Abstract
Saccharomyces cerevisiae ATCC 4126 was grown within the macroporous matrix of asymmetric-walled polysulfone hollow-fiber membranes and on the exterior surfaces of isotropic-walled polypropylene hollow-fiber membranes. Nutrients were supplied and products were removed by single-pass perfusion of the fiber lumens. Growth of yeast cells within the macrovoids of the asymmetric-walled membranes attained densities of greater than 1010 cells per ml and in some regions accounted for nearly 100% of the available macrovoid volume, forming a tissue-like mass. A radial distribution of cell packing existed across the fiber wall, indicating an inadequate glucose supply to cells located beyond 100 μm from the lumen surface. By comparison, yeast cell growth on the exterior surfaces of the isotropic-walled membranes resulted in an average density of 3.5 × 109 viable cells per ml. Ethanol production by reactors containing isotropic polypropylene fibers reached a maximum value of 26 g/liter-h based on the total reactor volume. Reactor performance depended on the fiber packing density and on the glucose medium flow rate and was limited by low nutrient and product transport rates. The inhibition of ethanol production and the reduction in fermentation efficiency arose primarily from the accumulation of CO2 gas within the sealed reactor shell space.
Full text
PDF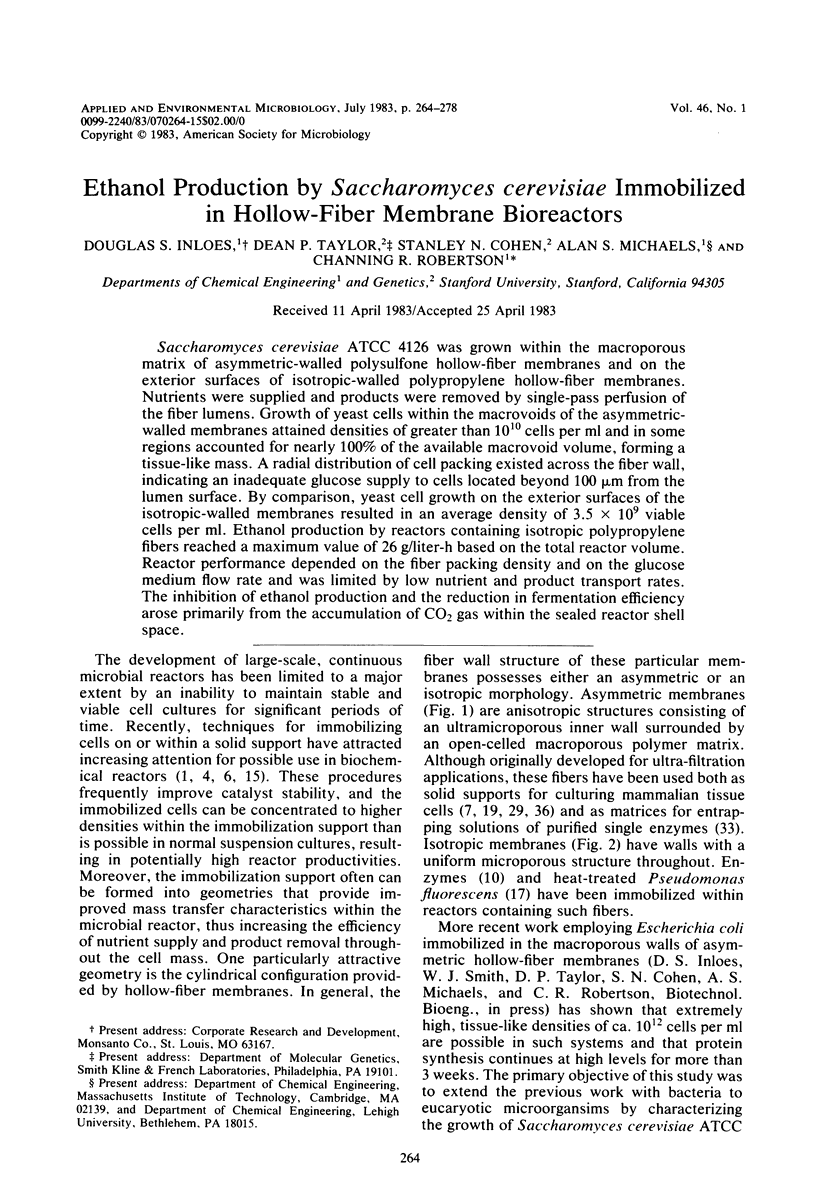
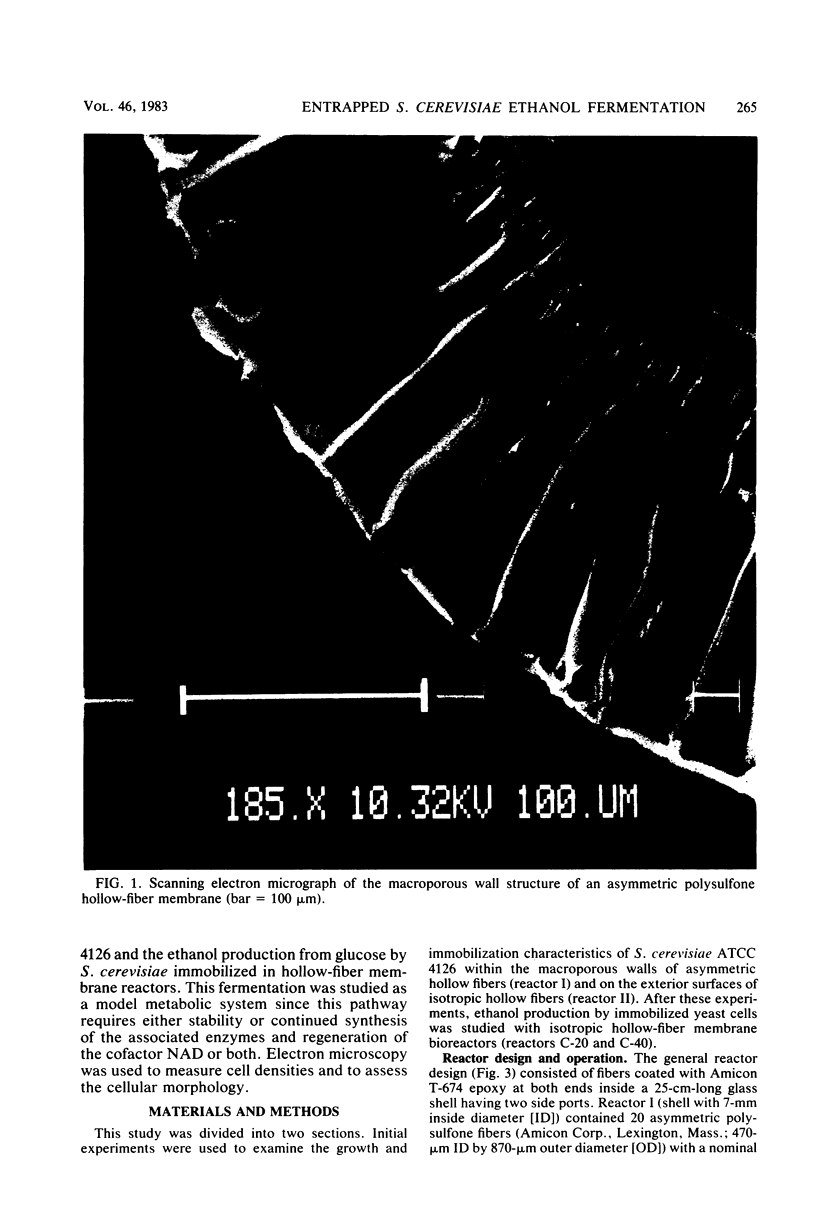
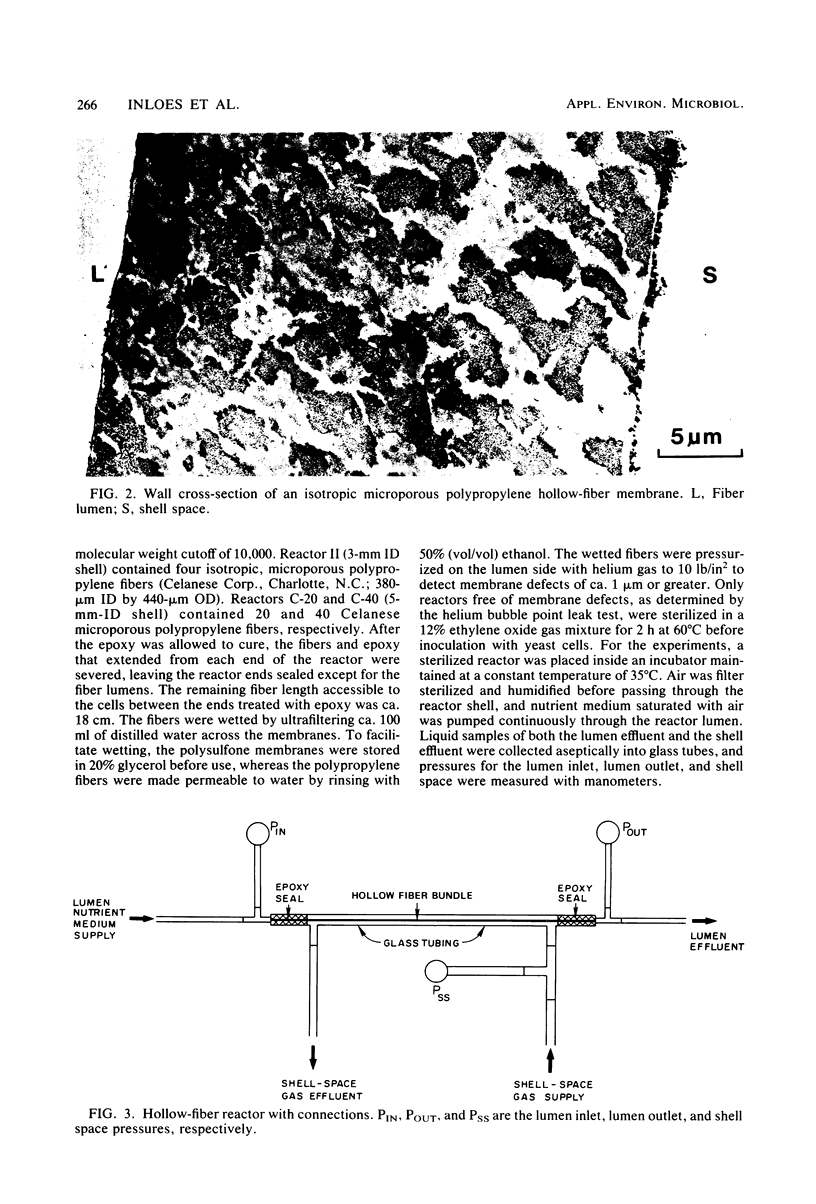
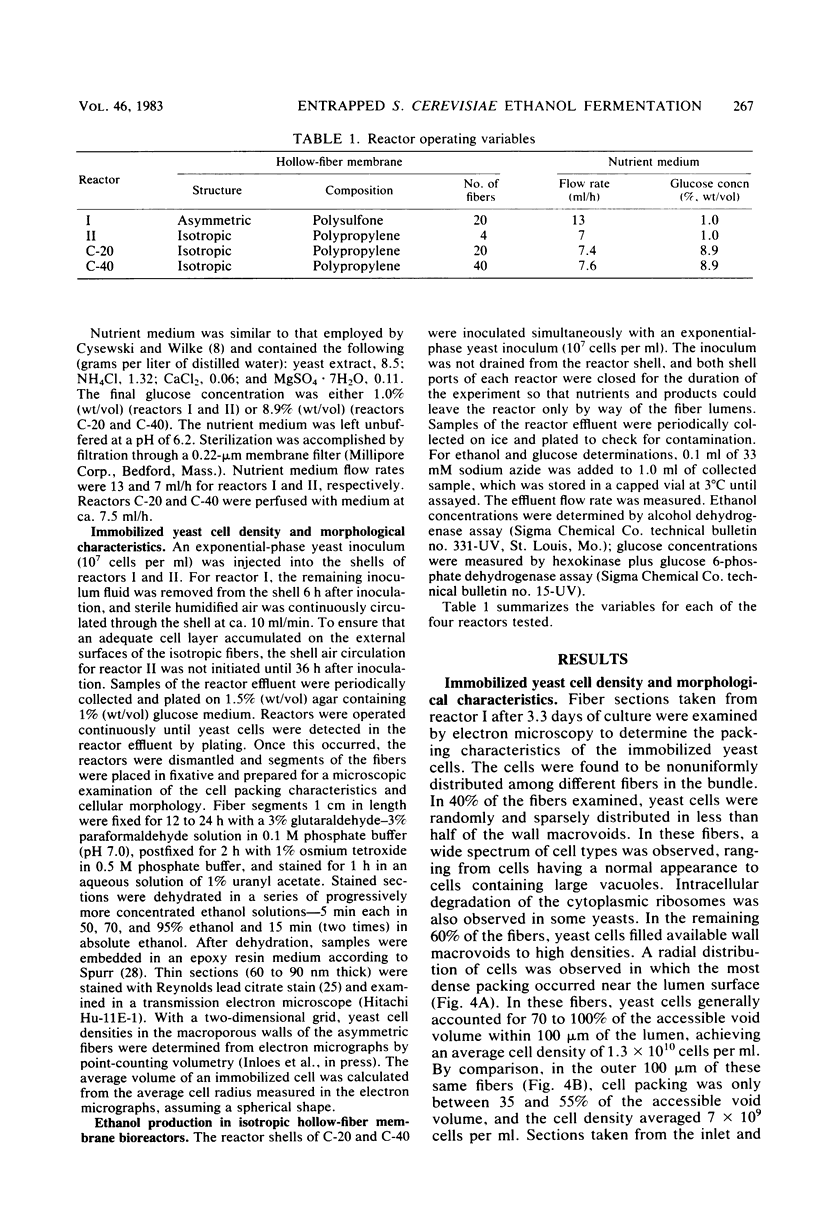
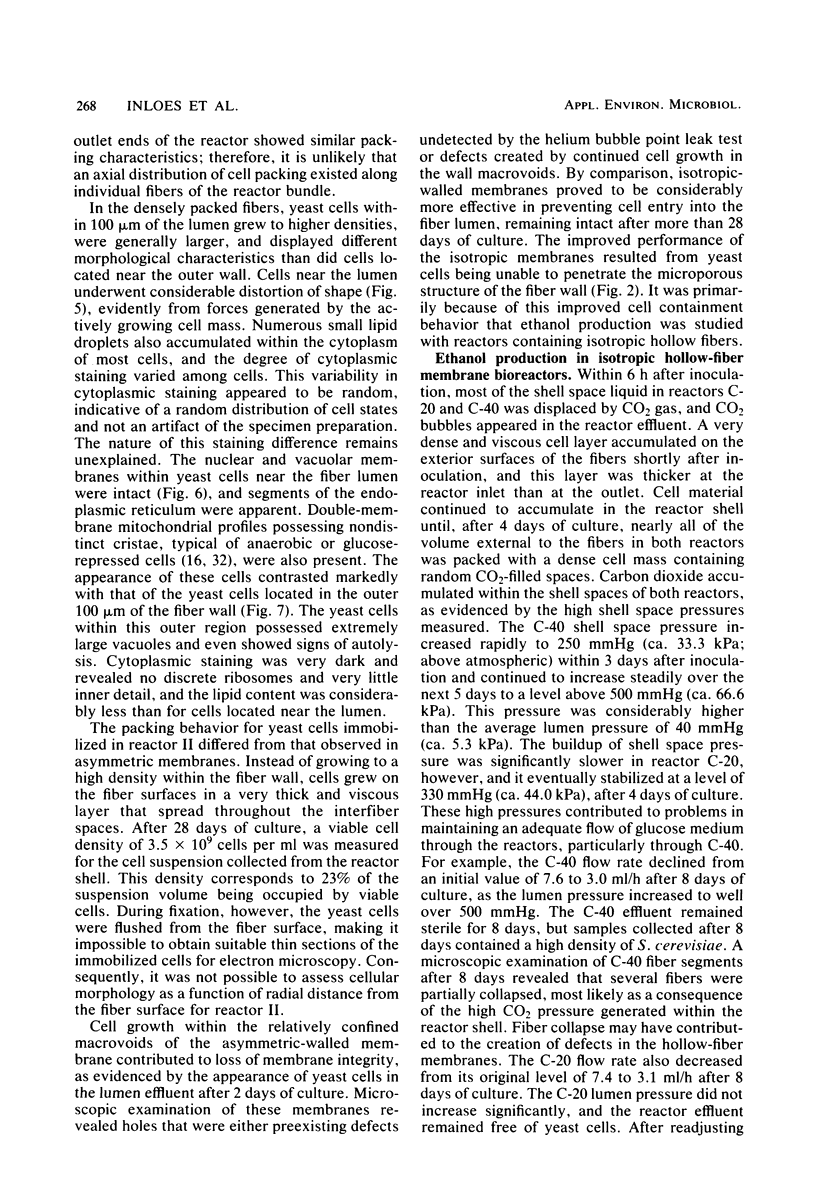
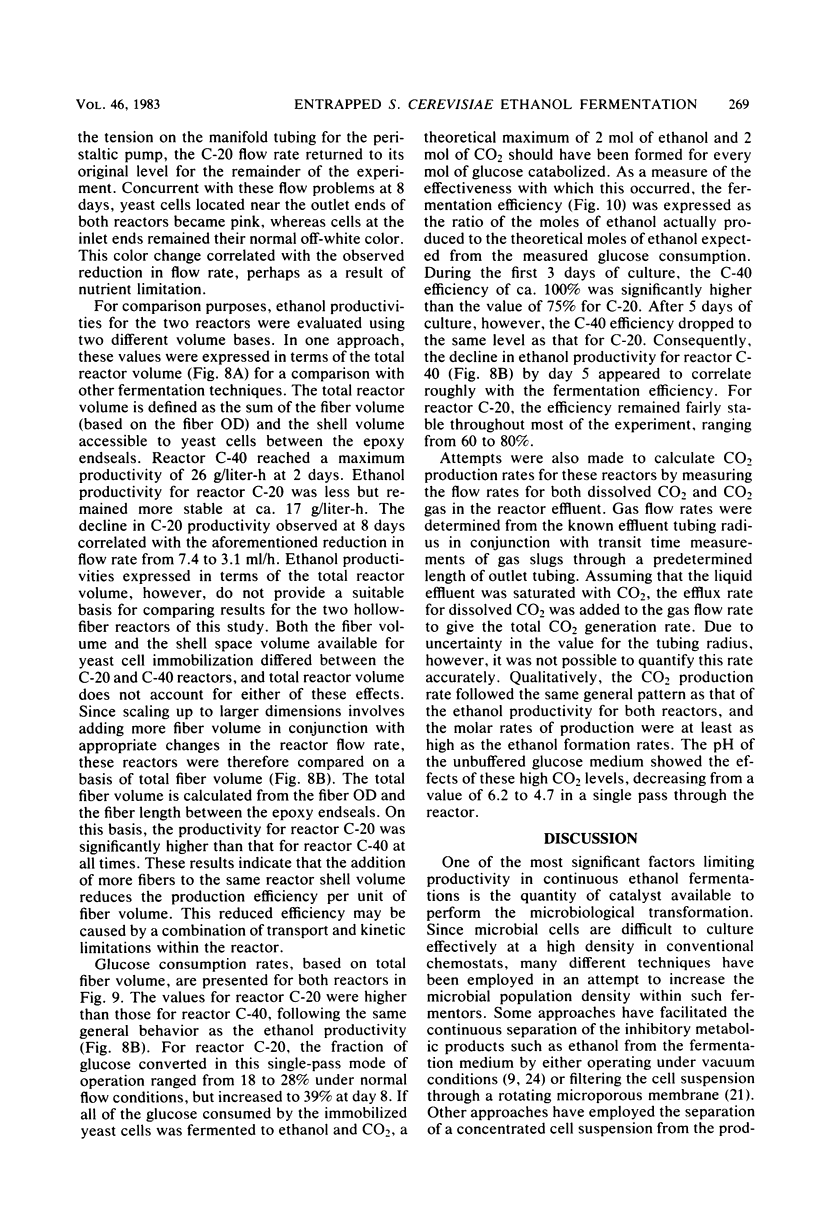
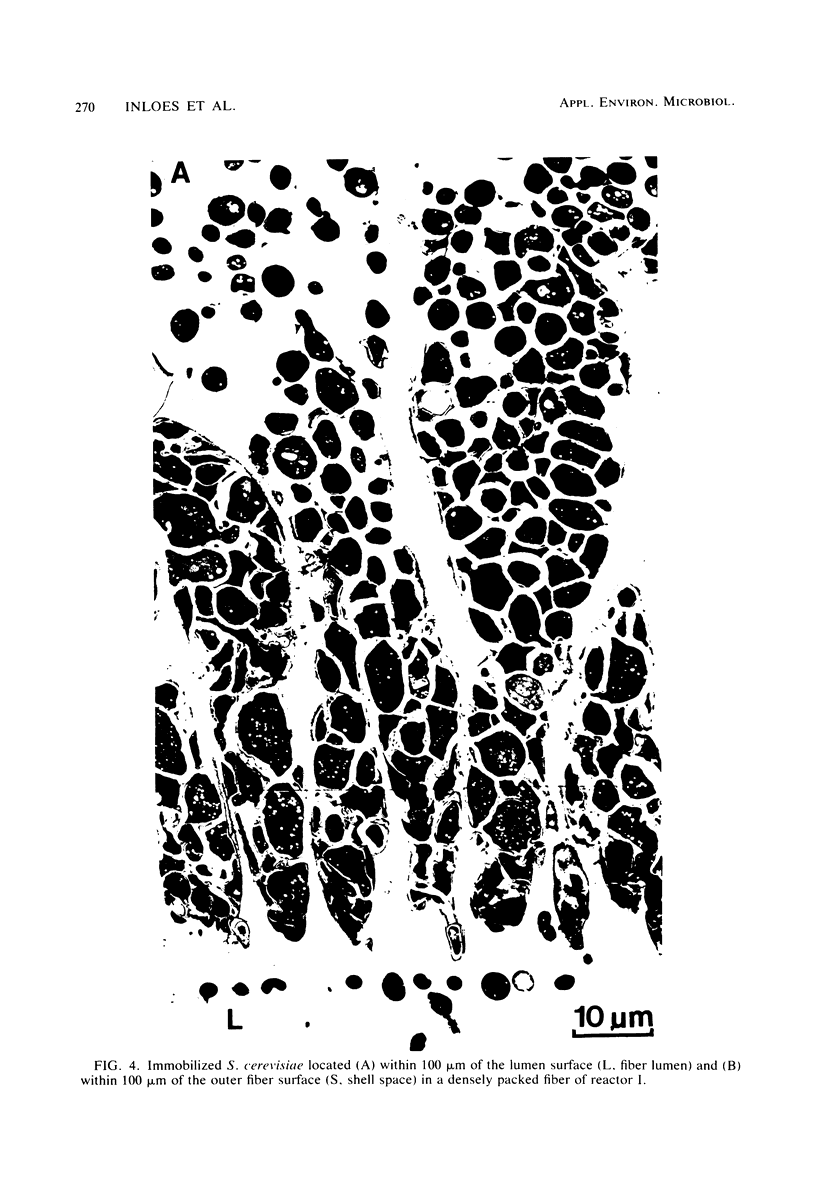
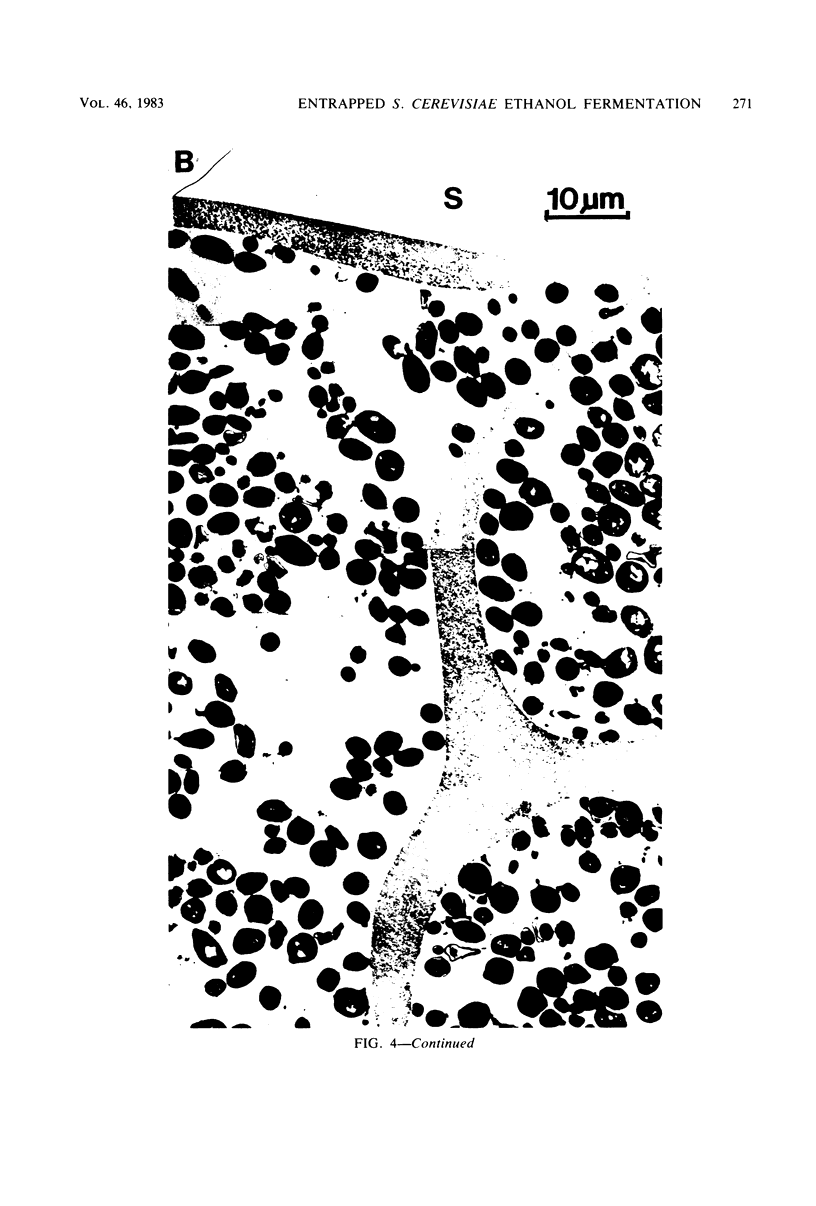
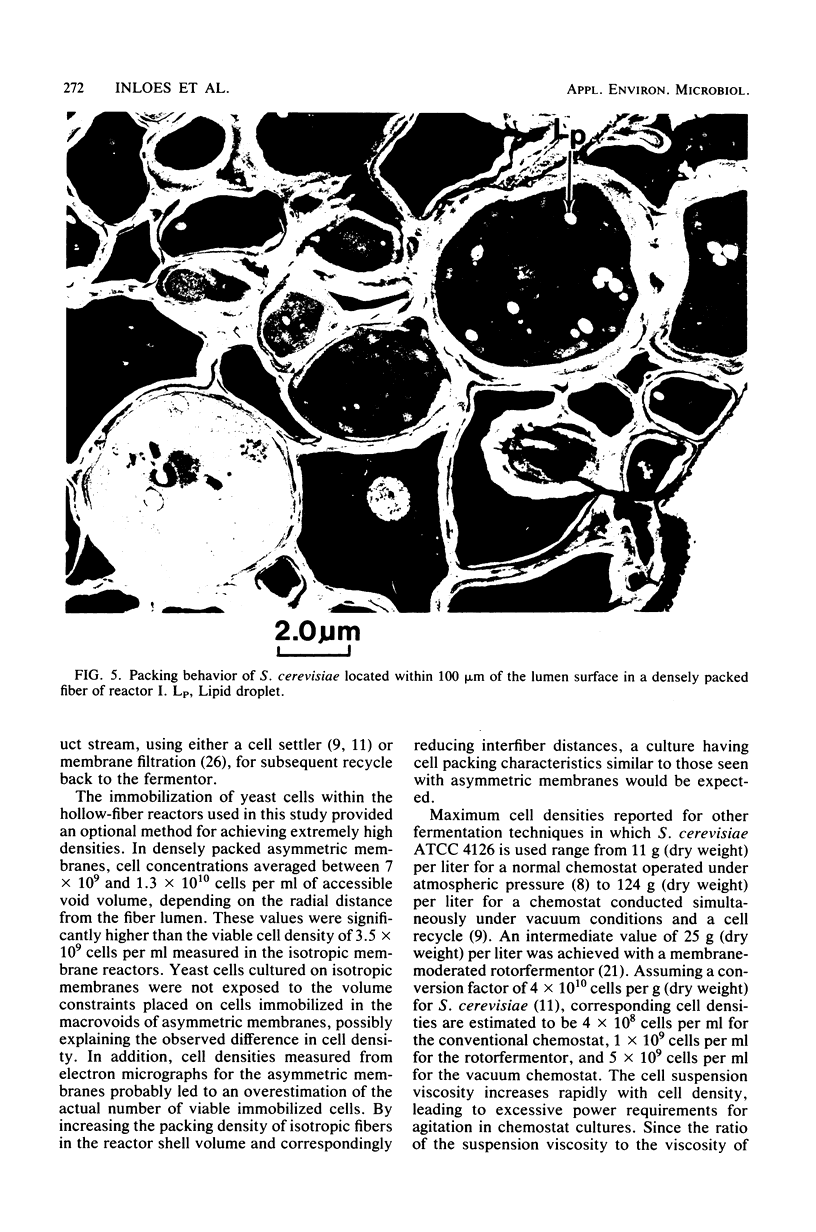
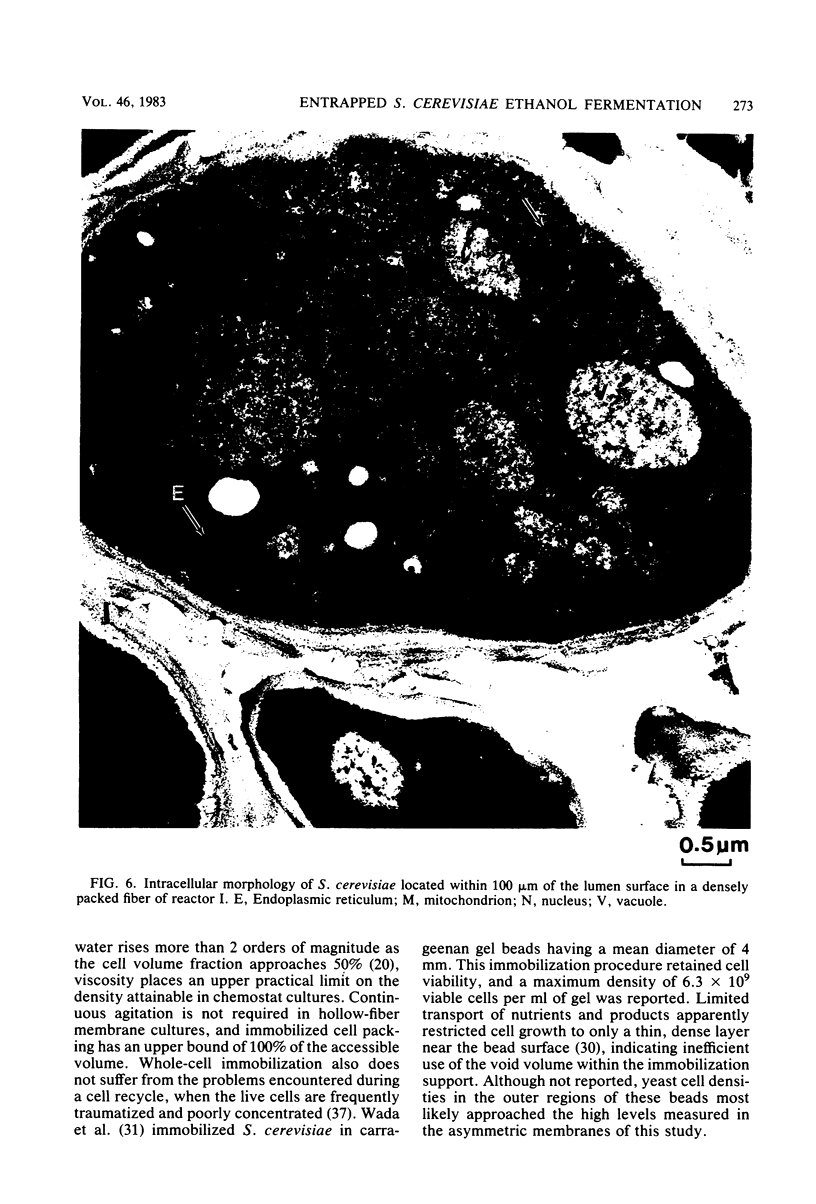
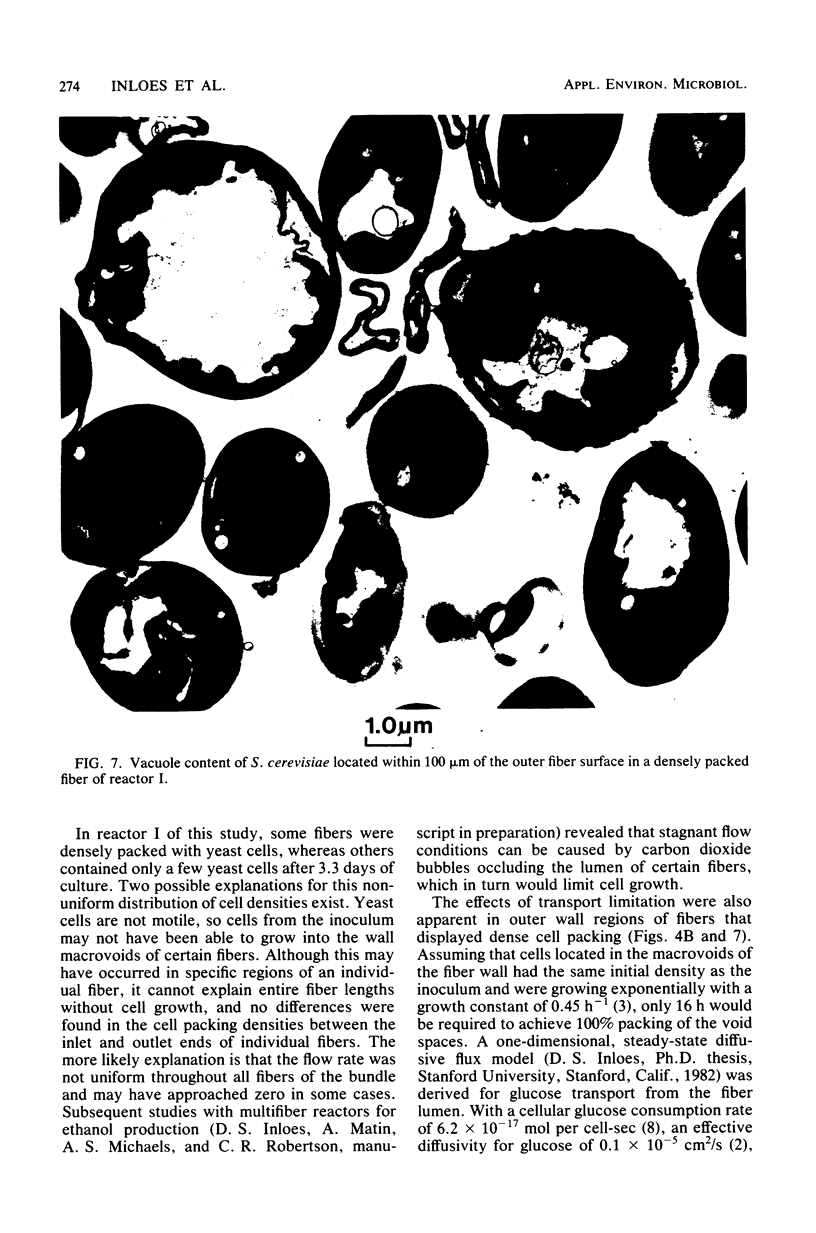
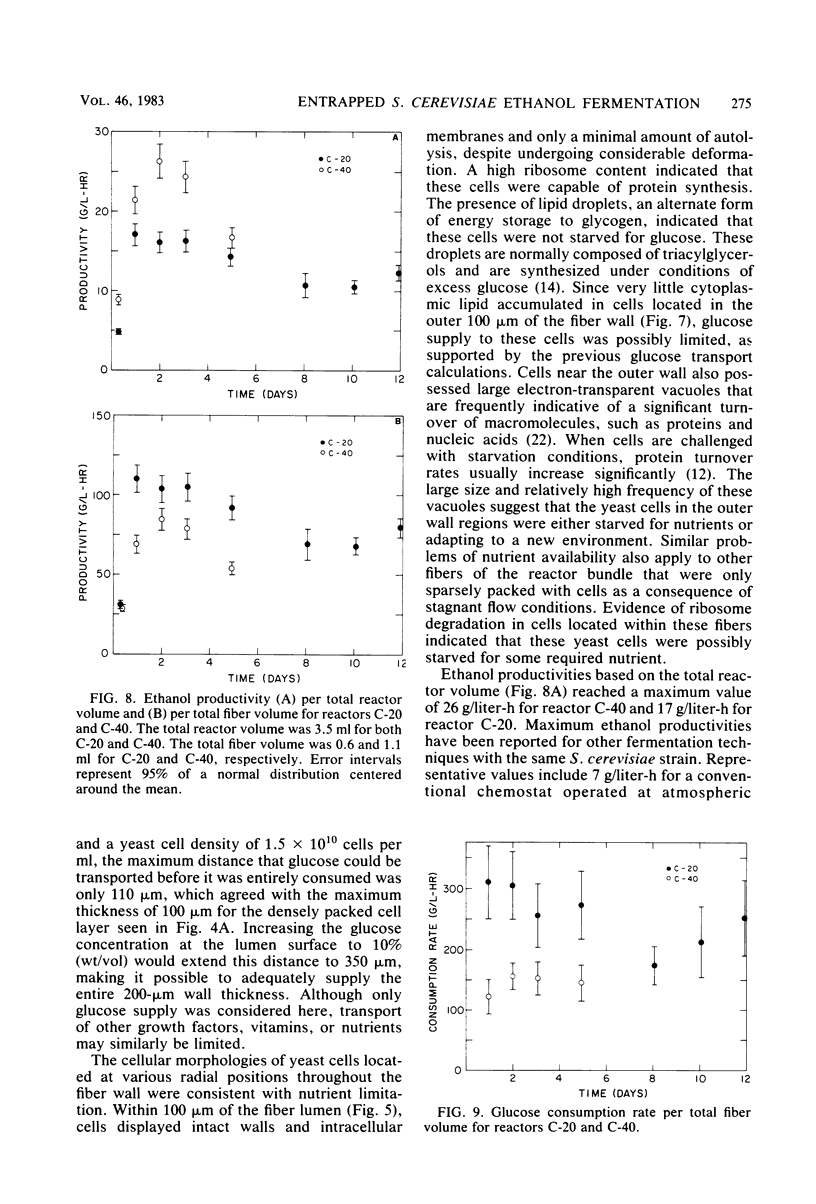
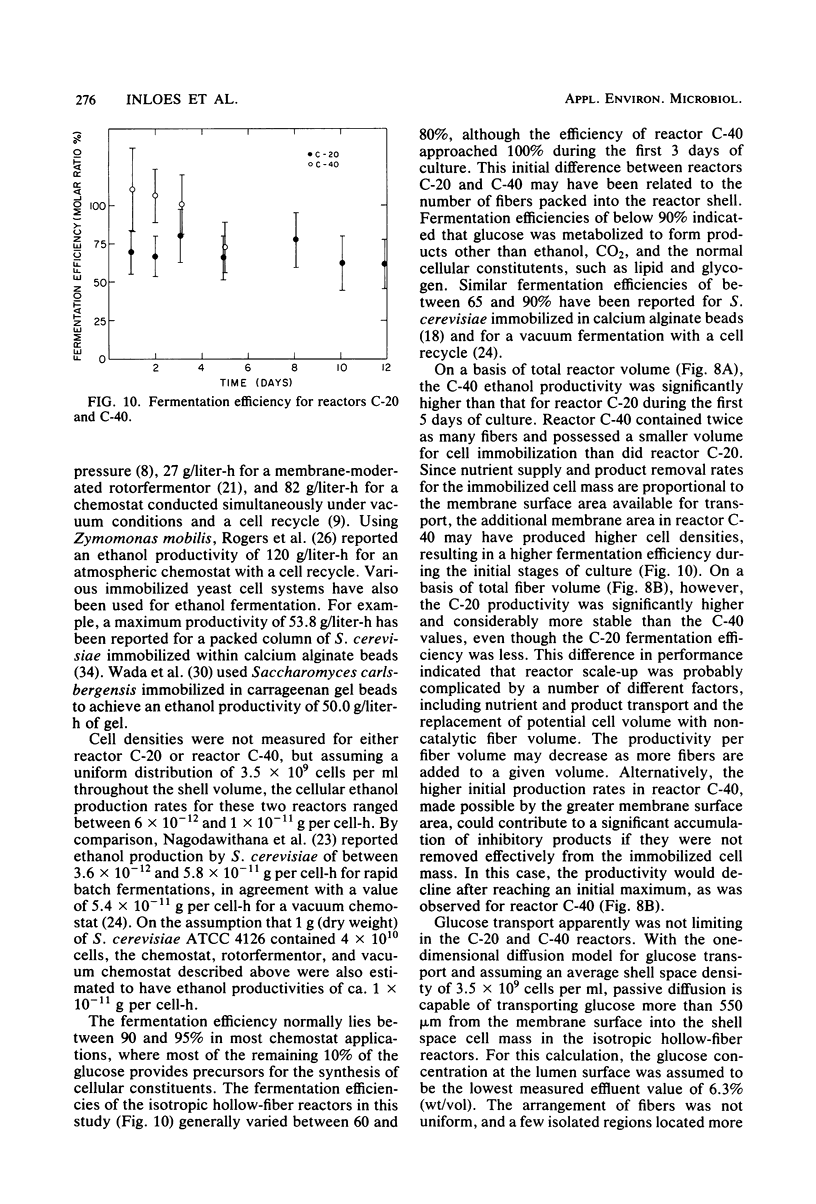
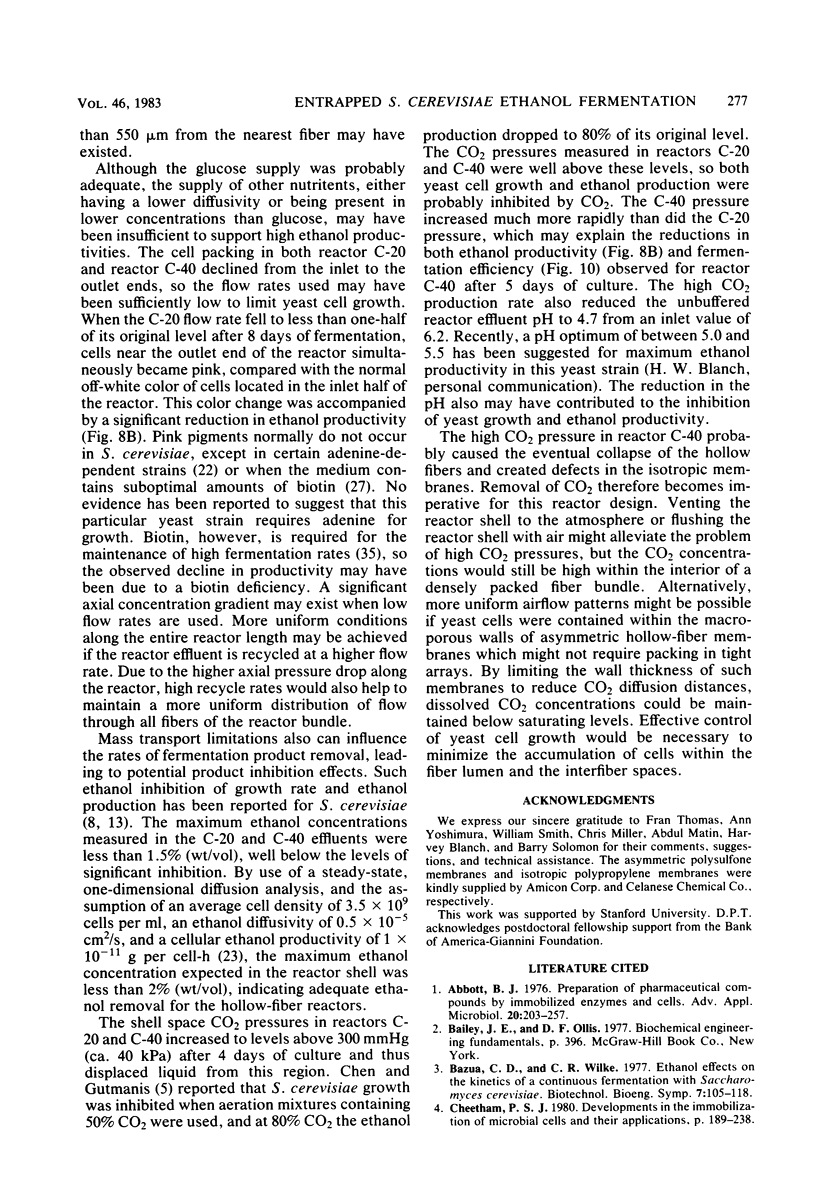
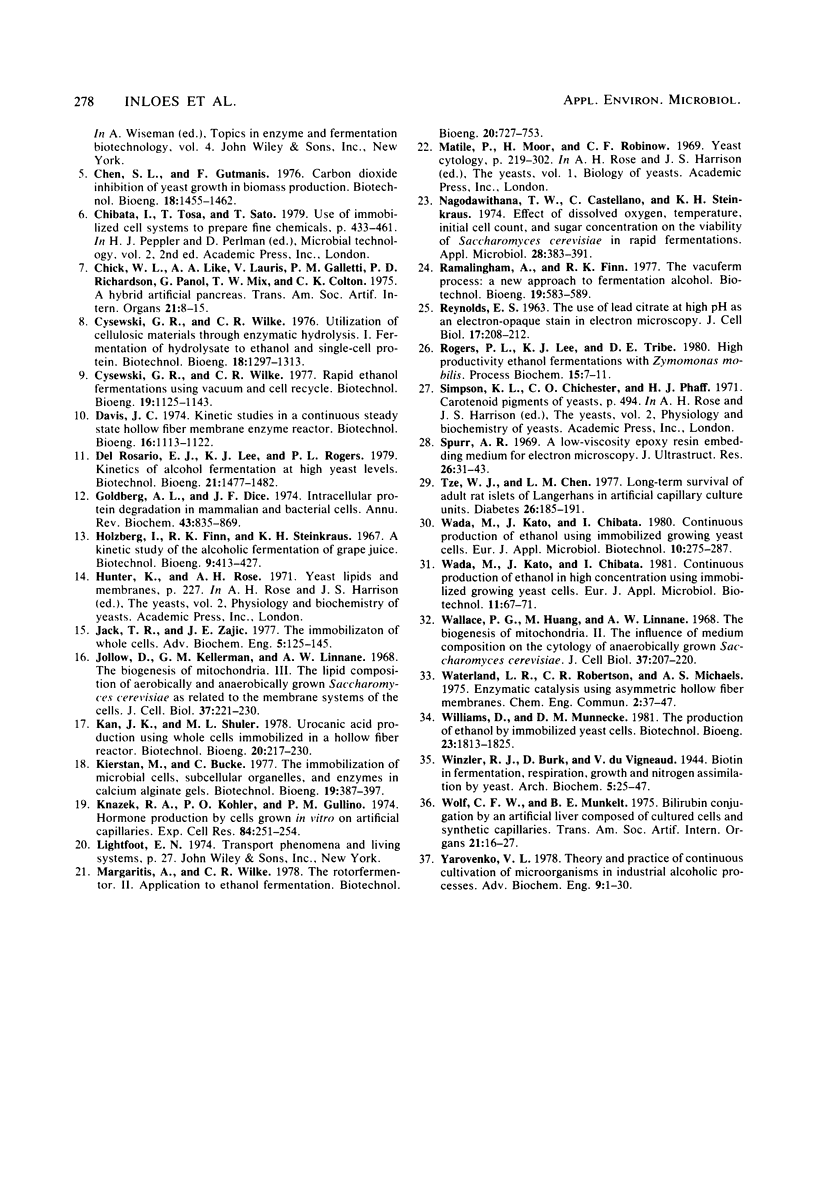
Images in this article
Selected References
These references are in PubMed. This may not be the complete list of references from this article.
- Abbott B. J. Preparation of pharmaceutical compounds by immobilized enzymes and cells. Adv Appl Microbiol. 1976;20:203–257. doi: 10.1016/s0065-2164(08)70113-8. [DOI] [PubMed] [Google Scholar]
- Bazua C. D., Wilke C. R. Ethanol effects on the kinetics of a continuous fermentation with Saccharomyces cerevisiae. Biotechnol Bioeng Symp. 1977;(7):105–118. [PubMed] [Google Scholar]
- Chen S. L., Gutmains F. Carbon dioxide inhibition of yeast growth in biomass production. Biotechnol Bioeng. 1976 Oct;18(10):1455–1462. doi: 10.1002/bit.260181012. [DOI] [PubMed] [Google Scholar]
- Chick W. L., Like A. A., Lauris V., Galletti P. M., Richardson P. D., Panol G., Mix T. W., Colton C. K. A hybird artifical pancreas. Trans Am Soc Artif Intern Organs. 1975;21:8–15. [PubMed] [Google Scholar]
- Cysewski G. R., Wilke C. R. Utilization of cellulosic materials through enzyamtic hydrolysis. I. Fermentation of hydrolysate to ethanol and single-cell protein. Biotechnol Bioeng. 1976 Sep;18(9):1297–1313. doi: 10.1002/bit.260180908. [DOI] [PubMed] [Google Scholar]
- Davis J. C. Kinetics studies in a continuous steady state hollow fiber membrane enzyme reactor. Biotechnol Bioeng. 1974 Aug;16(8):1113–1122. doi: 10.1002/bit.260160811. [DOI] [PubMed] [Google Scholar]
- Goldberg A. L., Dice J. F. Intracellular protein degradation in mammalian and bacterial cells. Annu Rev Biochem. 1974;43(0):835–869. doi: 10.1146/annurev.bi.43.070174.004155. [DOI] [PubMed] [Google Scholar]
- Jollow D., Kellerman G. M., Linnane A. W. The biogenesis of mitochondria. 3. The lipid composition of aerobically and anaerobically grown Saccharomyces cerevisiae as related to the membrane systems of the cells. J Cell Biol. 1968 May;37(2):221–230. doi: 10.1083/jcb.37.2.221. [DOI] [PMC free article] [PubMed] [Google Scholar]
- Kierstan M., Bucke C. The immobilization of microbial cells, subcellular organelles, and enzymes in calcium alginate gels. Biotechnol Bioeng. 1977 Mar;19(3):387–397. doi: 10.1002/bit.260190309. [DOI] [PubMed] [Google Scholar]
- Knazek R. A., Kohler P. O., Gullino P. M. Hormone production by cells grown in vitro on artificial capillaries. Exp Cell Res. 1974 Mar 15;84(1):251–254. doi: 10.1016/0014-4827(74)90403-0. [DOI] [PubMed] [Google Scholar]
- Margaritis A., Wilke C. R. The rotorfermentor. II. Application to ethanol fermentation. Biotechnol Bioeng. 1978 May;20(5):727–753. doi: 10.1002/bit.260200508. [DOI] [PubMed] [Google Scholar]
- Nagodawithana T. W., Castellano C., Steinkraus K. H. Effect of dissolved oxygen, temperature, initial cell count, and sugar concentration on the viability of Saccharomyces cerevisiae in rapid fermentations. Appl Microbiol. 1974 Sep;28(3):383–391. doi: 10.1128/am.28.3.383-391.1974. [DOI] [PMC free article] [PubMed] [Google Scholar]
- REYNOLDS E. S. The use of lead citrate at high pH as an electron-opaque stain in electron microscopy. J Cell Biol. 1963 Apr;17:208–212. doi: 10.1083/jcb.17.1.208. [DOI] [PMC free article] [PubMed] [Google Scholar]
- Spurr A. R. A low-viscosity epoxy resin embedding medium for electron microscopy. J Ultrastruct Res. 1969 Jan;26(1):31–43. doi: 10.1016/s0022-5320(69)90033-1. [DOI] [PubMed] [Google Scholar]
- Tze W. J., Chen L. M. Long-term survival of adult rat islets of langerhans in artificial capillary culture units. Diabetes. 1977 Mar;26(3):185–191. doi: 10.2337/diab.26.3.185. [DOI] [PubMed] [Google Scholar]
- Wallace P. G., Huang M., Linnane A. W. The biogenesis of mitochondria. II. The influence of medium composition on the cytology of anaerobically grown Saccharomyces cerevisiae. J Cell Biol. 1968 May;37(2):207–220. doi: 10.1083/jcb.37.2.207. [DOI] [PMC free article] [PubMed] [Google Scholar]
- Wolf C. F., Munkelt B. E. Bilirubin conjugation by an artificial liver composed of cultured cells and synthetic capillaries. Trans Am Soc Artif Intern Organs. 1975;21:16–27. [PubMed] [Google Scholar]