Abstract
Adaptation of Acinetobacter calcoaceticus from river water to aniline depends on the dynamics of parent and mutant populations. The parent, Acinetobacter strain DON26 phenotype Ani0, was common in river water and assimilated aniline effectively at micromolar concentrations, but was inhibited at higher concentrations of aniline. The Ani0 phenotype was also characterized by a broad specificity for oxidation of chloroanilines by aniline-induced cells. The mutant Ani+ phenotype was represented by DON2, isolated from a population of less than 100 cells ml-1 in a mixed river water culture, and by DON261, isolated during continuous culture of DON26. Ani+ strains assimilated aniline at a greater maximum specific rate than the parent and were able to grow at concentrations of aniline greater than 16 mM. These strains cooxidized phenol after growth at high aniline concentrations, but showed reduced activity toward chloroanilines. These changes plus kinetic data, oxygen uptake data, and the results of auxanography indicate that the mutant has an increased activity and altered specificity of the initial enzyme in the aniline catabolic pathway. The parent strain, DON26, was at a selective advantage relative to the mutant at low concentrations of aniline, but was replaced by the mutant when aniline concentrations increased. Adaptation of the mixed river water community to aniline involved selection of both phenotypes. Reversion of the Ani+ to Ani0 phenotype occurred at a frequency of 10(-2) in the absence of aniline selection. Plasmid content was not altered during either acquisition or loss of the Ani+ phenotype. Adaptive changes in Acinetobacter spp. populations illustrate important differences in the catabolic activities of natural and pollutant selected strains.(ABSTRACT TRUNCATED AT 250 WORDS)
Full text
PDF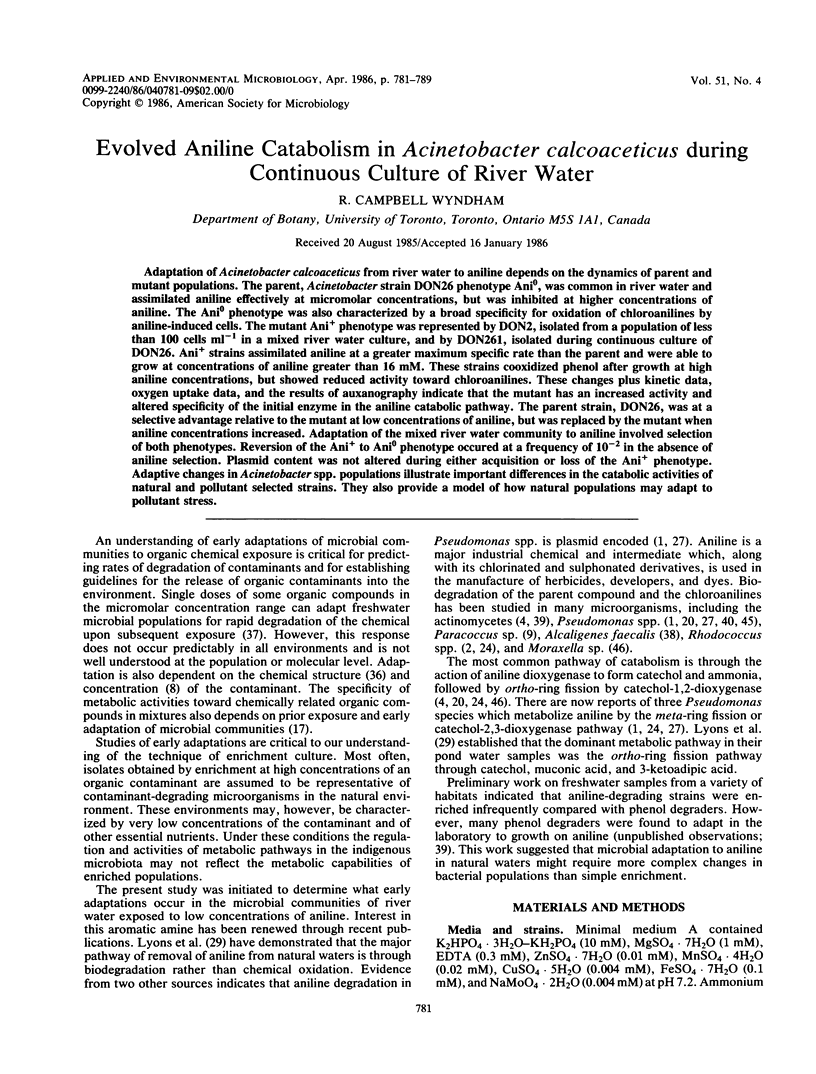
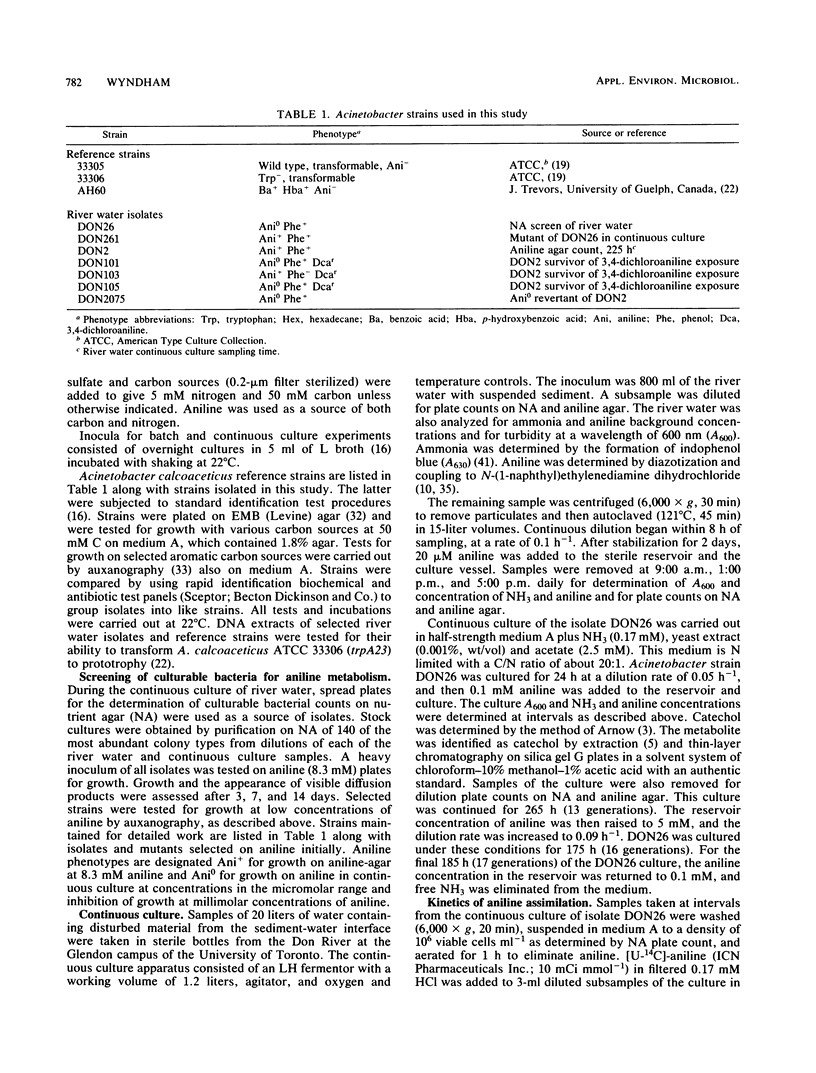
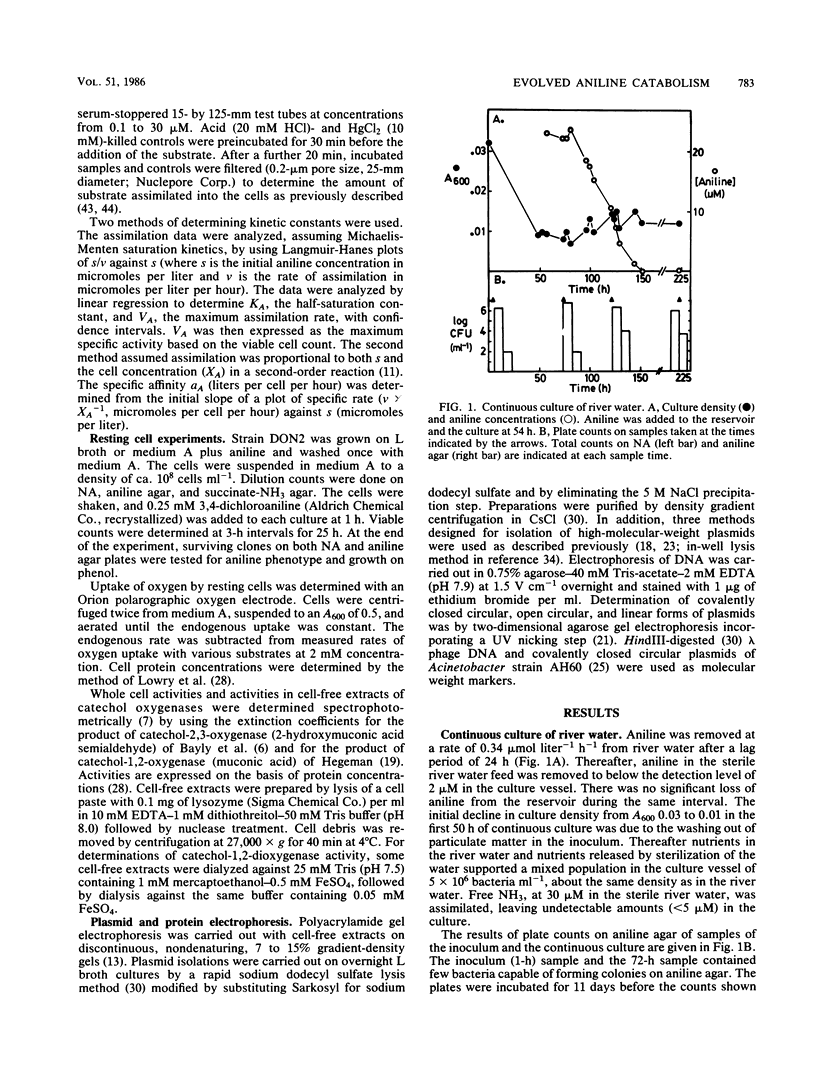
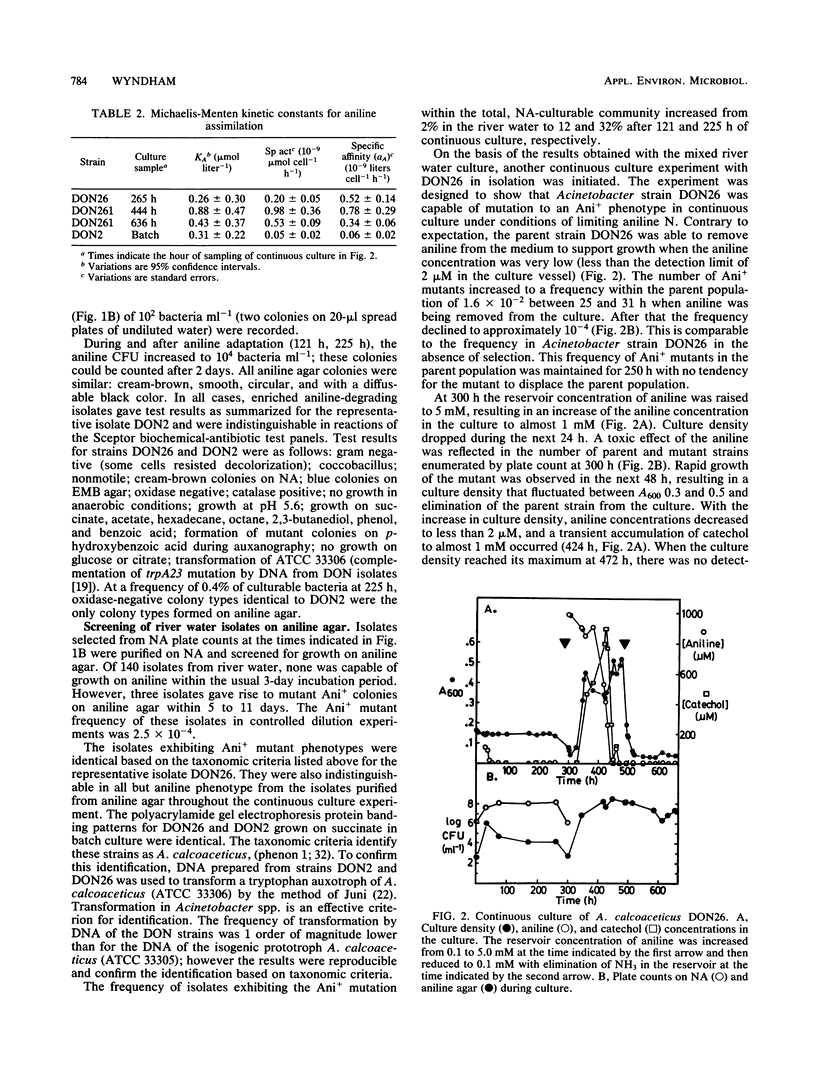
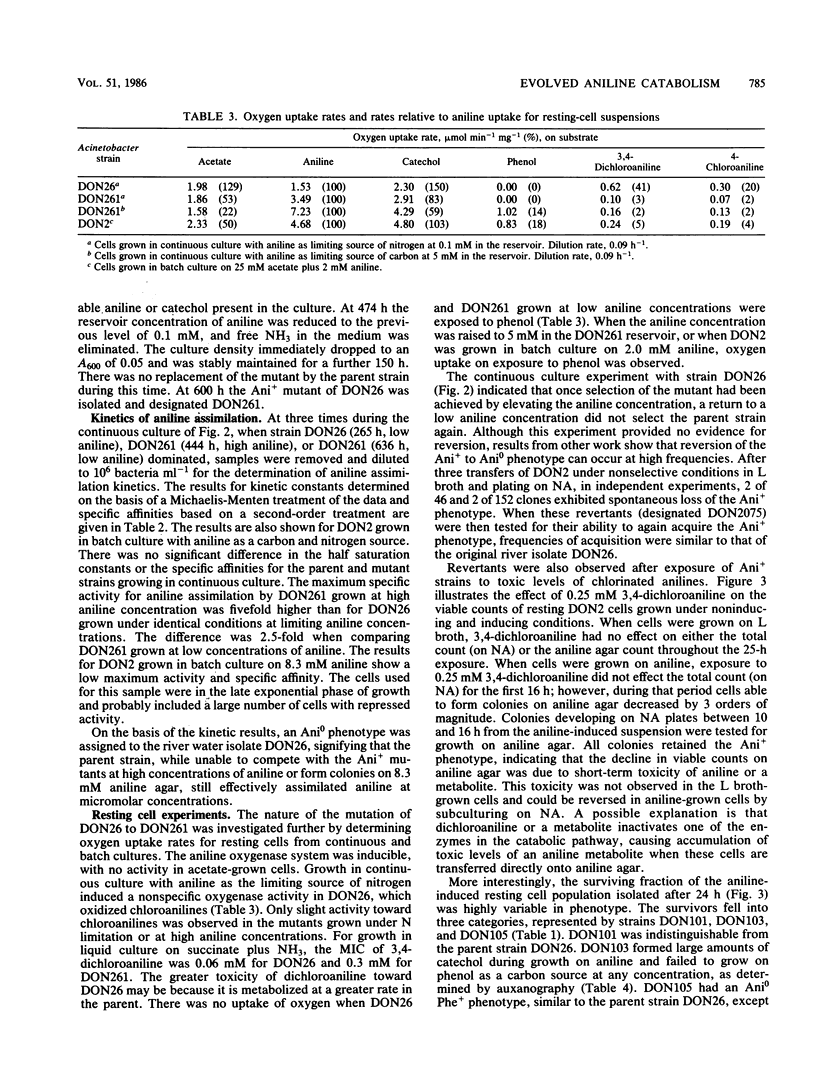
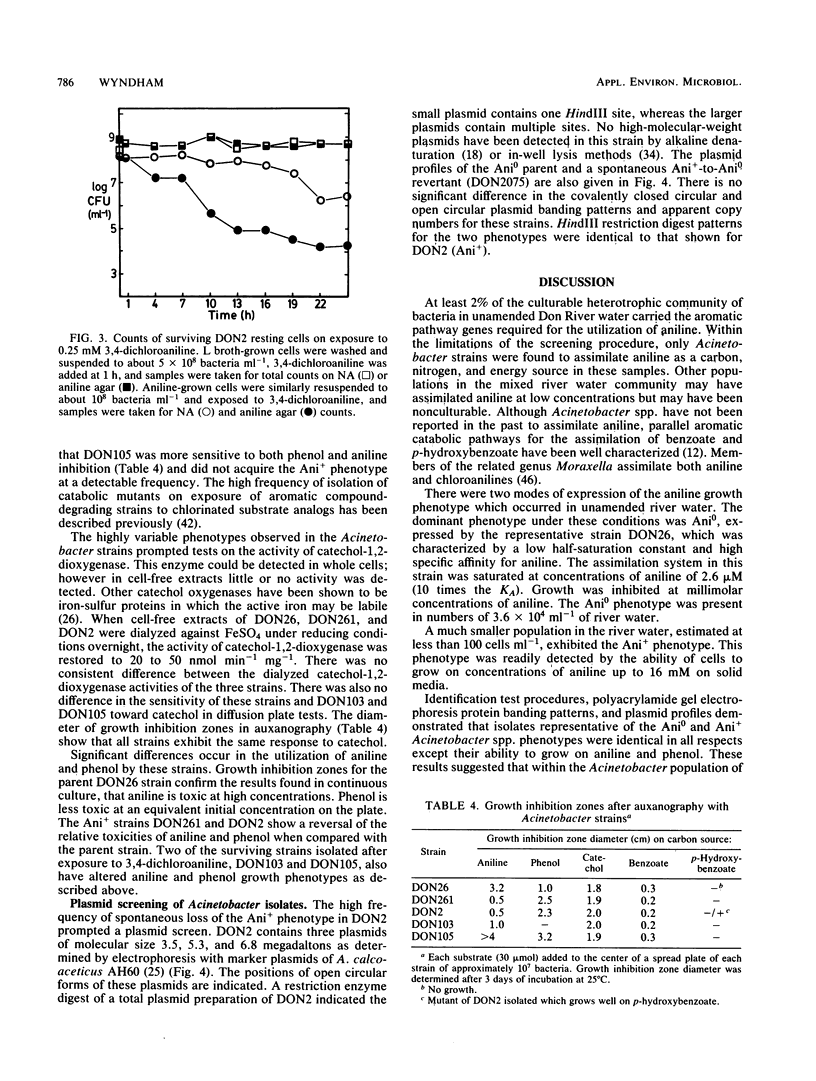
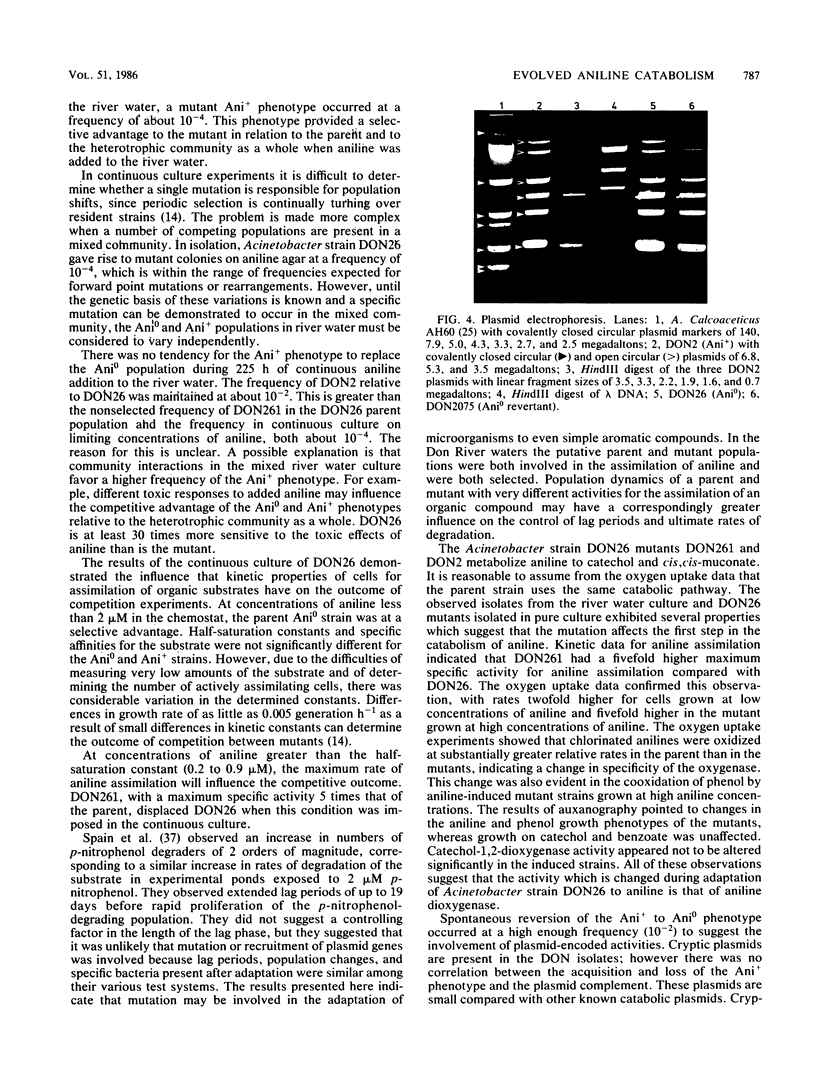
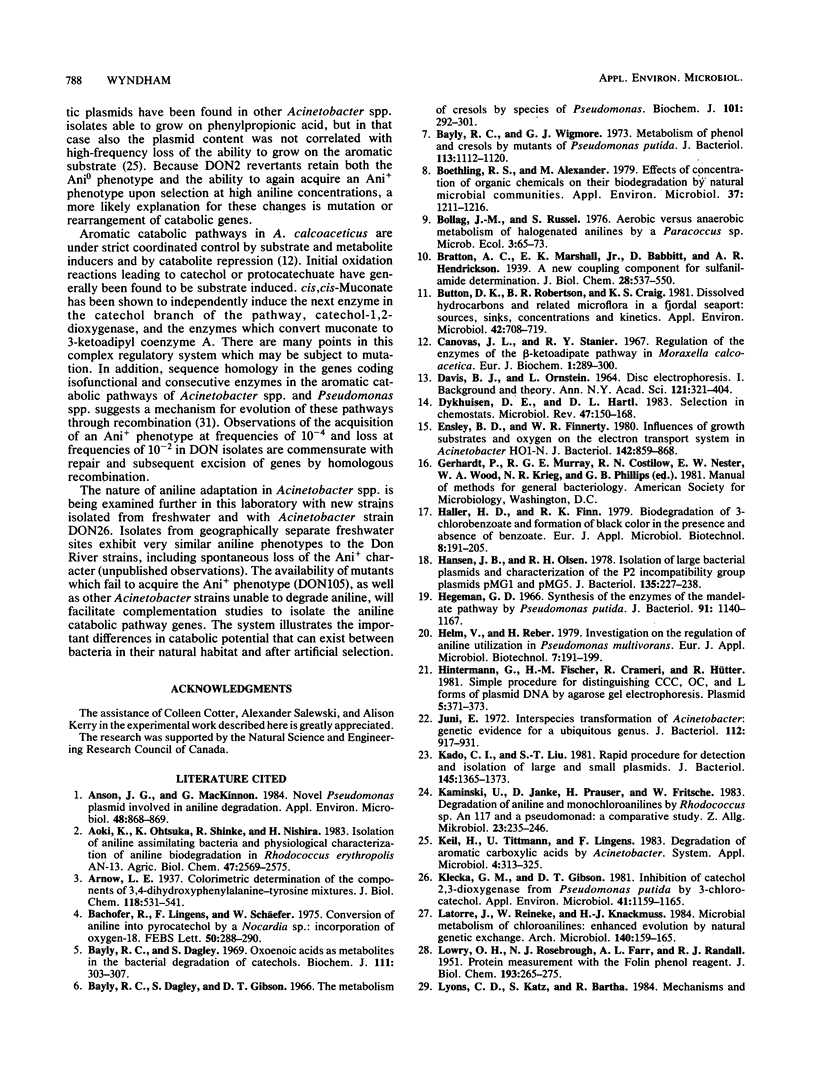
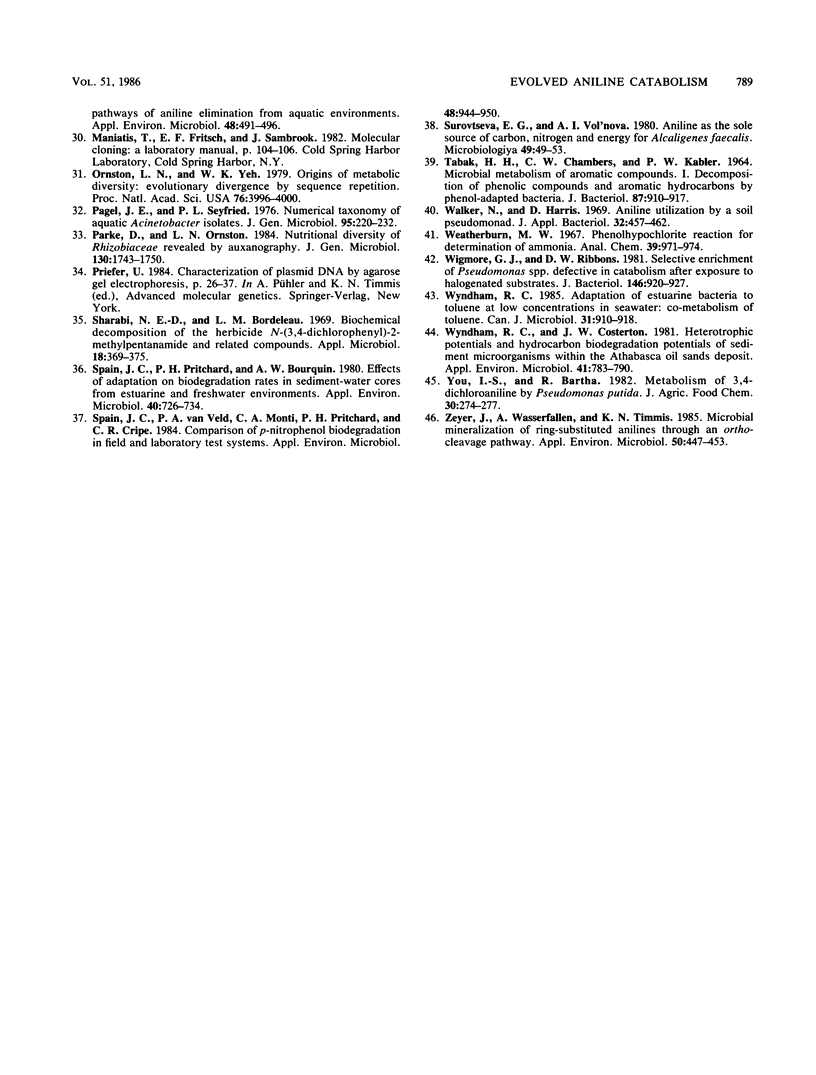
Images in this article
Selected References
These references are in PubMed. This may not be the complete list of references from this article.
- Anson J. G., Mackinnon G. Novel pseudomonas plasmid involved in aniline degradation. Appl Environ Microbiol. 1984 Oct;48(4):868–869. doi: 10.1128/aem.48.4.868-869.1984. [DOI] [PMC free article] [PubMed] [Google Scholar]
- Bachofer R., Lingens F., Schäfer W. Conversion of aniline into pyrocatechol by a Nocardia sp.; incorporation of oxygen-18. FEBS Lett. 1975 Feb 1;50(2):288–290. doi: 10.1016/0014-5793(75)80510-2. [DOI] [PubMed] [Google Scholar]
- Bayly R. C., Dagley S., Gibson D. T. The metabolism of cresols by species of Pseudomonas. Biochem J. 1966 Nov;101(2):293–301. doi: 10.1042/bj1010293. [DOI] [PMC free article] [PubMed] [Google Scholar]
- Bayly R. C., Dagley S. Oxoenoic acids as metabolites in the bacterial degradation of catechols. Biochem J. 1969 Feb;111(3):303–307. doi: 10.1042/bj1110303. [DOI] [PMC free article] [PubMed] [Google Scholar]
- Bayly R. C., Wigmore G. J. Metabolism of phenol and cresols by mutants of Pseudomonas putida. J Bacteriol. 1973 Mar;113(3):1112–1120. doi: 10.1128/jb.113.3.1112-1120.1973. [DOI] [PMC free article] [PubMed] [Google Scholar]
- Boethling R. S., Alexander M. Effect of concentration of organic chemicals on their biodegradation by natural microbial communities. Appl Environ Microbiol. 1979 Jun;37(6):1211–1216. doi: 10.1128/aem.37.6.1211-1216.1979. [DOI] [PMC free article] [PubMed] [Google Scholar]
- Button D. K., Robertson B. R., Craig K. S. Dissolved hydrocarbons and related microflora in a fjordal seaport: sources, sinks, concentrations, and kinetics. Appl Environ Microbiol. 1981 Oct;42(4):708–719. doi: 10.1128/aem.42.4.708-719.1981. [DOI] [PMC free article] [PubMed] [Google Scholar]
- Cánovas J. L., Stanier R. Y. Regulation of the enzymes of the beta-ketoadipate pathway in Moraxella calcoacetica. 1. General aspects. Eur J Biochem. 1967 May;1(3):289–300. doi: 10.1007/978-3-662-25813-2_40. [DOI] [PubMed] [Google Scholar]
- Dykhuizen D. E., Hartl D. L. Selection in chemostats. Microbiol Rev. 1983 Jun;47(2):150–168. doi: 10.1128/mr.47.2.150-168.1983. [DOI] [PMC free article] [PubMed] [Google Scholar]
- Ensley B. D., Jr, Finnerty W. R. Influences of growth substrates and oxygen on the electron transport system in Acinetobacter sp. HO1-N. J Bacteriol. 1980 Jun;142(3):859–868. doi: 10.1128/jb.142.3.859-868.1980. [DOI] [PMC free article] [PubMed] [Google Scholar]
- Hansen J. B., Olsen R. H. Isolation of large bacterial plasmids and characterization of the P2 incompatibility group plasmids pMG1 and pMG5. J Bacteriol. 1978 Jul;135(1):227–238. doi: 10.1128/jb.135.1.227-238.1978. [DOI] [PMC free article] [PubMed] [Google Scholar]
- Hegeman G. D. Synthesis of the enzymes of the mandelate pathway by Pseudomonas putida. I. Synthesis of enzymes by the wild type. J Bacteriol. 1966 Mar;91(3):1140–1154. doi: 10.1128/jb.91.3.1140-1154.1966. [DOI] [PMC free article] [PubMed] [Google Scholar]
- Hintermann G., Fischer H. M., Crameri R., Hütter R. Simple procedure for distinguishing CCC, OC, and L forms of plasmid DNA by agarose gel electrophoresis. Plasmid. 1981 May;5(3):371–373. doi: 10.1016/0147-619x(81)90012-3. [DOI] [PubMed] [Google Scholar]
- Juni E. Interspecies transformation of Acinetobacter: genetic evidence for a ubiquitous genus. J Bacteriol. 1972 Nov;112(2):917–931. doi: 10.1128/jb.112.2.917-931.1972. [DOI] [PMC free article] [PubMed] [Google Scholar]
- Kado C. I., Liu S. T. Rapid procedure for detection and isolation of large and small plasmids. J Bacteriol. 1981 Mar;145(3):1365–1373. doi: 10.1128/jb.145.3.1365-1373.1981. [DOI] [PMC free article] [PubMed] [Google Scholar]
- Kaminski U., Janke D., Prauser H., Fritsche W. Degradation of aniline and monochloroanilines by Rhodococcus sp. An 117 and a pseudomonad: a comparative study. Z Allg Mikrobiol. 1983;23(4):235–246. doi: 10.1002/jobm.3630230405. [DOI] [PubMed] [Google Scholar]
- Klecka G. M., Gibson D. T. Inhibition of catechol 2,3-dioxygenase from Pseudomonas putida by 3-chlorocatechol. Appl Environ Microbiol. 1981 May;41(5):1159–1165. doi: 10.1128/aem.41.5.1159-1165.1981. [DOI] [PMC free article] [PubMed] [Google Scholar]
- LOWRY O. H., ROSEBROUGH N. J., FARR A. L., RANDALL R. J. Protein measurement with the Folin phenol reagent. J Biol Chem. 1951 Nov;193(1):265–275. [PubMed] [Google Scholar]
- Lyons C. D., Katz S., Bartha R. Mechanisms and pathways of aniline elimination from aquatic environments. Appl Environ Microbiol. 1984 Sep;48(3):491–496. doi: 10.1128/aem.48.3.491-496.1984. [DOI] [PMC free article] [PubMed] [Google Scholar]
- ORNSTEIN L. DISC ELECTROPHORESIS. I. BACKGROUND AND THEORY. Ann N Y Acad Sci. 1964 Dec 28;121:321–349. doi: 10.1111/j.1749-6632.1964.tb14207.x. [DOI] [PubMed] [Google Scholar]
- Ornston L. N., Yeh W. K. Origins of metabolic diversity: evolutionary divergence by sequence repetition. Proc Natl Acad Sci U S A. 1979 Aug;76(8):3996–4000. doi: 10.1073/pnas.76.8.3996. [DOI] [PMC free article] [PubMed] [Google Scholar]
- Pagel J. E., Seyfried P. L. Numerical taxonomy of aquatic Acinetobacter isolates. J Gen Microbiol. 1976 Aug;96(2):220–232. doi: 10.1099/00221287-95-2-220. [DOI] [PubMed] [Google Scholar]
- Sharabi N. E., Bordeleau L. M. Biochemical decomposition of the herbicide N-(3,4-dichlorophenyl)-2-methylpentanamide and related compounds. Appl Microbiol. 1969 Sep;18(3):369–375. doi: 10.1128/am.18.3.369-375.1969. [DOI] [PMC free article] [PubMed] [Google Scholar]
- Spain J. C., Pritchard P. H., Bourquin A. W. Effects of adaptation on biodegradation rates in sediment/water cores from estuarine and freshwater environments. Appl Environ Microbiol. 1980 Oct;40(4):726–734. doi: 10.1128/aem.40.4.726-734.1980. [DOI] [PMC free article] [PubMed] [Google Scholar]
- Spain J. C., Van Veld P. A., Monti C. A., Pritchard P. H., Cripe C. R. Comparison of p-Nitrophenol Biodegradation in Field and Laboratory Test Systems. Appl Environ Microbiol. 1984 Nov;48(5):944–950. doi: 10.1128/aem.48.5.944-950.1984. [DOI] [PMC free article] [PubMed] [Google Scholar]
- Surovtseva E. G., Vol'nova A. I. Anilin kak edinstvennyi istochnik ugleroda, azota i énergii dlia Alcaligenes faecalis. Mikrobiologiia. 1980 Jan-Feb;49(1):49–53. [PubMed] [Google Scholar]
- TABAK H. H., CHAMBERS C. W., KABLER P. W. MICROBIAL METABOLISM OF AROMATIC COMPOUNDS. I. DECOMPOSITION OF PHENOLIC COMPOUNDS AND AROMATIC HYDROCARBONS BY PHENOL-ADAPTED BACTERIA. J Bacteriol. 1964 Apr;87:910–919. doi: 10.1128/jb.87.4.910-919.1964. [DOI] [PMC free article] [PubMed] [Google Scholar]
- Wigmore G. J., Ribbons D. W. Selective enrichment of Pseudomonas spp. defective in catabolism after exposure to halogenated substrates. J Bacteriol. 1981 Jun;146(3):920–927. doi: 10.1128/jb.146.3.920-927.1981. [DOI] [PMC free article] [PubMed] [Google Scholar]
- Wyndham R. C., Costerton J. W. Heterotrophic potentials and hydrocarbon biodegradation potentials of sediment microorganisms within the athabasca oil sands deposit. Appl Environ Microbiol. 1981 Mar;41(3):783–790. doi: 10.1128/aem.41.3.783-790.1981. [DOI] [PMC free article] [PubMed] [Google Scholar]
- Zeyer J., Wasserfallen A., Timmis K. N. Microbial mineralization of ring-substituted anilines through an ortho-cleavage pathway. Appl Environ Microbiol. 1985 Aug;50(2):447–453. doi: 10.1128/aem.50.2.447-453.1985. [DOI] [PMC free article] [PubMed] [Google Scholar]