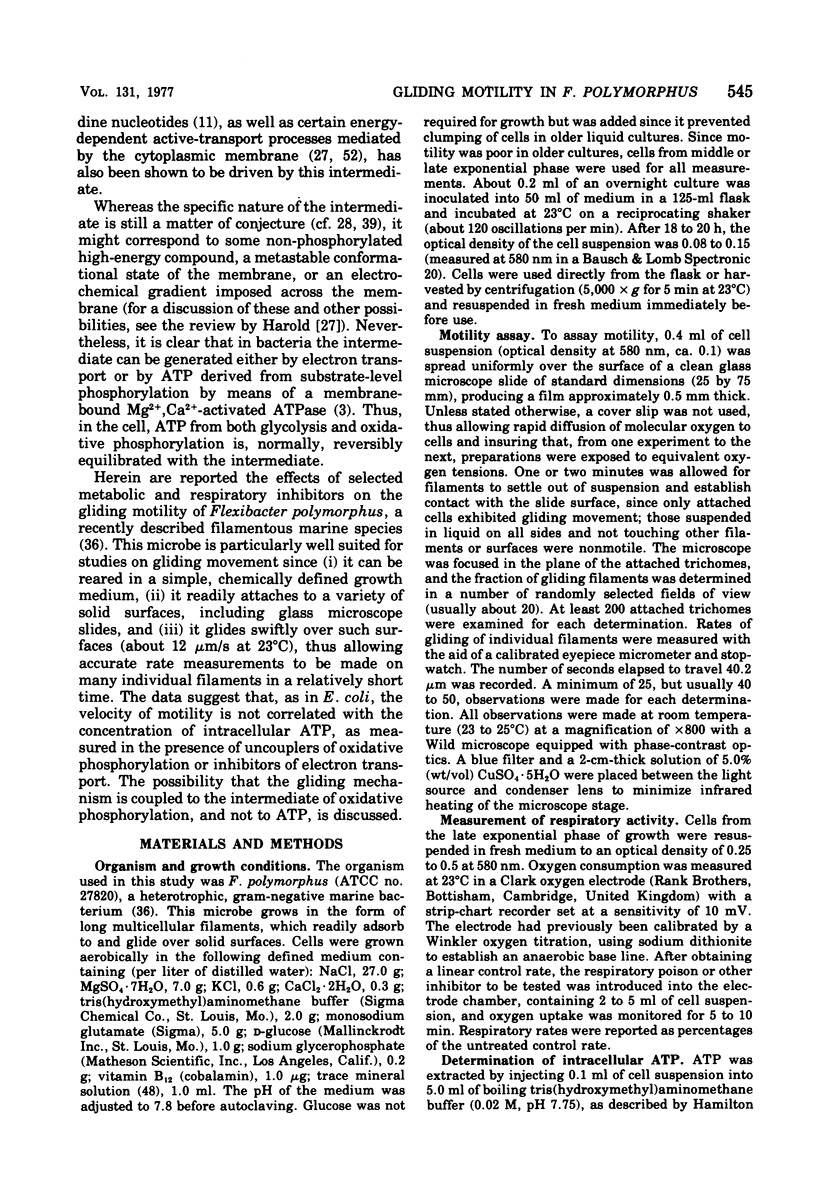
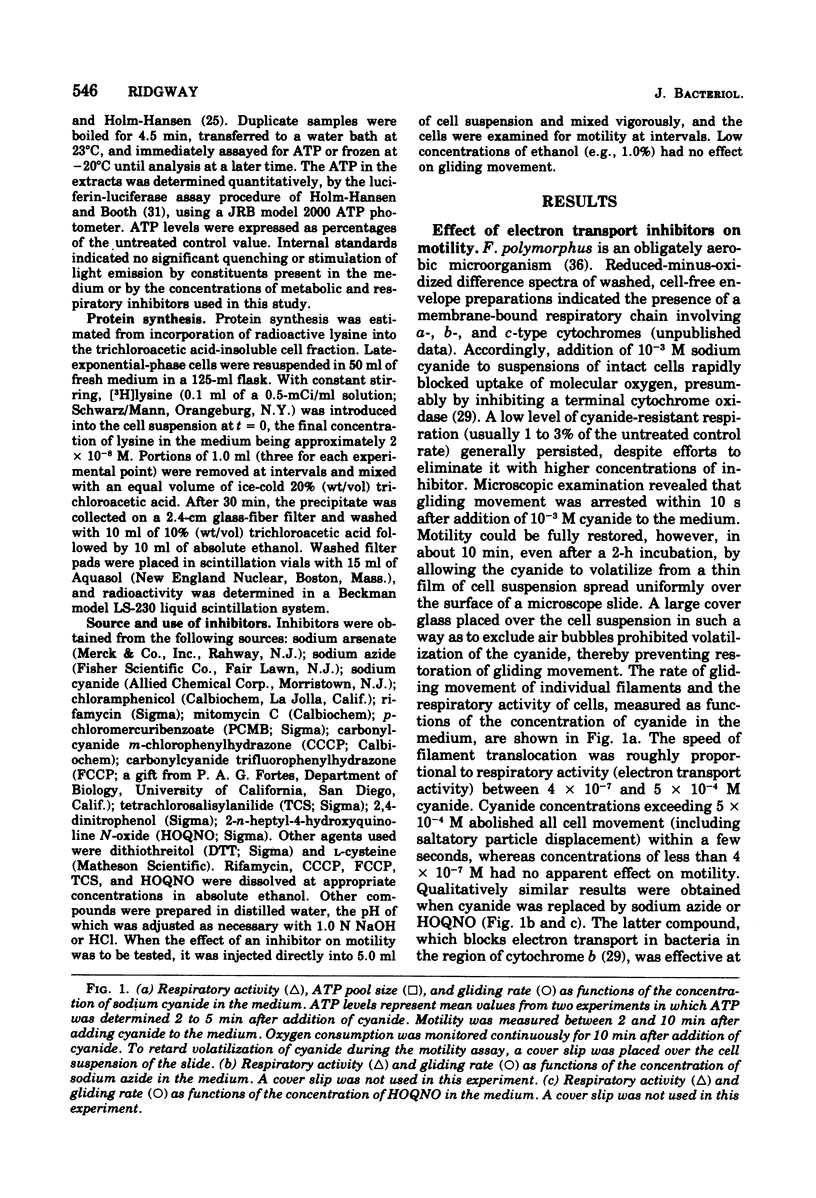
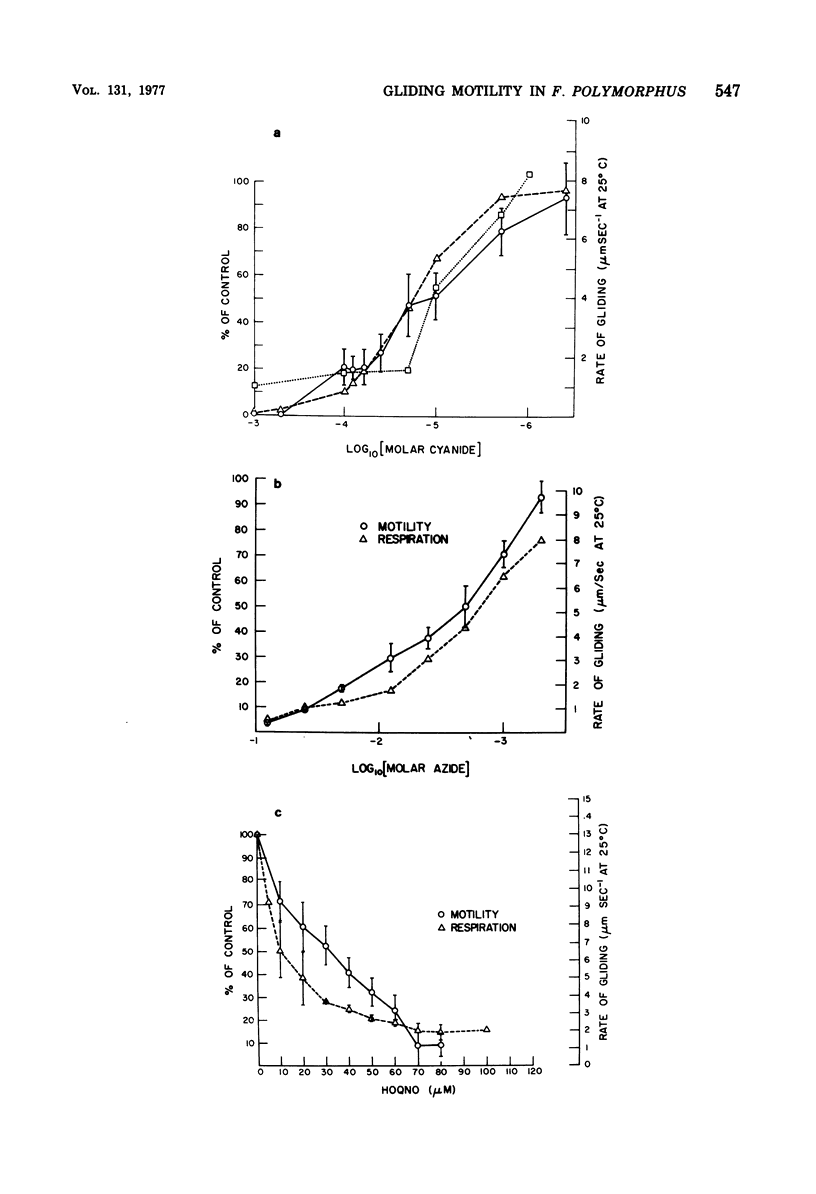
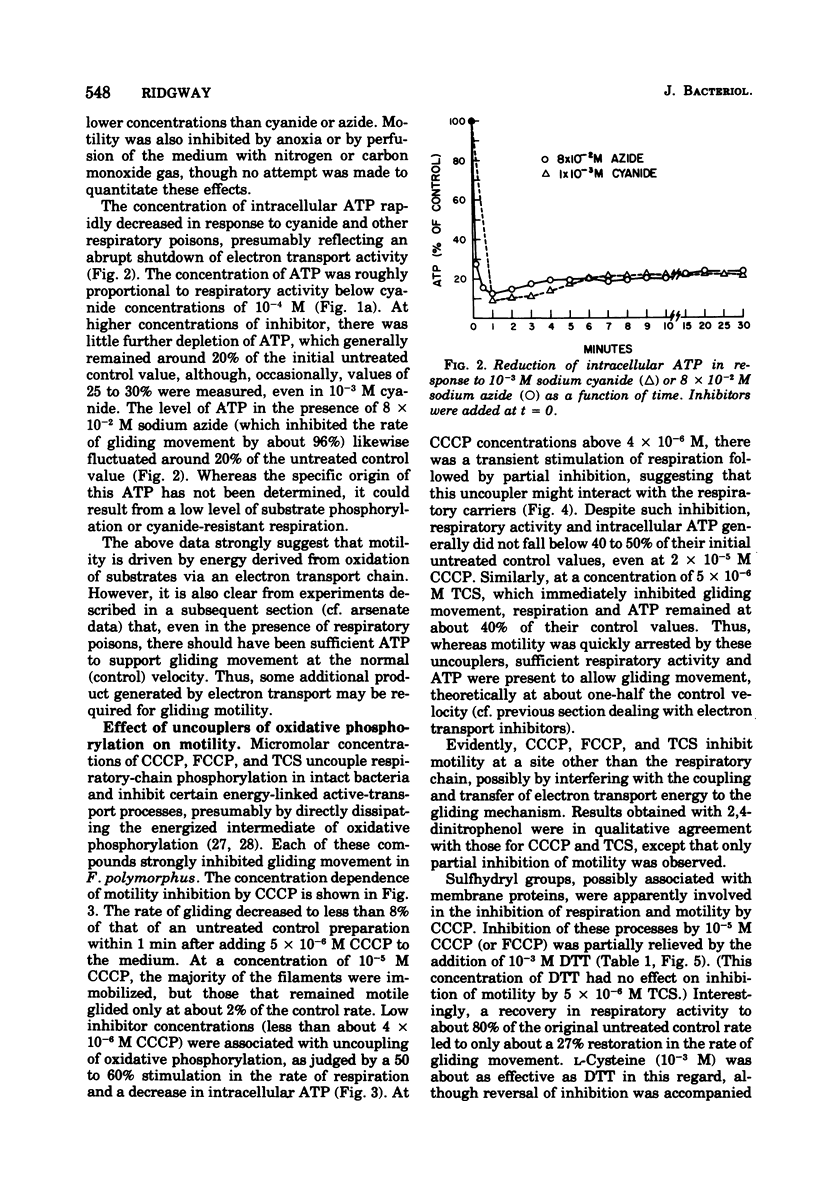
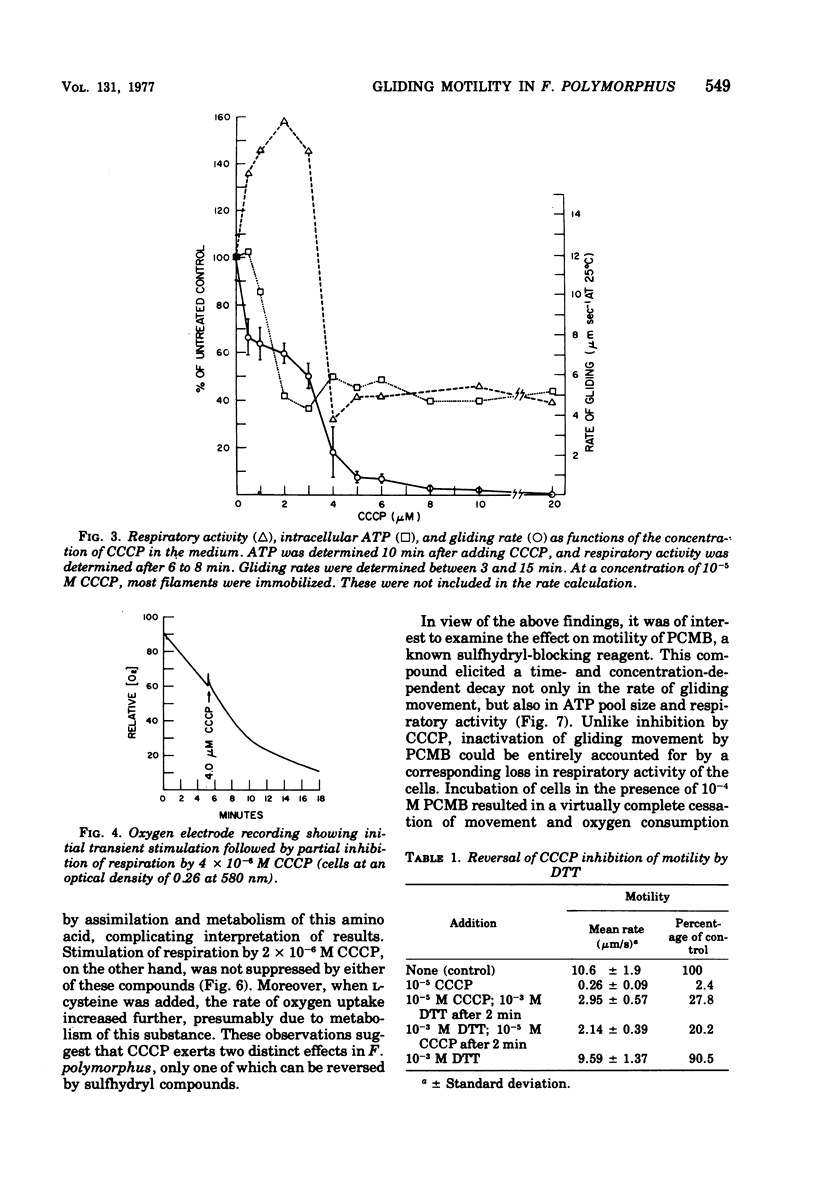
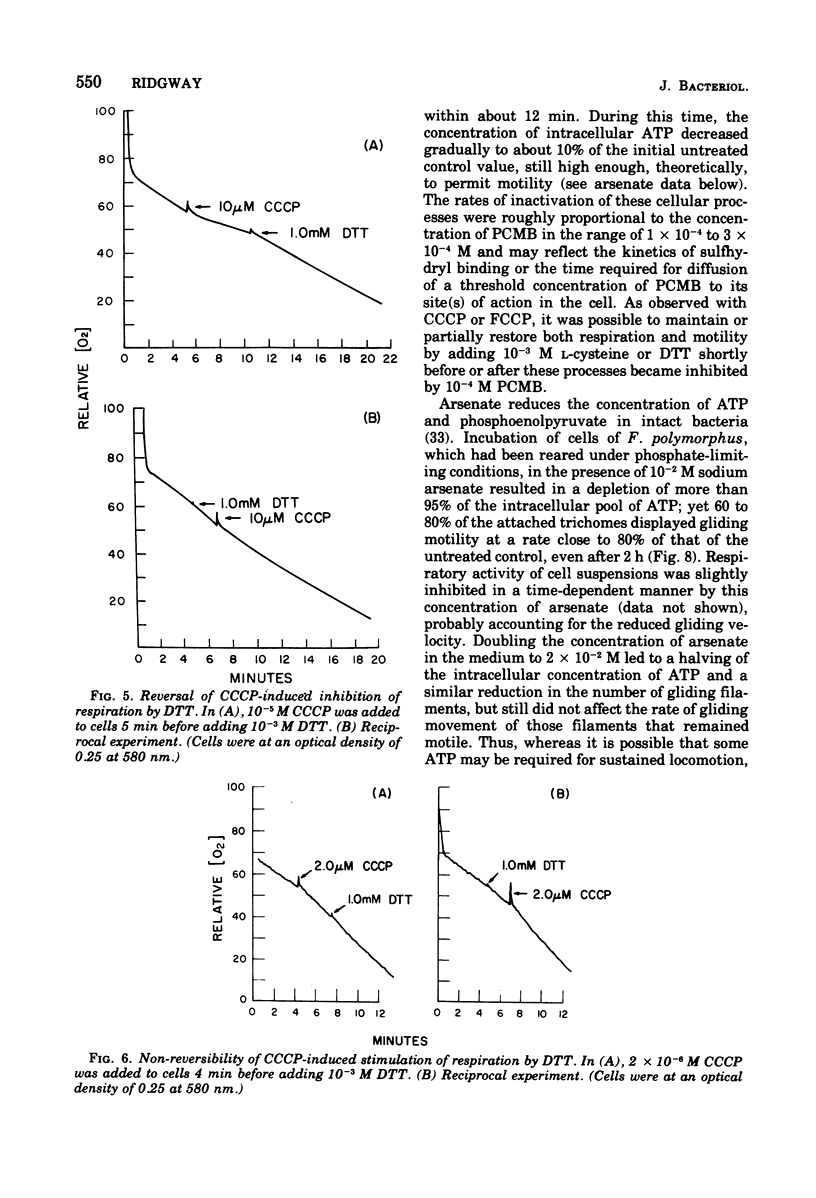
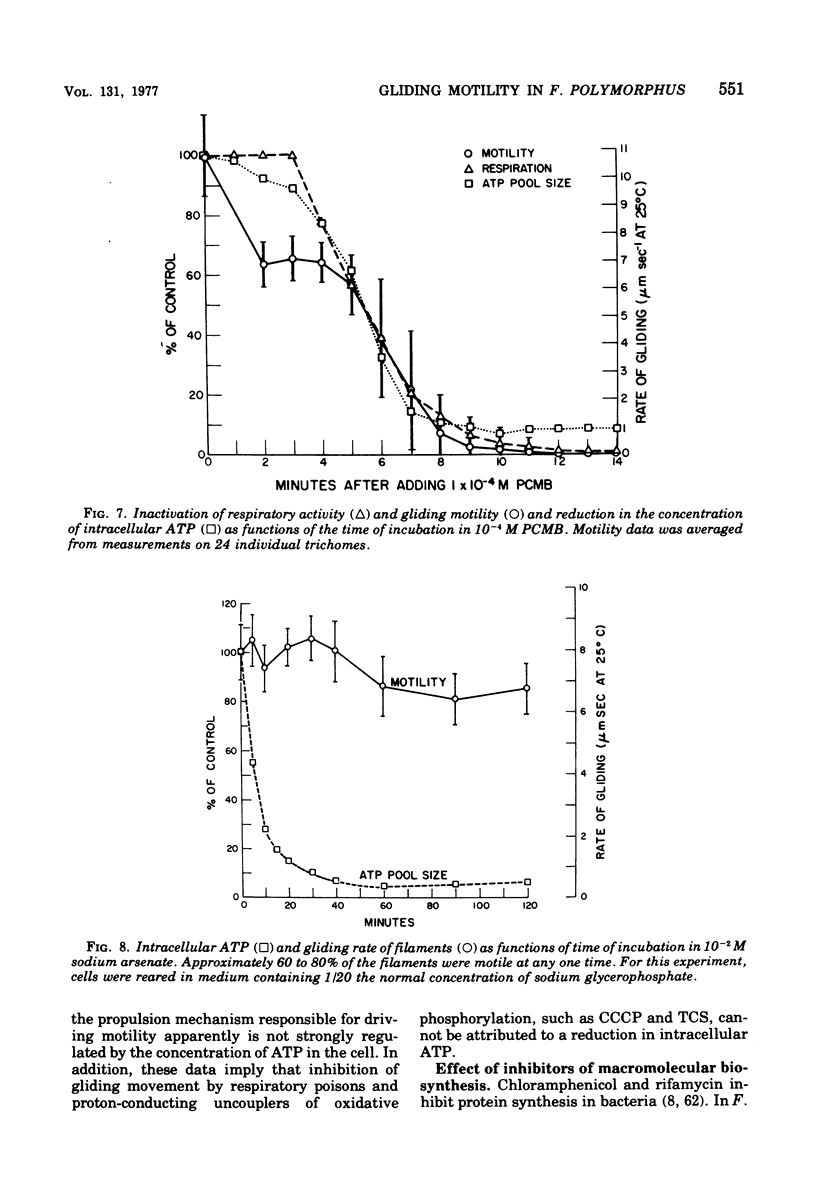
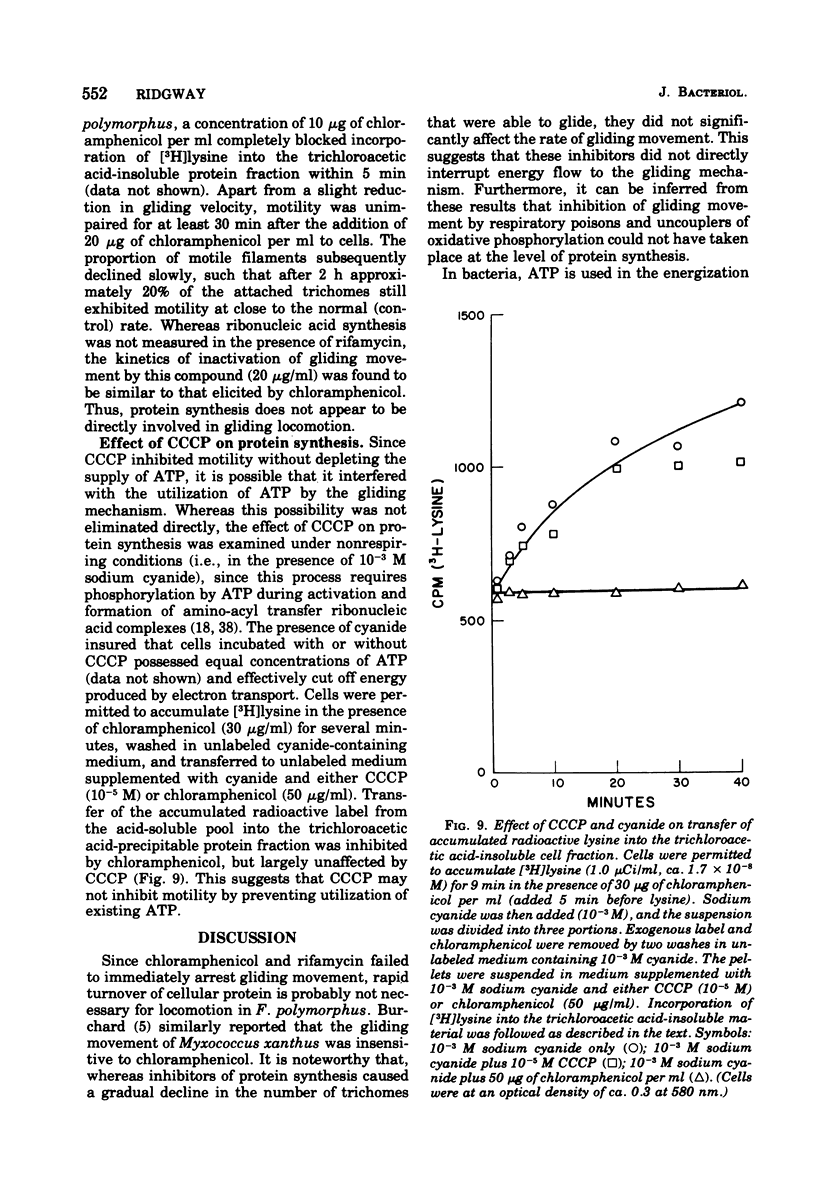
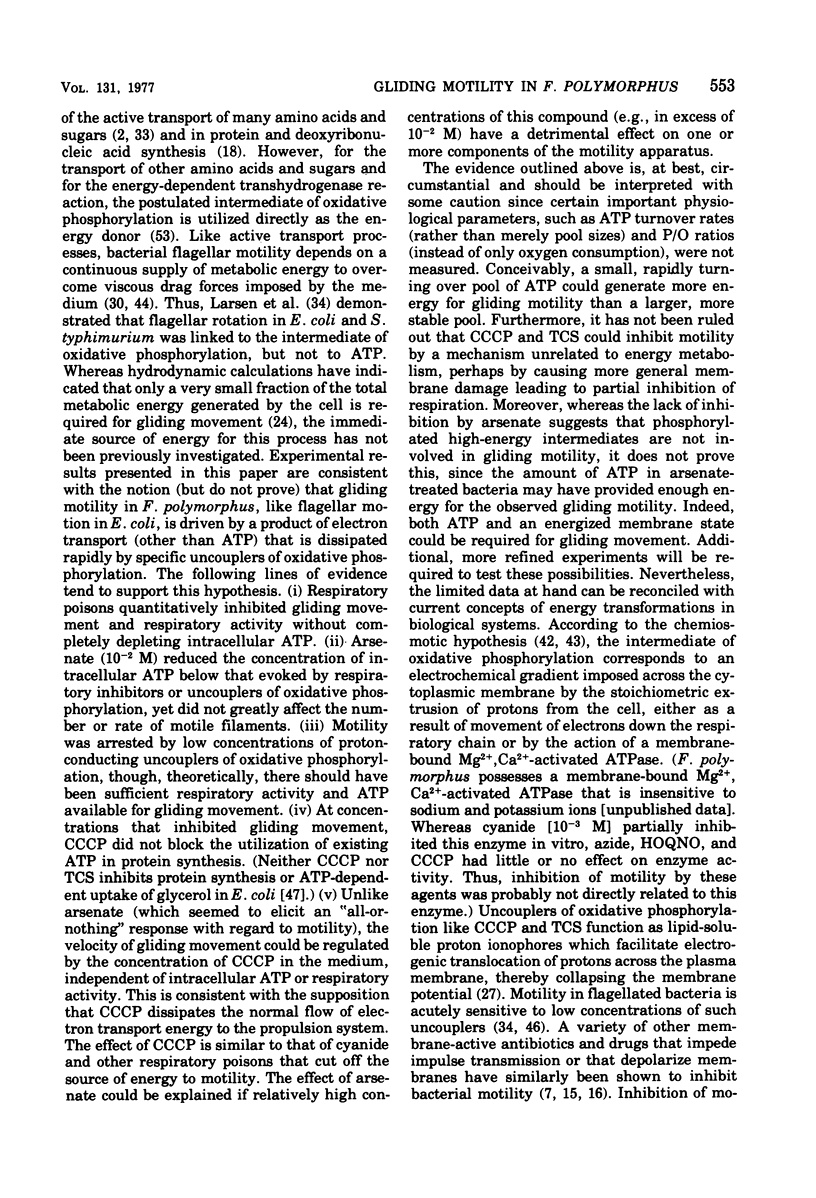
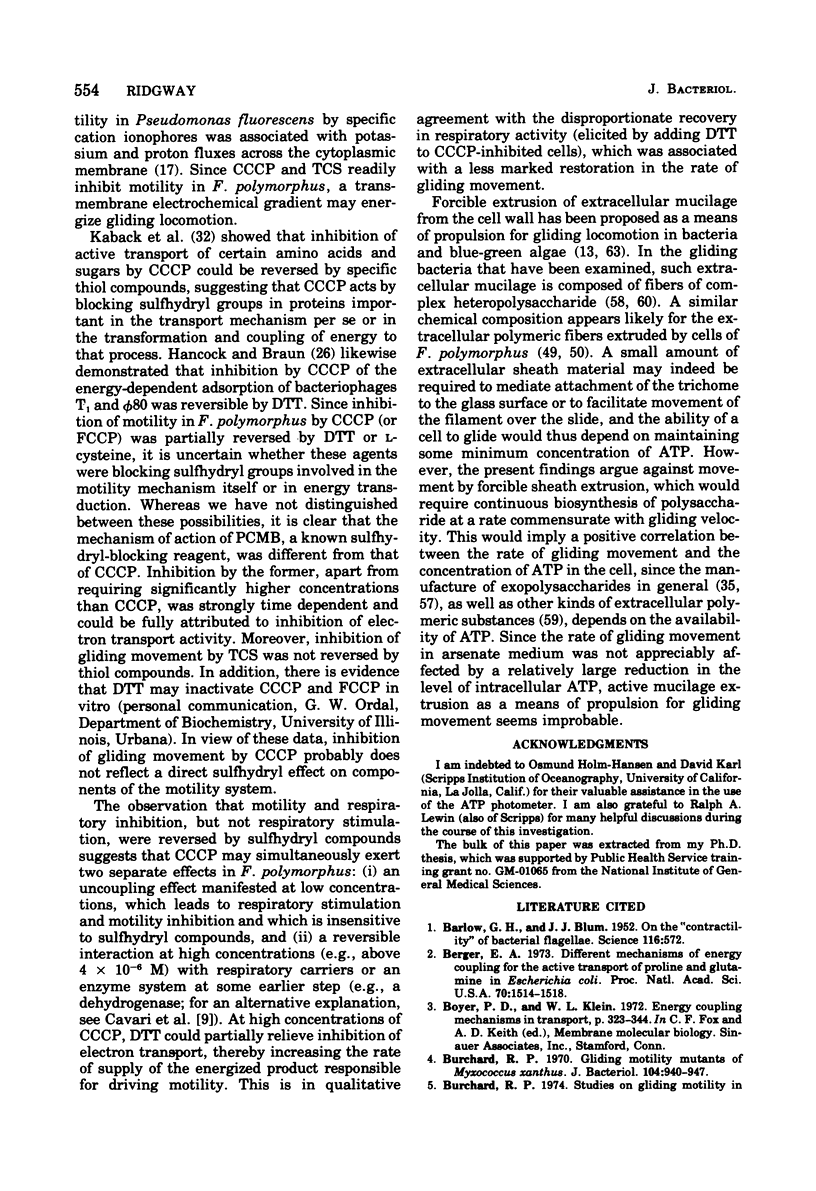
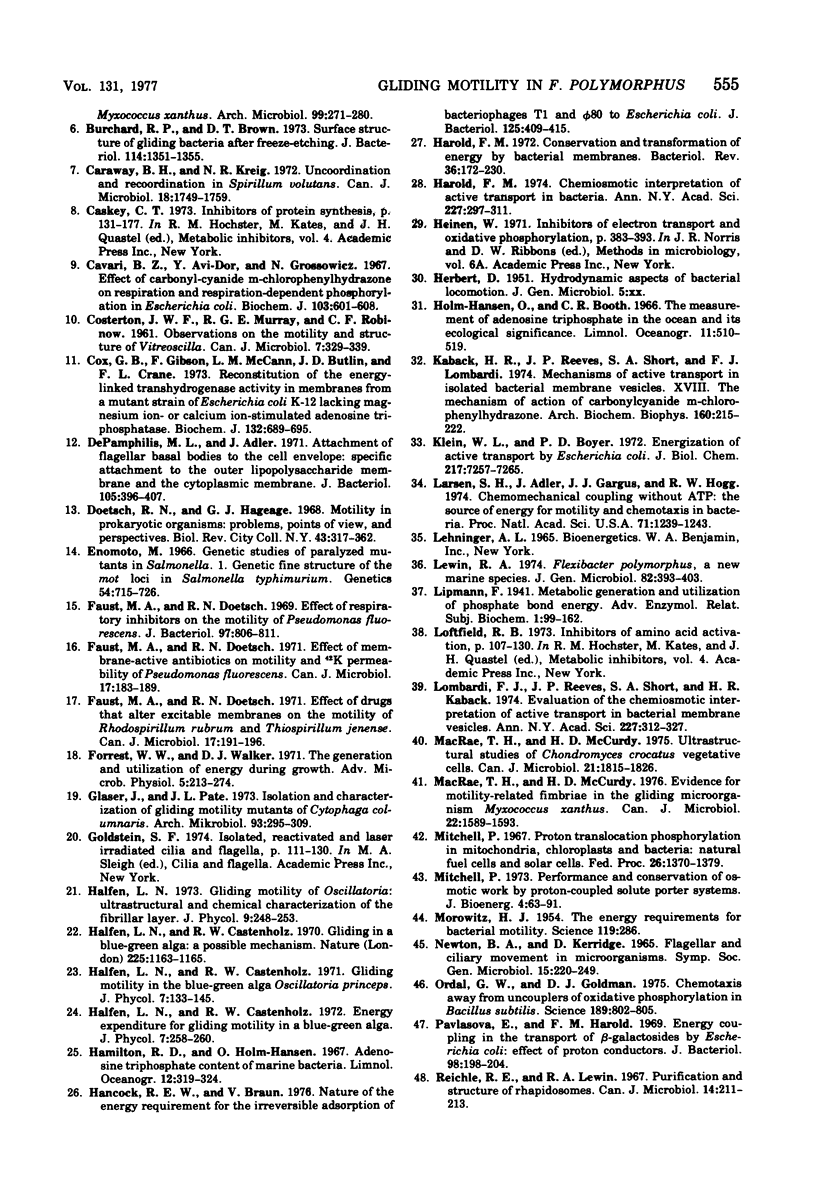
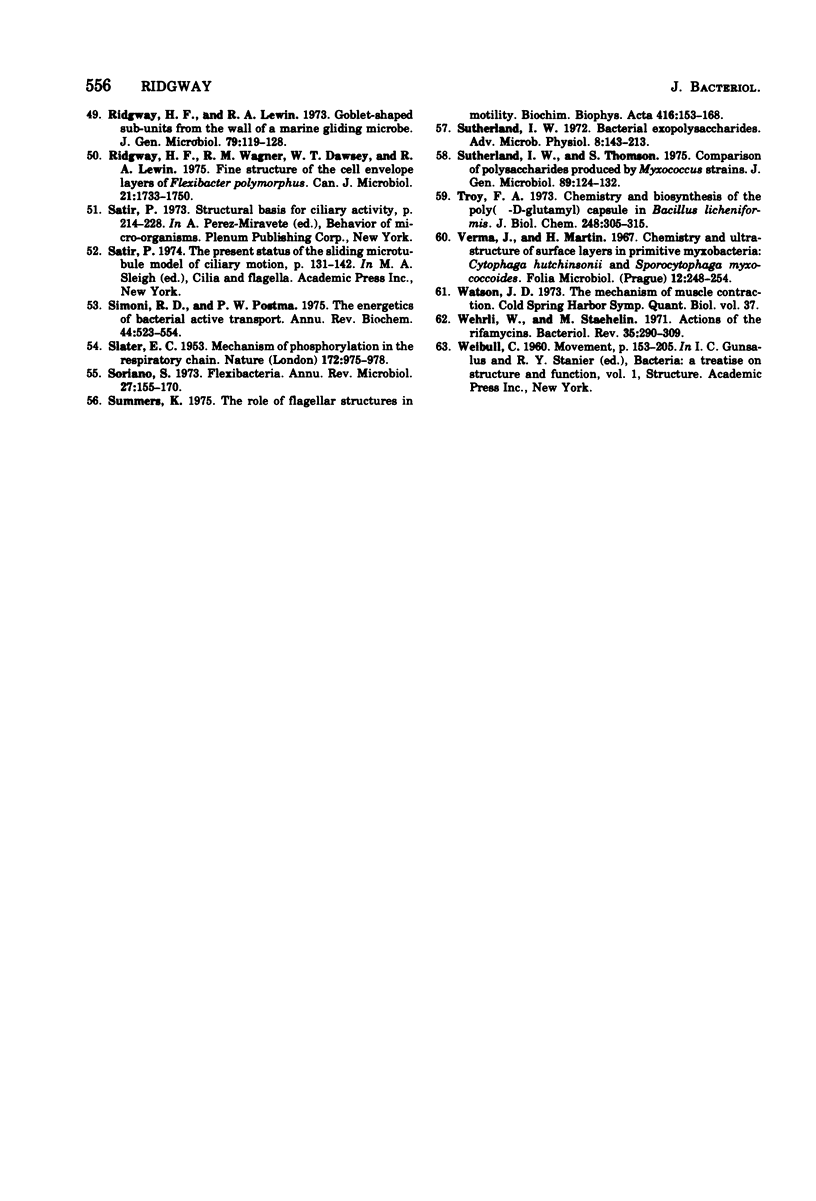
Selected References
These references are in PubMed. This may not be the complete list of references from this article.
- Barlow G. H., Blum J. J. On the "Contractility" of Bacterial Flagellae. Science. 1952 Nov 21;116(3021):572–572. doi: 10.1126/science.116.3021.572-a. [DOI] [PubMed] [Google Scholar]
- Berger E. A. Different mechanisms of energy coupling for the active transport of proline and glutamine in Escherichia coli. Proc Natl Acad Sci U S A. 1973 May;70(5):1514–1518. doi: 10.1073/pnas.70.5.1514. [DOI] [PMC free article] [PubMed] [Google Scholar]
- Burchard R. P., Brown D. T. Surface structure of gliding bacteria after freeze-etching. J Bacteriol. 1973 Jun;114(3):1351–1355. doi: 10.1128/jb.114.3.1351-1355.1973. [DOI] [PMC free article] [PubMed] [Google Scholar]
- Burchard R. P. Gliding motility mutants of Myxococcus xanthus. J Bacteriol. 1970 Nov;104(2):940–947. doi: 10.1128/jb.104.2.940-947.1970. [DOI] [PMC free article] [PubMed] [Google Scholar]
- Burchard R. P. Studies on gliding motility in Myxococcus xanthus. Arch Microbiol. 1974;99(3):271–280. doi: 10.1007/BF00696242. [DOI] [PubMed] [Google Scholar]
- COSTERTON J. W., MURRAY R. G., ROBINOW C. F. Observations on the motility and the structure of Vitreoscilla. Can J Microbiol. 1961 Jun;7:329–339. doi: 10.1139/m61-040. [DOI] [PubMed] [Google Scholar]
- Caraway B. H., Krieg N. R. Uncoordination and recoordination in Spirillum volutans. Can J Microbiol. 1972 Nov;18(11):1749–1759. doi: 10.1139/m72-271. [DOI] [PubMed] [Google Scholar]
- Cavari B. Z., Avi-Dor Y. Effect of carbonyl cyanide m-chlorophenylhydrazone on respiration and respiration-dependent phosphorylation in Escherichia coli. Biochem J. 1967 May;103(2):601–608. doi: 10.1042/bj1030601. [DOI] [PMC free article] [PubMed] [Google Scholar]
- Cox G. B., Gibson F., McCann L. M., Butlin J. D., Crane F. L. Reconstitution of the energy-linked transhydrogenase activity in membranes from a mutant strain of Escherichia coli K12 lacking magnesium ion- or calcium ion-stimulated adenosine triphosphatase. Biochem J. 1973 Apr;132(4):689–695. doi: 10.1042/bj1320689. [DOI] [PMC free article] [PubMed] [Google Scholar]
- DePamphilis M. L., Adler J. Attachment of flagellar basal bodies to the cell envelope: specific attachment to the outer, lipopolysaccharide membrane and the cyoplasmic membrane. J Bacteriol. 1971 Jan;105(1):396–407. doi: 10.1128/jb.105.1.396-407.1971. [DOI] [PMC free article] [PubMed] [Google Scholar]
- Doetsch R. N., Hageage G. J. Motility in procaryotic organisms: problems, points of view, and perspectives. Biol Rev Camb Philos Soc. 1968 Aug;43(3):317–362. doi: 10.1111/j.1469-185x.1968.tb00963.x. [DOI] [PubMed] [Google Scholar]
- Enomoto M. Genetic studies of paralyzed mutant in Salmonella. I. Genetic fine structure of the mot loci in Salmonella typhimurium. Genetics. 1966 Sep;54(3):715–726. doi: 10.1093/genetics/54.3.715. [DOI] [PMC free article] [PubMed] [Google Scholar]
- Faust M. A., Doetsch R. N. Effect of drugs that alter excitable membranes on the motility of Rhodospirillum rubrum and Thiospirillum jenense. Can J Microbiol. 1971 Feb;17(2):191–196. doi: 10.1139/m71-033. [DOI] [PubMed] [Google Scholar]
- Faust M. A., Doetsch R. N. Effect of membrane-active antibiotics on motility and 42K permeability of Pseudomonas fluorescens. Can J Microbiol. 1971 Feb;17(2):183–189. doi: 10.1139/m71-032. [DOI] [PubMed] [Google Scholar]
- Faust M. A., Doetsch R. N. Effect of respiratory inhibitors on the motility of Pseudomonas fluorescens. J Bacteriol. 1969 Feb;97(2):806–811. doi: 10.1128/jb.97.2.806-811.1969. [DOI] [PMC free article] [PubMed] [Google Scholar]
- Forrest W. W., Walker D. J. The generation and utilization of energy during growth. Adv Microb Physiol. 1971;5:213–274. doi: 10.1016/s0065-2911(08)60408-7. [DOI] [PubMed] [Google Scholar]
- Glaser J., Pate J. L. Isolation and characterization of gliding motility mutants of Cytophaga columnaris. Arch Mikrobiol. 1973 Nov 19;93(4):295–309. doi: 10.1007/BF00427927. [DOI] [PubMed] [Google Scholar]
- Halfen L. N., Castenholz R. W. Gliding in a blue-green alga: a possible mechanism. Nature. 1970 Mar 21;225(5238):1163–1165. doi: 10.1038/2251163a0. [DOI] [PubMed] [Google Scholar]
- Hancock R. W., Braun V. Nature of the energy requirement for the irreversible adsorption of bacteriophages T1 and phi80 to Escherichia coli. J Bacteriol. 1976 Feb;125(2):409–415. doi: 10.1128/jb.125.2.409-415.1976. [DOI] [PMC free article] [PubMed] [Google Scholar]
- Harold F. M. Chemiosmotic interpretation of active transport in bacteria. Ann N Y Acad Sci. 1974 Feb 18;227:297–311. doi: 10.1111/j.1749-6632.1974.tb14395.x. [DOI] [PubMed] [Google Scholar]
- Harold F. M. Conservation and transformation of energy by bacterial membranes. Bacteriol Rev. 1972 Jun;36(2):172–230. doi: 10.1128/br.36.2.172-230.1972. [DOI] [PMC free article] [PubMed] [Google Scholar]
- Kaback H. R., Reeves J. P., Short S. A., Lombardi F. J. Mechanisms of active transport in isolated bacterial membrane vesicles. 18. The mechanism of action of carbonylcyanide m-chlorophenylhydrazone. Arch Biochem Biophys. 1974 Jan;160(1):215–222. doi: 10.1016/s0003-9861(74)80028-7. [DOI] [PubMed] [Google Scholar]
- Klein W. L., Boyer P. D. Energization of active transport by Escherichia coli. J Biol Chem. 1972 Nov 25;247(22):7257–7265. [PubMed] [Google Scholar]
- Larsen S. H., Adler J., Gargus J. J., Hogg R. W. Chemomechanical coupling without ATP: the source of energy for motility and chemotaxis in bacteria. Proc Natl Acad Sci U S A. 1974 Apr;71(4):1239–1243. doi: 10.1073/pnas.71.4.1239. [DOI] [PMC free article] [PubMed] [Google Scholar]
- Lombardi F. J., Reeves J. P., Short S. A., Kaback H. R. Evaluation of the chemiosmotic interpretation of active transport in bacterial membrane vesicles. Ann N Y Acad Sci. 1974 Feb 18;227:312–327. doi: 10.1111/j.1749-6632.1974.tb14396.x. [DOI] [PubMed] [Google Scholar]
- MOROWITZ H. J. The energy requirements for bacterial motility. Science. 1954 Feb 26;119(3087):286–286. doi: 10.1126/science.119.3087.286. [DOI] [PubMed] [Google Scholar]
- MacRae T. H., McCurdy D. Evidence for motility-related fimbriae in the gliding microorganism Myxococcus xanthus. Can J Microbiol. 1976 Oct;22(10):1589–1593. doi: 10.1139/m76-234. [DOI] [PubMed] [Google Scholar]
- MacRae T. H., McCurdy H. D. Ultrastructural studies of Chondromyces crocatus vegetative cells. Can J Microbiol. 1975 Nov;21(11):1815–1826. doi: 10.1139/m75-264. [DOI] [PubMed] [Google Scholar]
- Mitchell P. Performance and conservation of osmotic work by proton-coupled solute porter systems. J Bioenerg. 1973 Jan;4(1):63–91. doi: 10.1007/BF01516051. [DOI] [PubMed] [Google Scholar]
- Mitchell P. Proton-translocation phosphorylation in mitochondria, chloroplasts and bacteria: natural fuel cells and solar cells. Fed Proc. 1967 Sep;26(5):1370–1379. [PubMed] [Google Scholar]
- Ordal G. W., Goldman D. J. Chemotaxis away from uncouplers of oxidative phosphorylation in Bacillus subtilis. Science. 1975 Sep 5;189(4205):802–805. doi: 10.1126/science.808854. [DOI] [PubMed] [Google Scholar]
- Pavlasova E., Harold F. M. Energy coupling in the transport of beta-galactosides by Escherichia coli: effect of proton conductors. J Bacteriol. 1969 Apr;98(1):198–204. doi: 10.1128/jb.98.1.198-204.1969. [DOI] [PMC free article] [PubMed] [Google Scholar]
- Reichle R. E., Lewin R. A. Purification and structure of rhapidosomes. Can J Microbiol. 1968 Mar;14(3):211–213. doi: 10.1139/m68-036. [DOI] [PubMed] [Google Scholar]
- Ridgway H. F., Wagner R. M., Dawsey W. T., Lewin R. A. Fine structure of the cell envelope layers of Flexibacter polymorphus. Can J Microbiol. 1975 Nov;21(11):1733–1750. doi: 10.1139/m75-254. [DOI] [PubMed] [Google Scholar]
- SLATER E. C. Mechanism of phosphorylation in the respiratory chain. Nature. 1953 Nov 28;172(4387):975–978. doi: 10.1038/172975a0. [DOI] [PubMed] [Google Scholar]
- Simoni R. D., Postma P. W. The energetics of bacterial active transport. Annu Rev Biochem. 1975;44:523–554. doi: 10.1146/annurev.bi.44.070175.002515. [DOI] [PubMed] [Google Scholar]
- Soriano S. Flexibacteria. Annu Rev Microbiol. 1973;27:155–170. doi: 10.1146/annurev.mi.27.100173.001103. [DOI] [PubMed] [Google Scholar]
- Summers K. The role of flagellar structures in motility. Biochim Biophys Acta. 1975 Aug 15;416(2):153–168. doi: 10.1016/0304-4173(75)90005-1. [DOI] [PubMed] [Google Scholar]
- Sutherland I. W. Bacterial exopolysaccharides. Adv Microb Physiol. 1972;8:143–213. doi: 10.1016/s0065-2911(08)60190-3. [DOI] [PubMed] [Google Scholar]
- Sutherland I. W., Thomson S. Comparison of polysaccharides produced by Myxococcus strains. J Gen Microbiol. 1975 Jul;89(1):124–132. doi: 10.1099/00221287-89-1-124. [DOI] [PubMed] [Google Scholar]
- Troy F. A. Chemistry and biosynthesis of the poly( -D-glutamyl) capsule in Bacillus licheniformis. I. Properties of the membrane-mediated biosynthetic reaction. J Biol Chem. 1973 Jan 10;248(1):305–315. [PubMed] [Google Scholar]
- Verma J. P., Martin H. H. Chemistry and ultrastructure of surface layers in primitive myxobacteria: Cytophaga hutchinsonii and Sporocytophaga myxococcoides. Folia Microbiol (Praha) 1967;12(3):248–254. doi: 10.1007/BF02868739. [DOI] [PubMed] [Google Scholar]
- Wehrli W., Staehelin M. Actions of the rifamycins. Bacteriol Rev. 1971 Sep;35(3):290–309. doi: 10.1128/br.35.3.290-309.1971. [DOI] [PMC free article] [PubMed] [Google Scholar]