Abstract
The nature of the energy requirement for irreversible adsorption of phages T1 and phi80 was studied by using various specific energy inhibitors and mutants lacking either the Ca2+, Mg2+-adenosine triphosphatase or the ability to produce cytochromes in the absence of added 5-aminolaevulinic acid. It was found that irreversible adsorption could be energized both through the electron transport chain and from adenosine 5'-triphosphate via the Ca2+, Mg2+-adenosine triphosphatase, indicating the involvement of the energized membrane state. These results and the discovery that phages T1 and phi80 adsorb reversibly to the isolated tonA gene product are discussed in terms of the possible involvement of functions expressed by the tonB gene region in irreversible adsorption and the relationship to iron transport.
Full text
PDF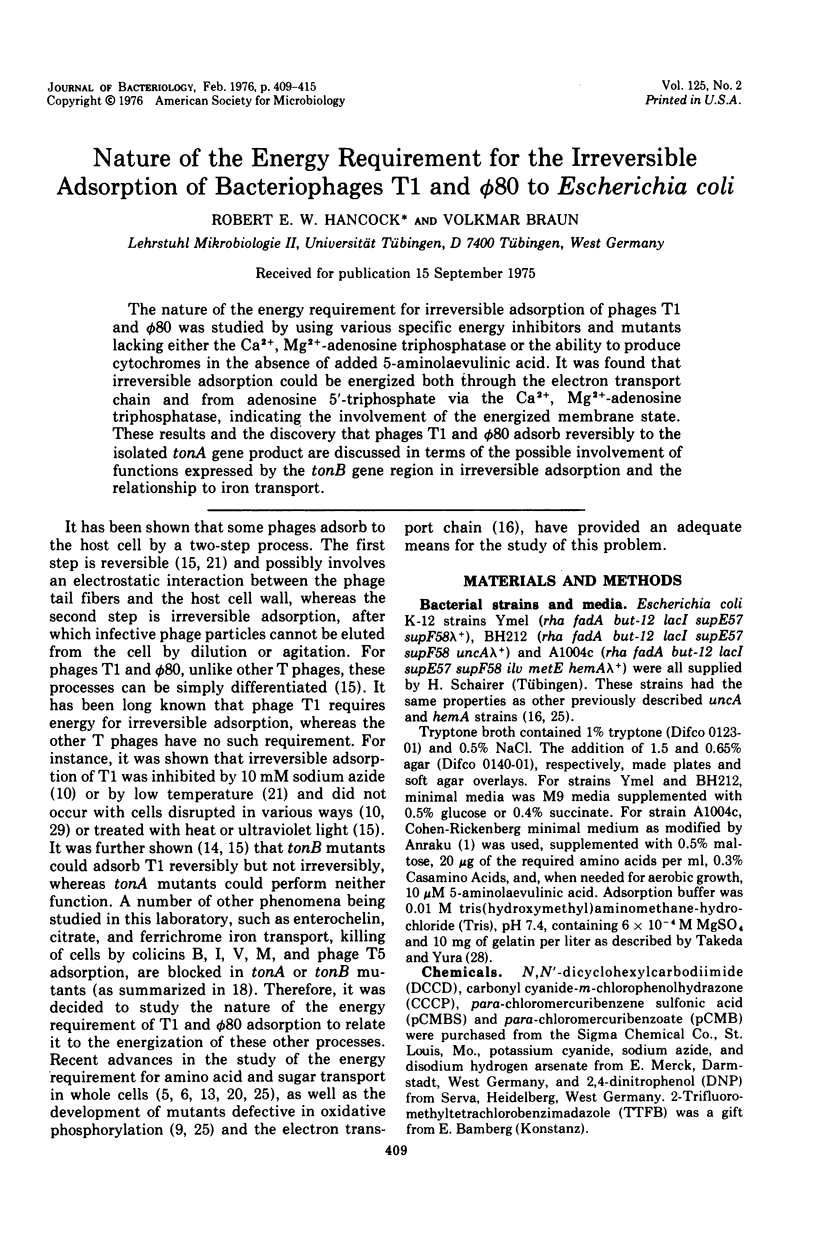
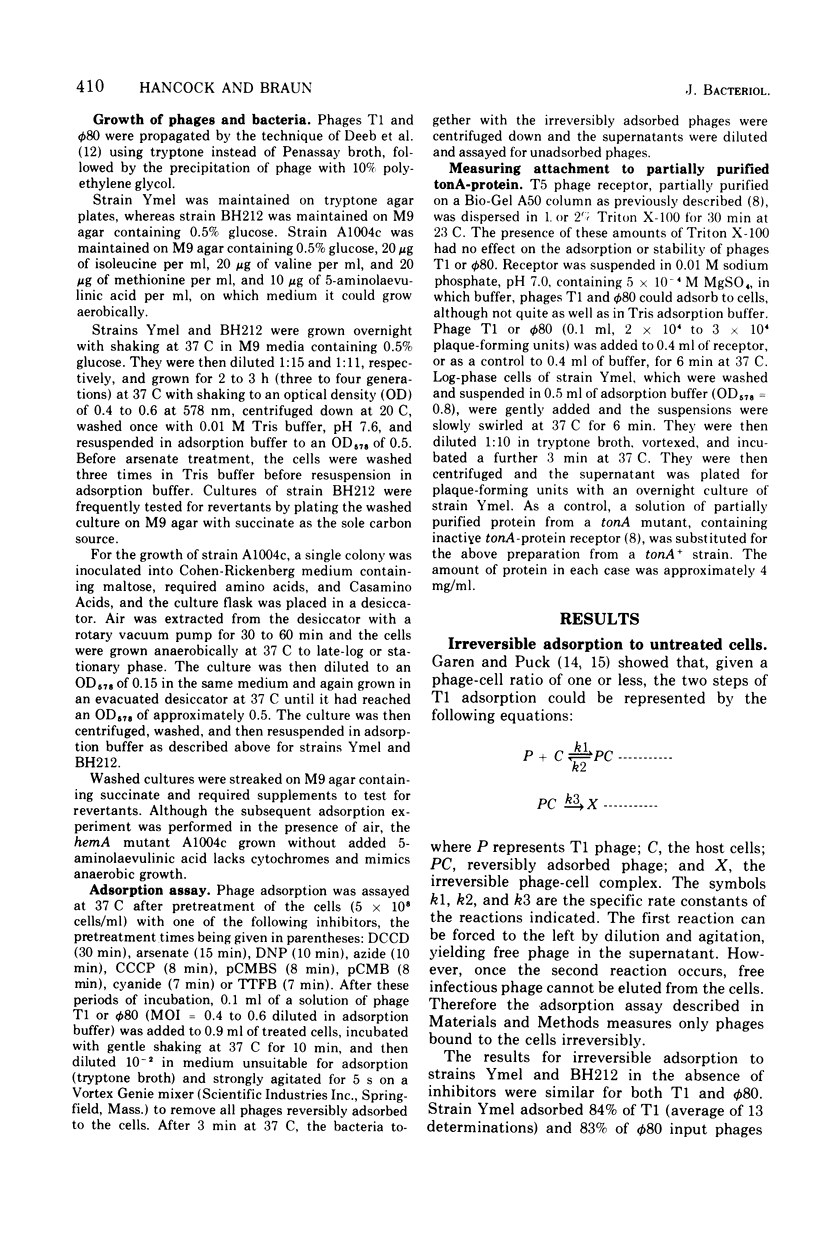
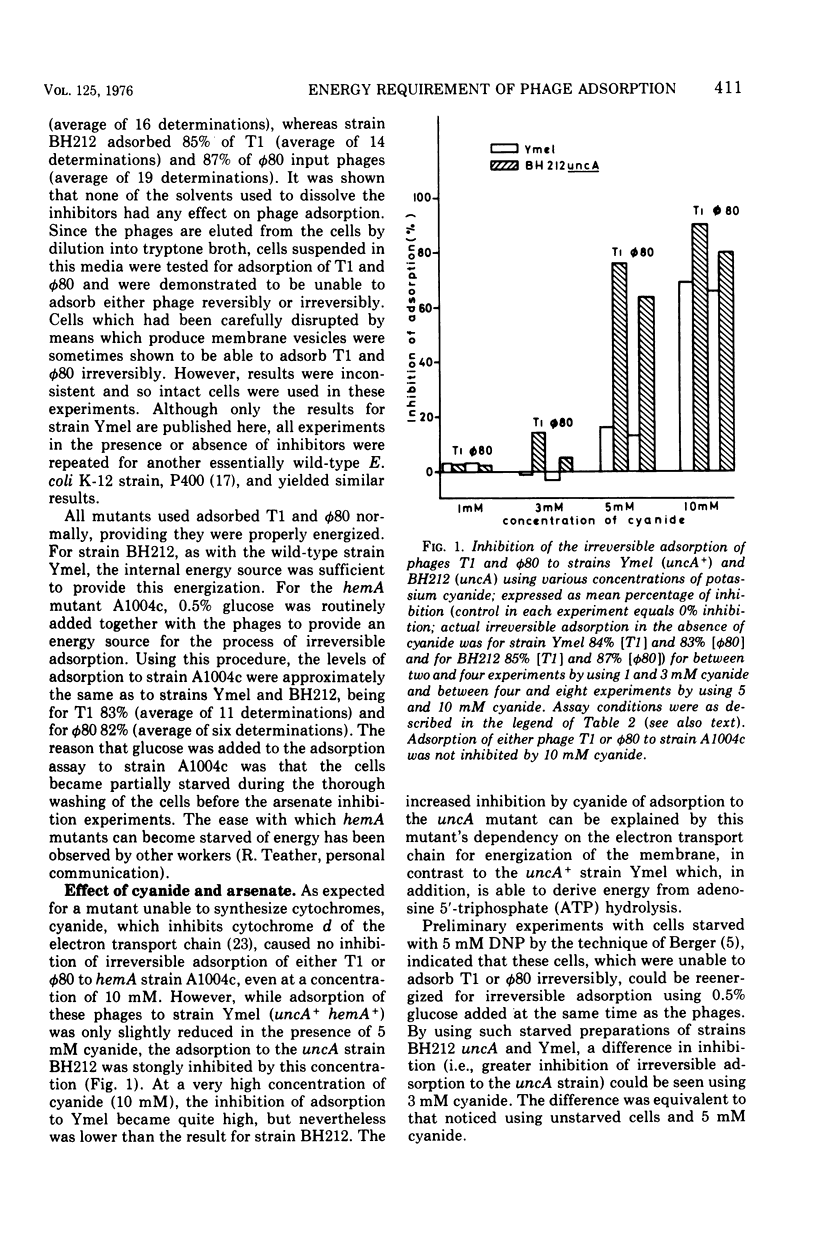
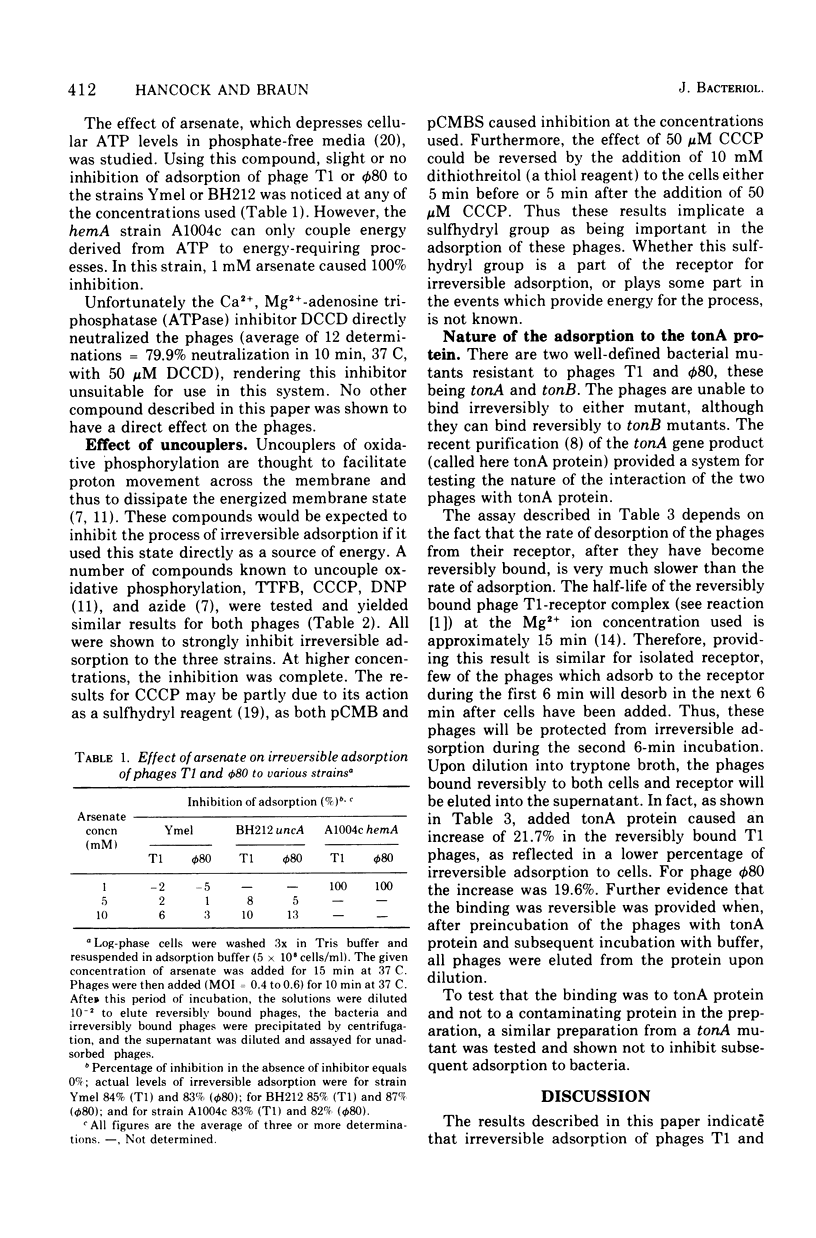
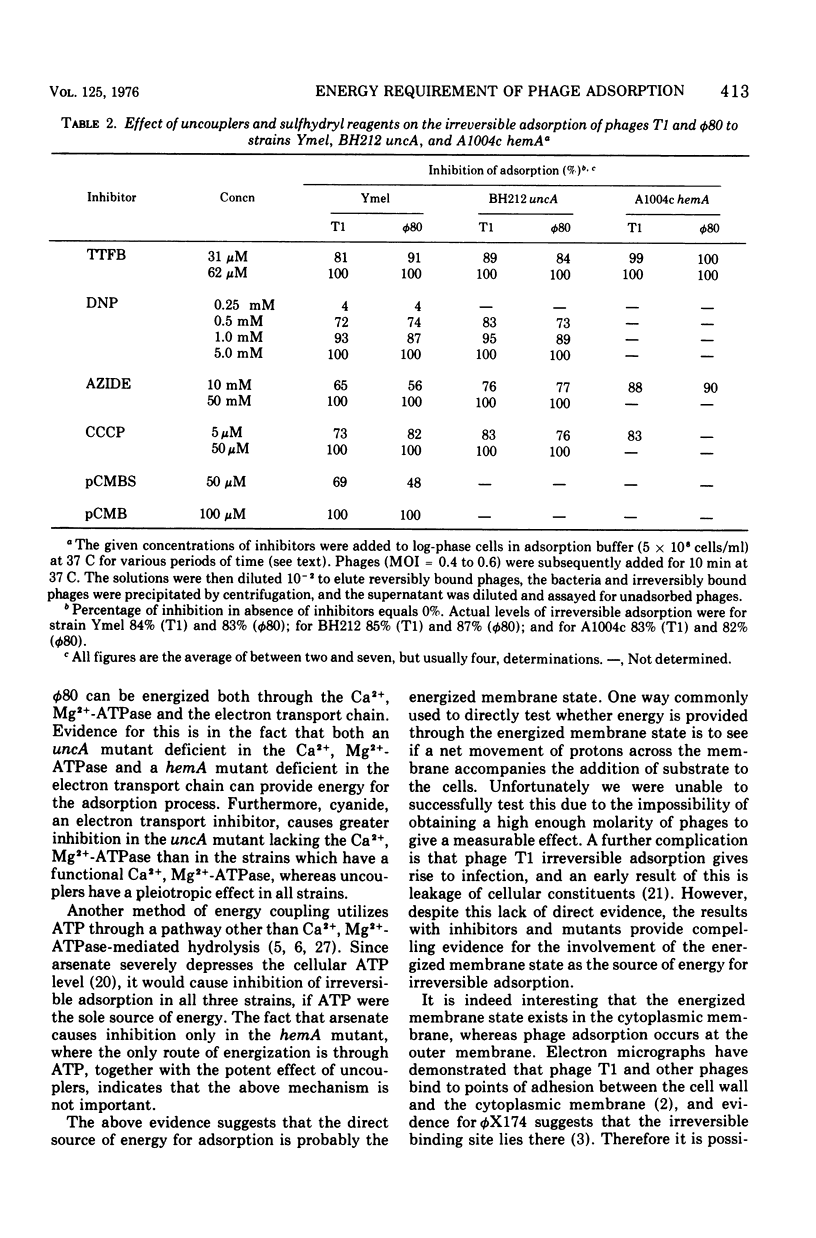
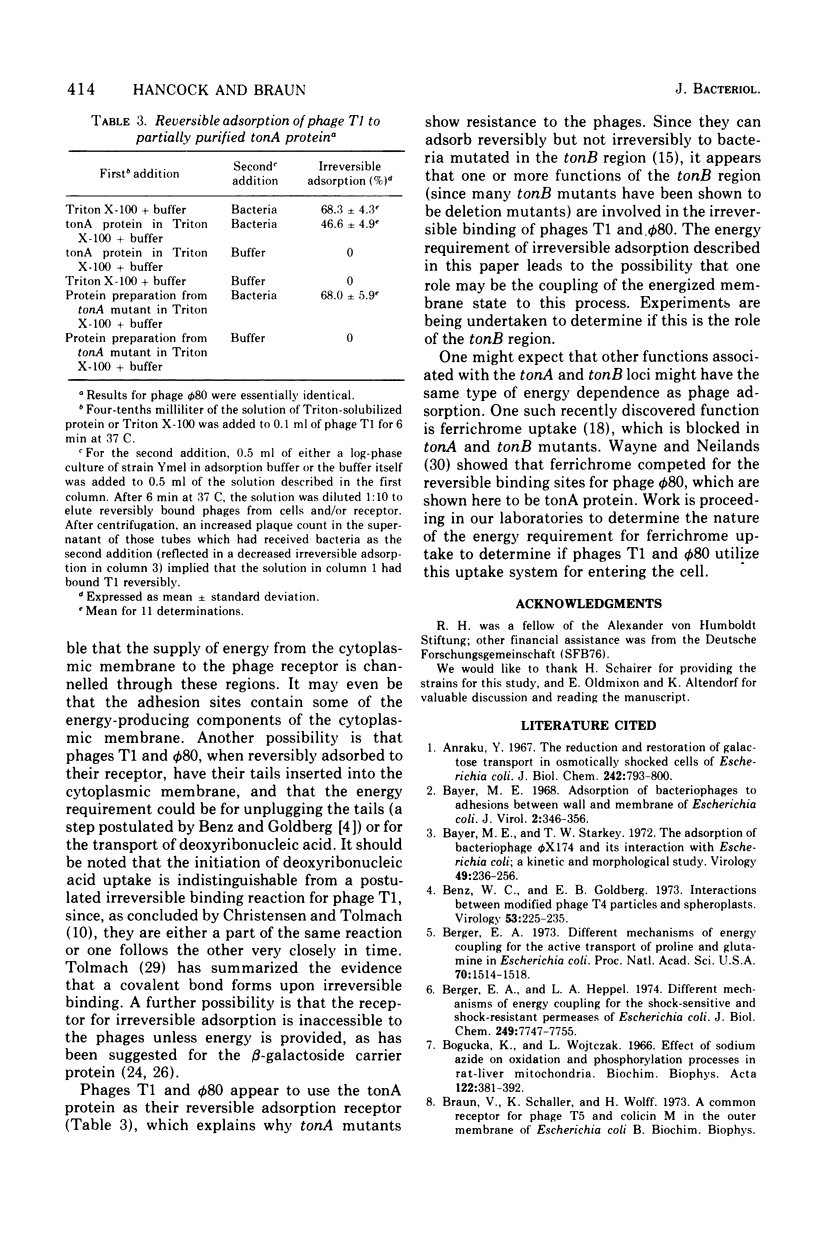
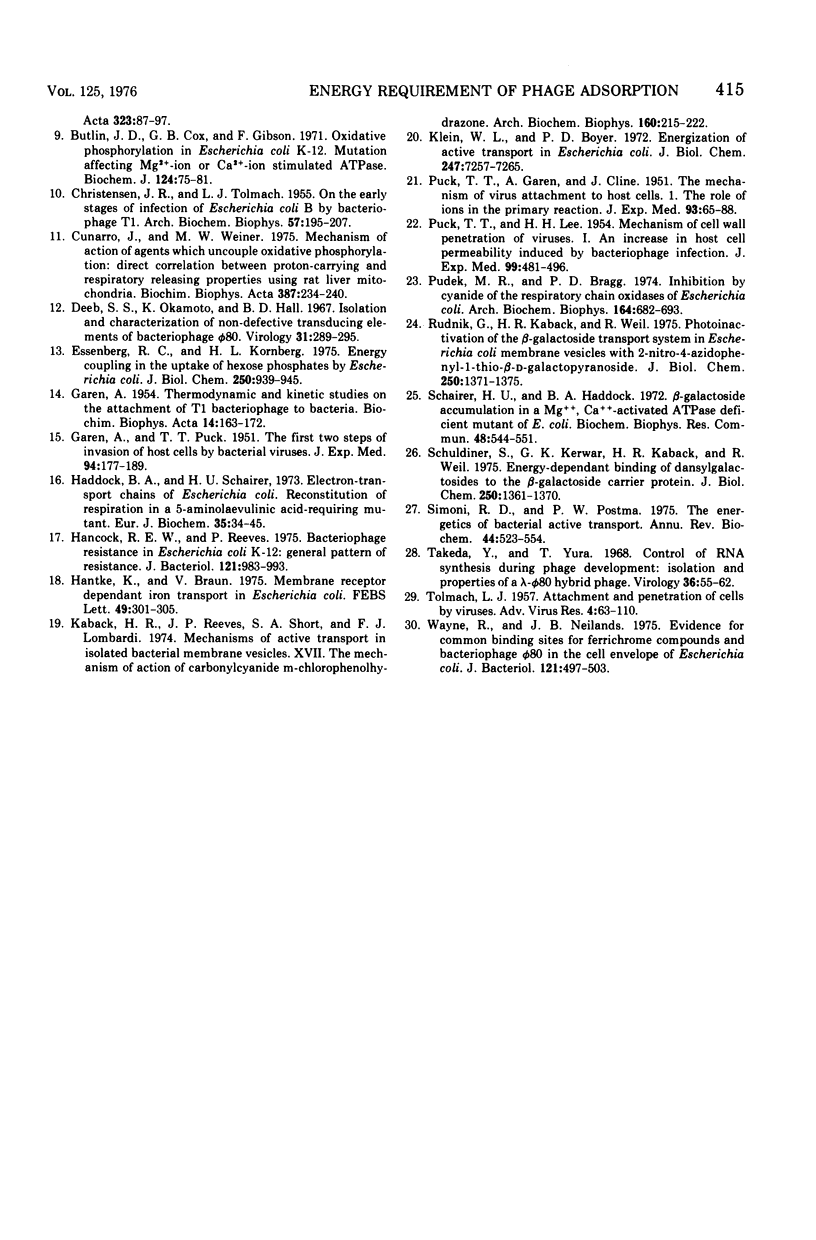
Selected References
These references are in PubMed. This may not be the complete list of references from this article.
- Anraku Y. The reduction and restoration of galactose transport in osmotically shocked cells of Escherichia coli. J Biol Chem. 1967 Mar 10;242(5):793–800. [PubMed] [Google Scholar]
- Bayer M. E. Adsorption of bacteriophages to adhesions between wall and membrane of Escherichia coli. J Virol. 1968 Apr;2(4):346–356. doi: 10.1128/jvi.2.4.346-356.1968. [DOI] [PMC free article] [PubMed] [Google Scholar]
- Bayer M. E., Starkey T. W. The adsorption of bacteriophage phi X174 and its interaction with Escherichia coli; a kinetic and morphological study. Virology. 1972 Jul;49(1):236–256. doi: 10.1016/s0042-6822(72)80026-6. [DOI] [PubMed] [Google Scholar]
- Benz W. C., Goldberg E. B. Interactions between modified phage T4 particles and spheroplasts. Virology. 1973 May;53(1):225–235. doi: 10.1016/0042-6822(73)90481-9. [DOI] [PubMed] [Google Scholar]
- Berger E. A. Different mechanisms of energy coupling for the active transport of proline and glutamine in Escherichia coli. Proc Natl Acad Sci U S A. 1973 May;70(5):1514–1518. doi: 10.1073/pnas.70.5.1514. [DOI] [PMC free article] [PubMed] [Google Scholar]
- Berger E. A., Heppel L. A. Different mechanisms of energy coupling for the shock-sensitive and shock-resistant amino acid permeases of Escherichia coli. J Biol Chem. 1974 Dec 25;249(24):7747–7755. [PubMed] [Google Scholar]
- Butlin J. D., Cox G. B., Gibson F. Oxidative phosphorylation in Escherichia coli K12. Mutations affecting magnesium ion- or calcium ion-stimulated adenosine triphosphatase. Biochem J. 1971 Aug;124(1):75–81. doi: 10.1042/bj1240075. [DOI] [PMC free article] [PubMed] [Google Scholar]
- CHRISTENSEN J. R., TOLMACH L. J. On the early stages of infection of Escherichia coli B by bacteriophage T1. Arch Biochem Biophys. 1955 Jul;57(1):195–207. doi: 10.1016/0003-9861(55)90192-2. [DOI] [PubMed] [Google Scholar]
- Cunarro J., Weiner M. W. Mechanism of action of agents which uncouple oxidative phosphorylation: direct correlation between proton-carrying and respiratory-releasing properties using rat liver mitochondria. Biochim Biophys Acta. 1975 May 15;387(2):234–240. doi: 10.1016/0005-2728(75)90106-1. [DOI] [PubMed] [Google Scholar]
- Deeb S. S., Okamoto K., Hall B. D. Isolation and characterization of non-defective transducing elements of bacteriophage phi-80. Virology. 1967 Feb;31(2):289–295. doi: 10.1016/0042-6822(67)90173-0. [DOI] [PubMed] [Google Scholar]
- Essenberg R. C., Kornberg H. L. Energy coupling in the uptake of hexose phosphates by Escherichia coli. J Biol Chem. 1975 Feb 10;250(3):939–945. [PubMed] [Google Scholar]
- GAREN A., PUCK T. T. The first two steps of the invasion of host cells by bacterial viruses. II. J Exp Med. 1951 Sep;94(3):177–189. doi: 10.1084/jem.94.3.177. [DOI] [PMC free article] [PubMed] [Google Scholar]
- GAREN A. Thermodynamic and kinetic studies on the attachment of T1 bacteriophage to bacteria. Biochim Biophys Acta. 1954 Jun;14(2):163–172. doi: 10.1016/0006-3002(54)90155-9. [DOI] [PubMed] [Google Scholar]
- Haddock B. A., Schairer H. U. Electron-transport chains of Escherichia coli. Reconstitution of respiration in a 5-aminolaevulinic acid-requiring mutant. Eur J Biochem. 1973 May;35(1):34–45. doi: 10.1111/j.1432-1033.1973.tb02806.x. [DOI] [PubMed] [Google Scholar]
- Hancock R. E., Reeves P. Bacteriophage resistance in Escherichia coli K-12: general pattern of resistance. J Bacteriol. 1975 Mar;121(3):983–993. doi: 10.1128/jb.121.3.983-993.1975. [DOI] [PMC free article] [PubMed] [Google Scholar]
- Hantke K., Braun V. Membrane receptor dependent iron transport in Escherichia coli. FEBS Lett. 1975 Jan 1;49(3):301–305. doi: 10.1016/0014-5793(75)80771-x. [DOI] [PubMed] [Google Scholar]
- Kaback H. R., Reeves J. P., Short S. A., Lombardi F. J. Mechanisms of active transport in isolated bacterial membrane vesicles. 18. The mechanism of action of carbonylcyanide m-chlorophenylhydrazone. Arch Biochem Biophys. 1974 Jan;160(1):215–222. doi: 10.1016/s0003-9861(74)80028-7. [DOI] [PubMed] [Google Scholar]
- Klein W. L., Boyer P. D. Energization of active transport by Escherichia coli. J Biol Chem. 1972 Nov 25;247(22):7257–7265. [PubMed] [Google Scholar]
- PUCK T. T., GAREN A., CLINE J. The mechanism of virus attachment to host cells. I. The role of ions in the primary reaction. J Exp Med. 1951 Jan;93(1):65–88. doi: 10.1084/jem.93.1.65. [DOI] [PMC free article] [PubMed] [Google Scholar]
- PUCK T. T., LEE H. H. Mechanism of cell wall penetration by viruses. I. An increase in host cell permeability induced by bacteriophage infection. J Exp Med. 1954 May 1;99(5):481–494. doi: 10.1084/jem.99.5.481. [DOI] [PMC free article] [PubMed] [Google Scholar]
- Pudek M. R., Bragg P. D. Inhibition by cyanide of the respiratory chain oxidases of Escherichia coli. Arch Biochem Biophys. 1974 Oct;164(2):682–693. doi: 10.1016/0003-9861(74)90081-2. [DOI] [PubMed] [Google Scholar]
- Rudnick G., Kaback H. R., Weil R. Photoinactivation of the beta-galactoside transport system in Escherichia coli membrane vesicles with 2-nitro-4-azidophenyl-1-thio-beta-D-galactopyranoside. J Biol Chem. 1975 Feb 25;250(4):1371–1375. [PubMed] [Google Scholar]
- Schairer H. U., Haddock B. A. -Galactoside accumulation in a Mg 2+ -,Ca 2+ -activated ATPase deficient mutant of E.coli. Biochem Biophys Res Commun. 1972 Aug 7;48(3):544–551. doi: 10.1016/0006-291x(72)90382-8. [DOI] [PubMed] [Google Scholar]
- Schuldiner S., Kerwar G. K., Kaback H. R., Weil R. Energy-dependent binding of dansylgalactosides to the beta-galactoside carrier protein. J Biol Chem. 1975 Feb 25;250(4):1361–1370. [PubMed] [Google Scholar]
- Simoni R. D., Postma P. W. The energetics of bacterial active transport. Annu Rev Biochem. 1975;44:523–554. doi: 10.1146/annurev.bi.44.070175.002515. [DOI] [PubMed] [Google Scholar]
- TOLMACH L. J. Attachment and penetration of cells by viruses. Adv Virus Res. 1957;4:63–110. doi: 10.1016/s0065-3527(08)60596-5. [DOI] [PubMed] [Google Scholar]
- Takeda Y., Yura T. Control of RNA synthesis during phage development: isolation and properties of a lambda-phi81 hybrid phage. Virology. 1968 Sep;36(1):55–62. doi: 10.1016/0042-6822(68)90116-5. [DOI] [PubMed] [Google Scholar]
- Wayne R., Neilands J. B. Evidence for common binding sites for ferrichrome compounds and bacteriophage phi 80 in the cell envelope of Escherichia coli. J Bacteriol. 1975 Feb;121(2):497–503. doi: 10.1128/jb.121.2.497-503.1975. [DOI] [PMC free article] [PubMed] [Google Scholar]