Abstract
The nucleotide sequence of the genome of equine arteritis virus (EAV) was determined from a set of overlapping cDNA clones and was found to contain eight open reading frames (ORFs). ORFs 2 through 7 are expressed from six 3'-coterminal subgenomic mRNAs, which are transcribed from the 3'-terminal quarter of the viral genome. A number of these ORFs are predicted to encode structural EAV proteins. The organization and expression of the 3' part of the EAV genome are remarkably similar to those of coronaviruses and toroviruses. The 5'-terminal three-quarters of the genome contain the putative EAV polymerase gene, which also shares a number of features with the corresponding gene of corona- and toroviruses. The gene contains two large ORFs, ORF1a and ORF1b, with an overlap region of 19 nucleotides. The presence of a "shifty" heptanucleotide sequence in this region and a downstream RNA pseudoknot structure indicate that ORF1b is probably expressed by ribosomal frameshifting. The frameshift-directing potential of the ORF1a/ORF1b overlap region was demonstrated by using a reporter gene. Moreover, the predicted ORF1b product was found to contain four domains which have been identified in the same relative positions in coronavirus and torovirus ORF1b products. The sequences of the EAV and coronavirus ORF1a proteins were found to be much more diverged. The EAV ORF1a product contains a putative trypsinlike serine protease motif. Our data indicate that EAV, presently considered a togavirus, is evolutionarily related to viruses from the coronaviruslike superfamily.
Full text
PDF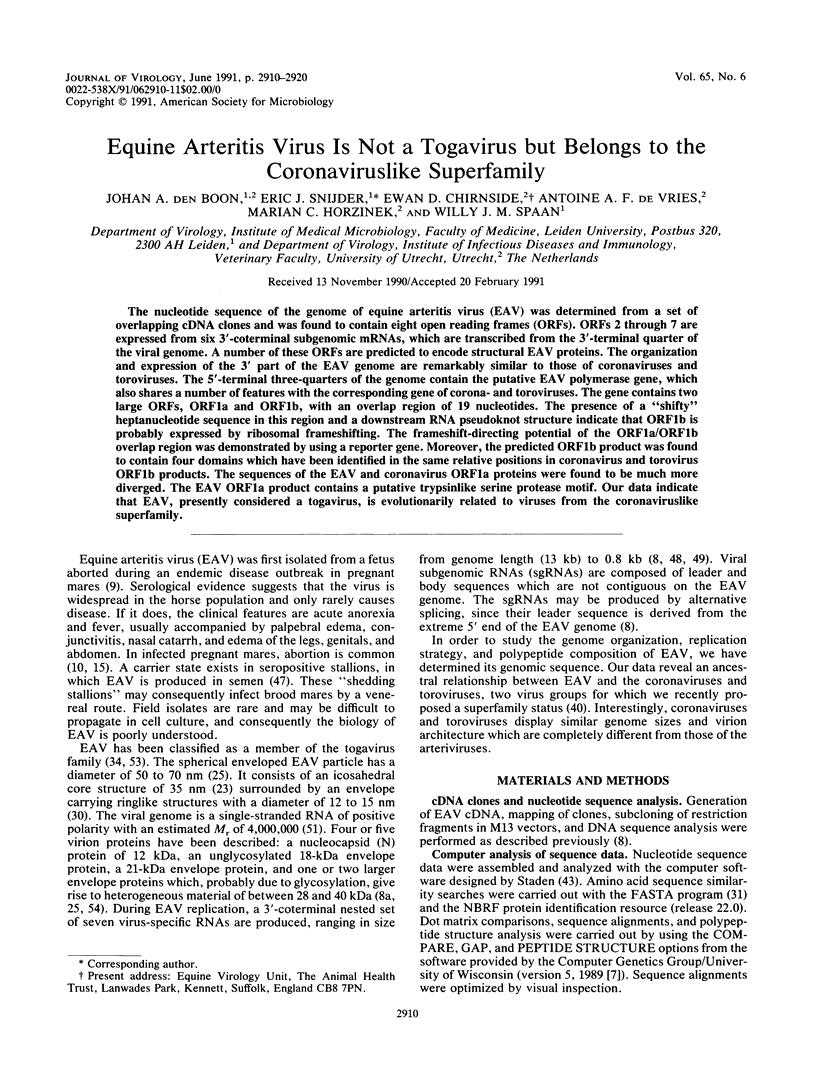
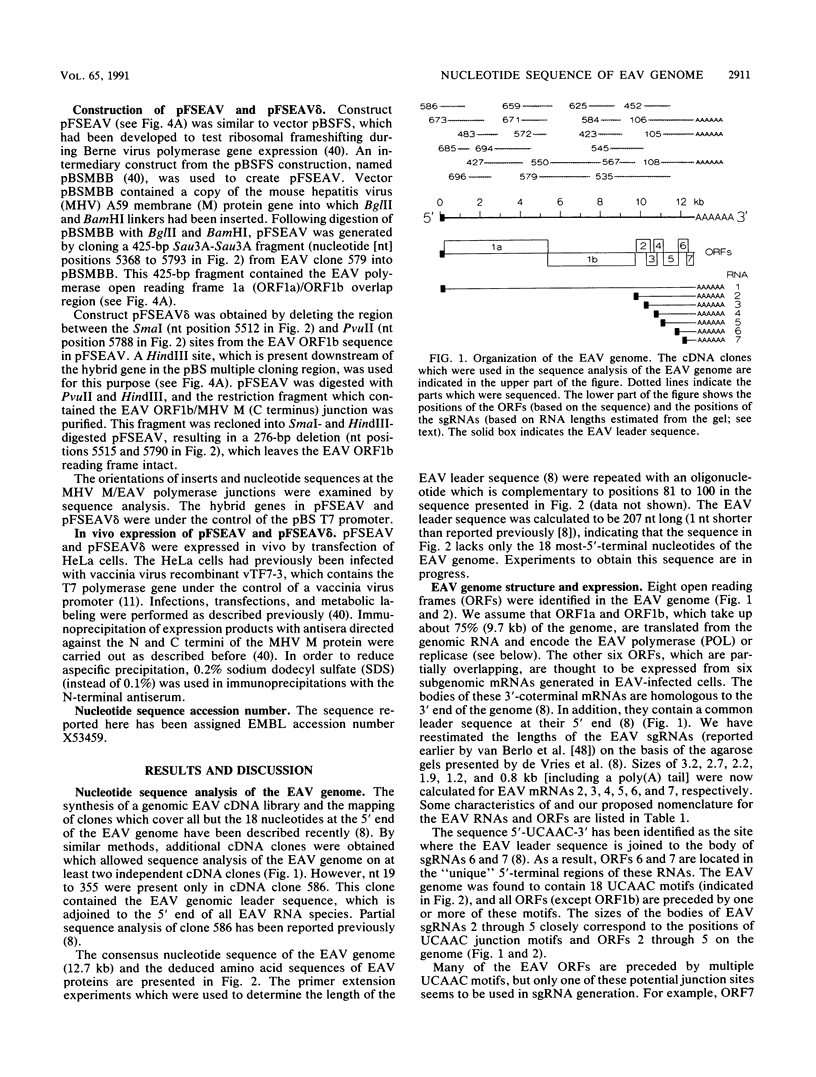
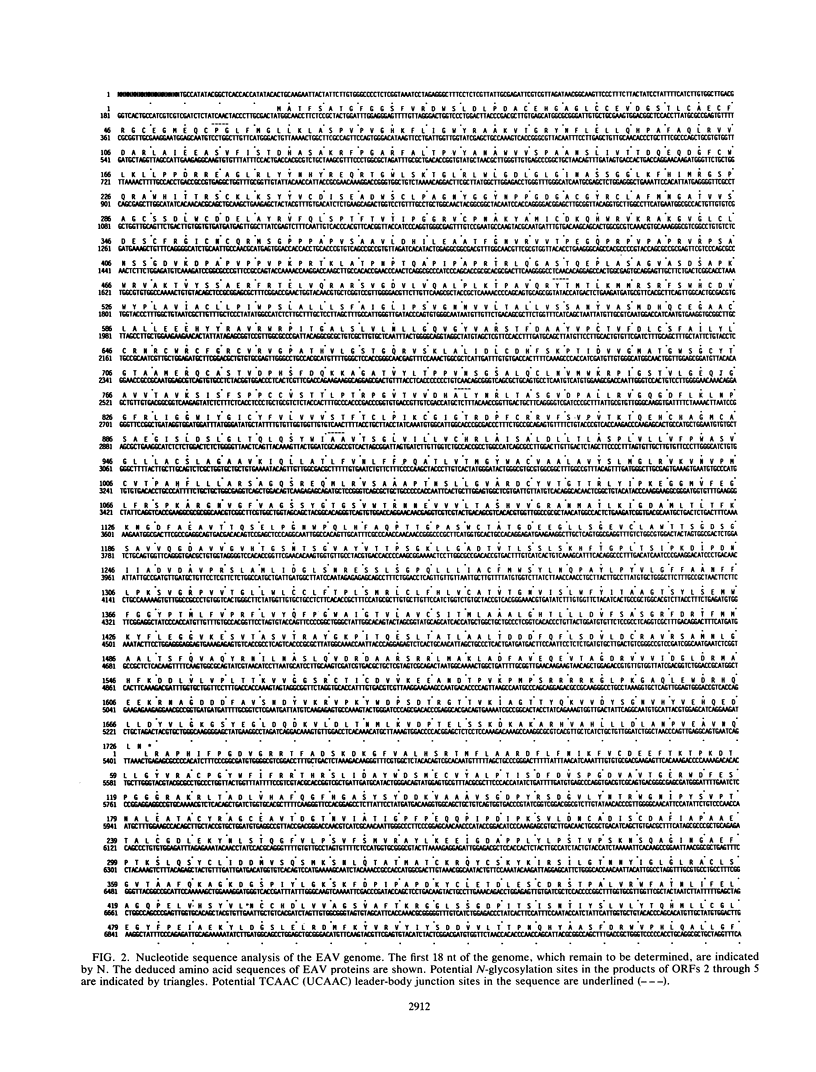
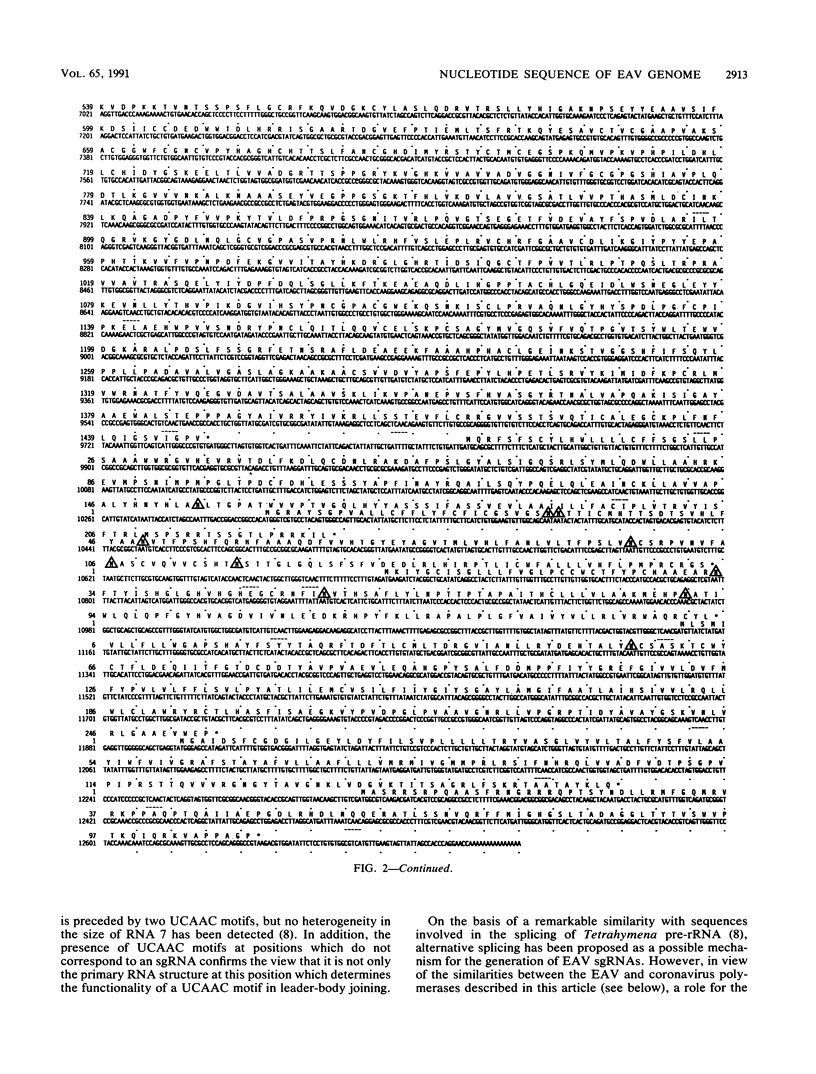
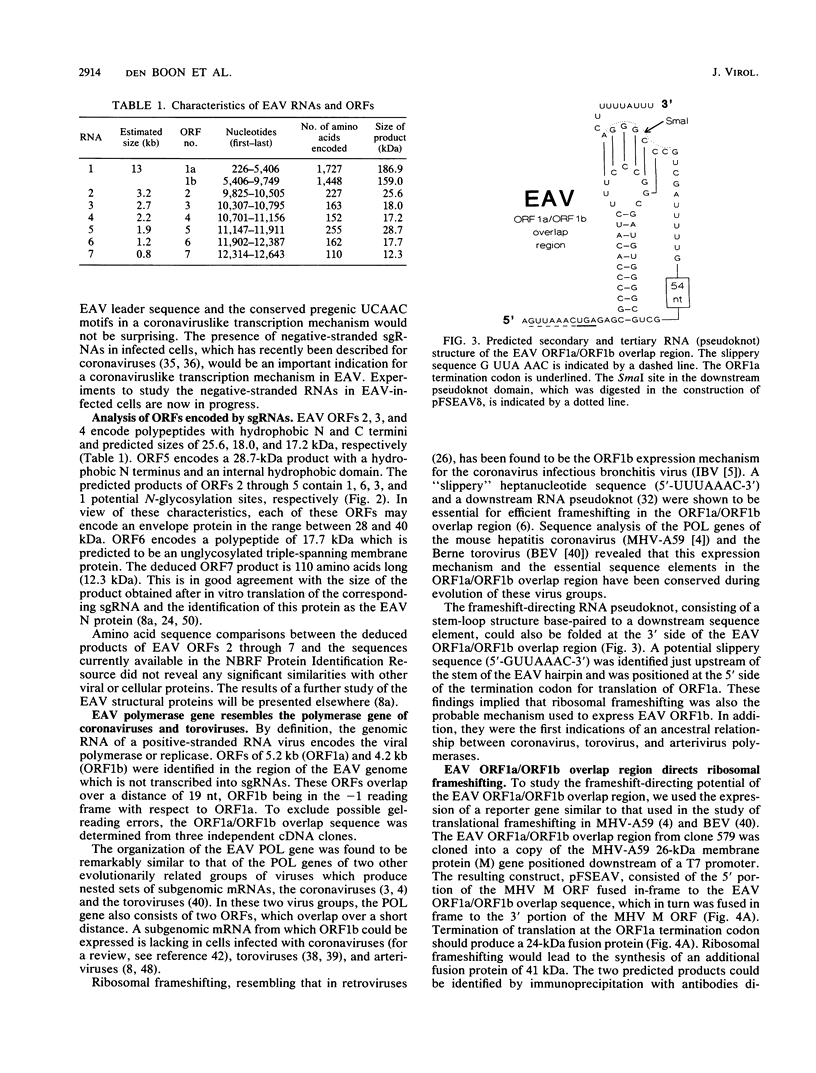
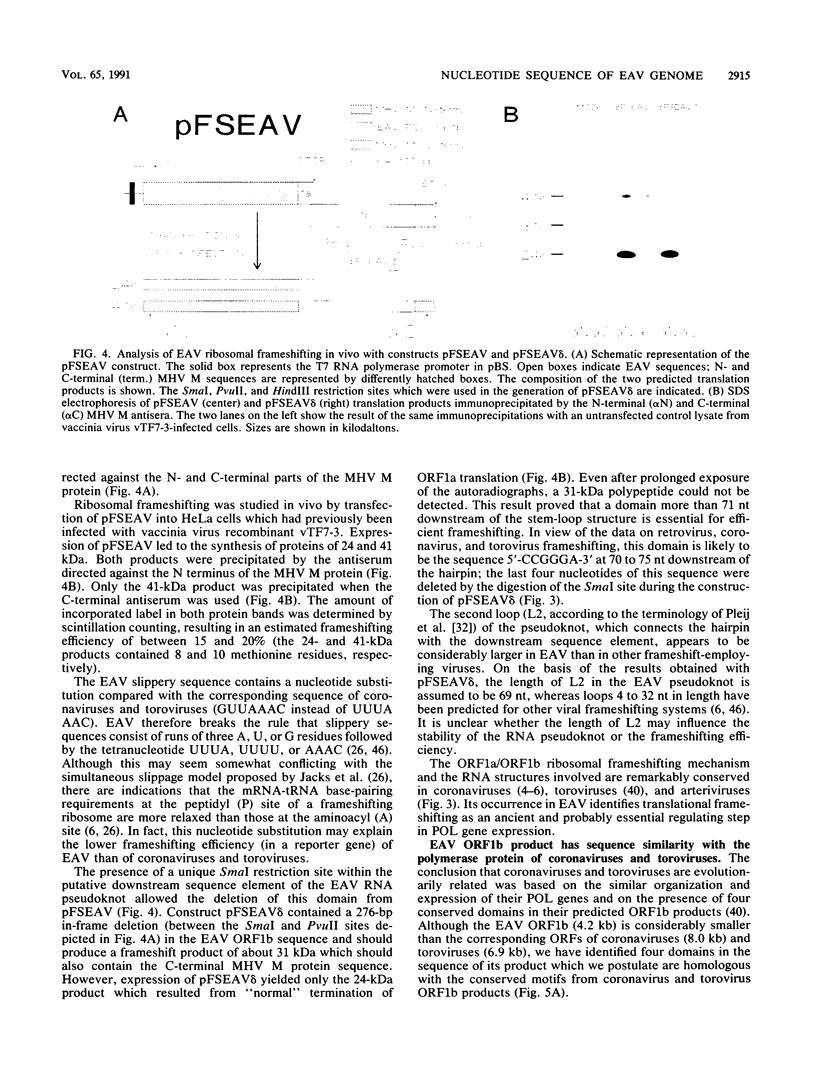
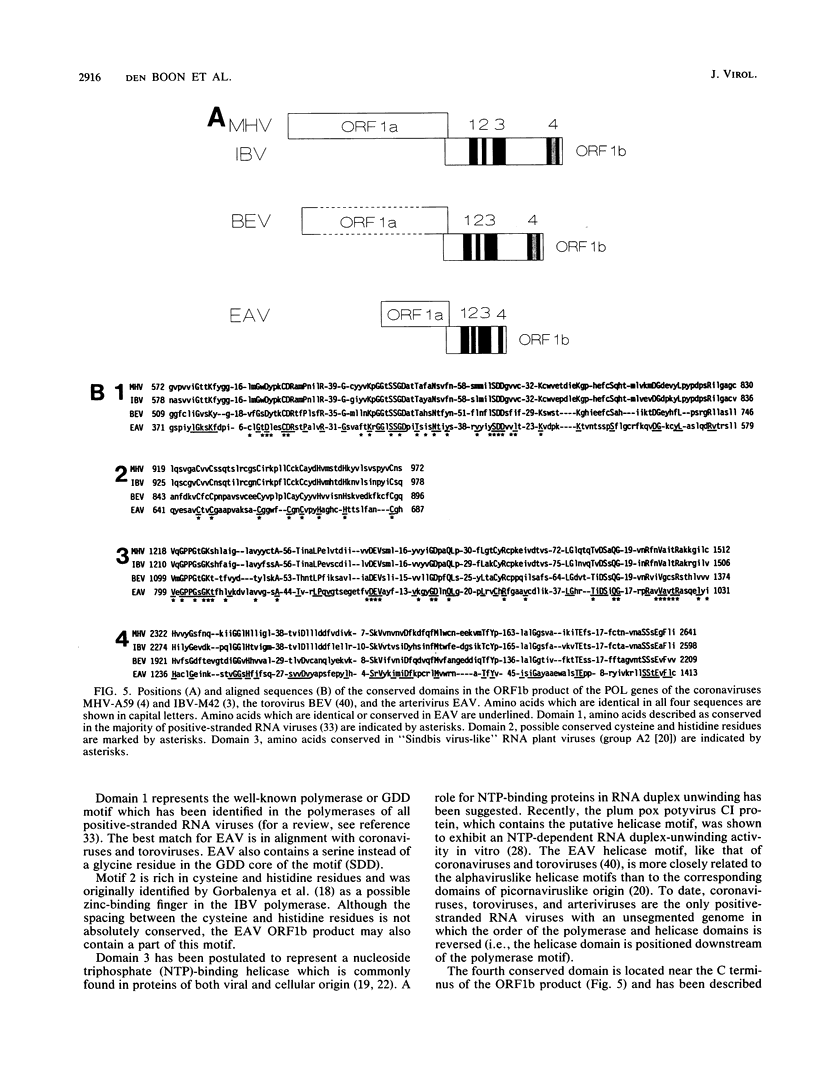
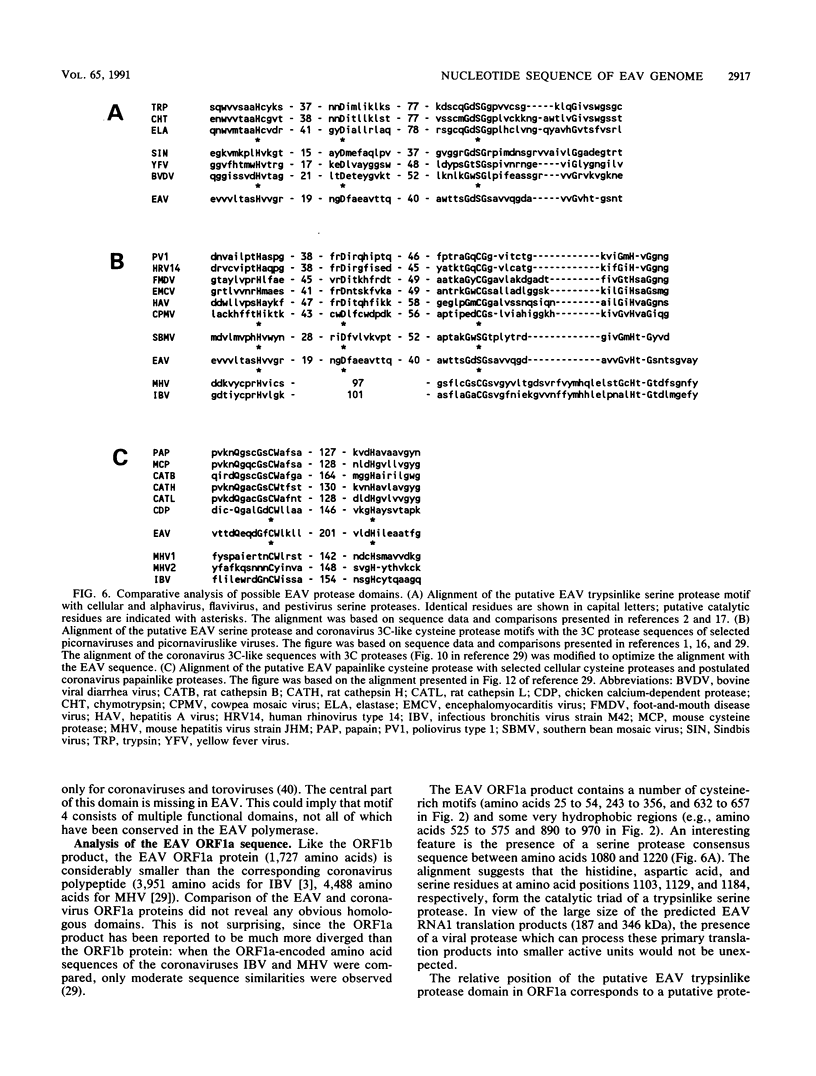
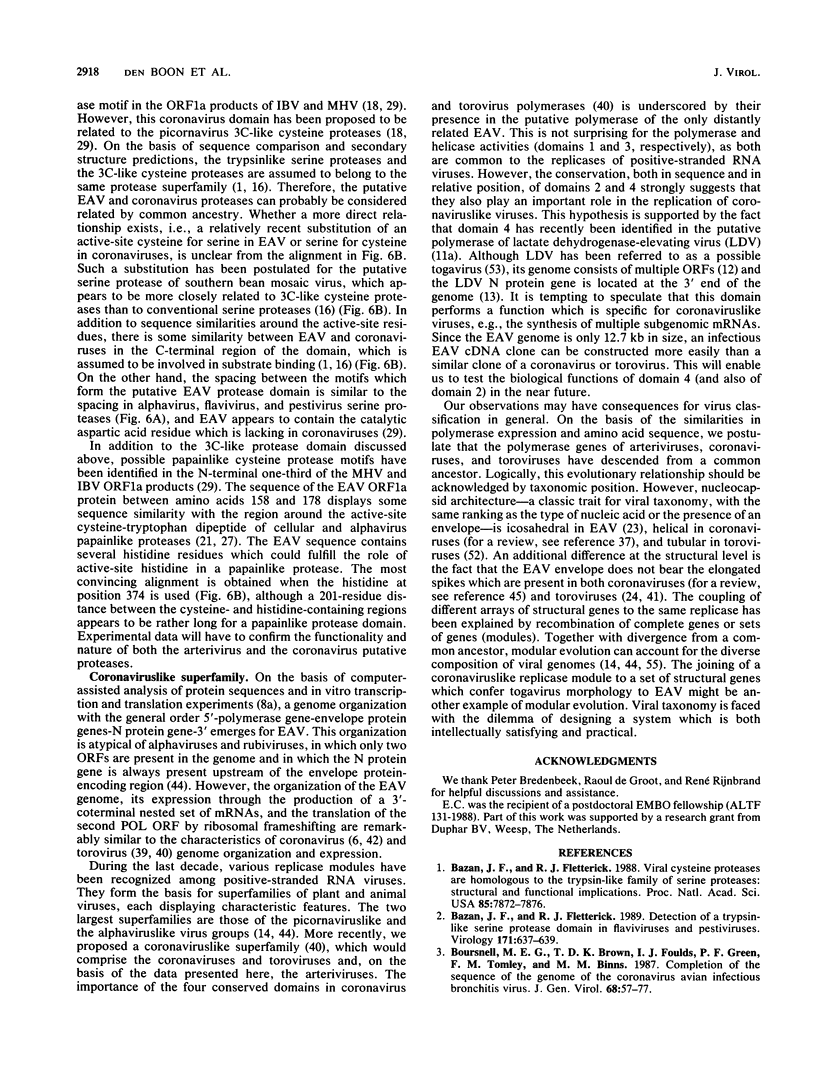
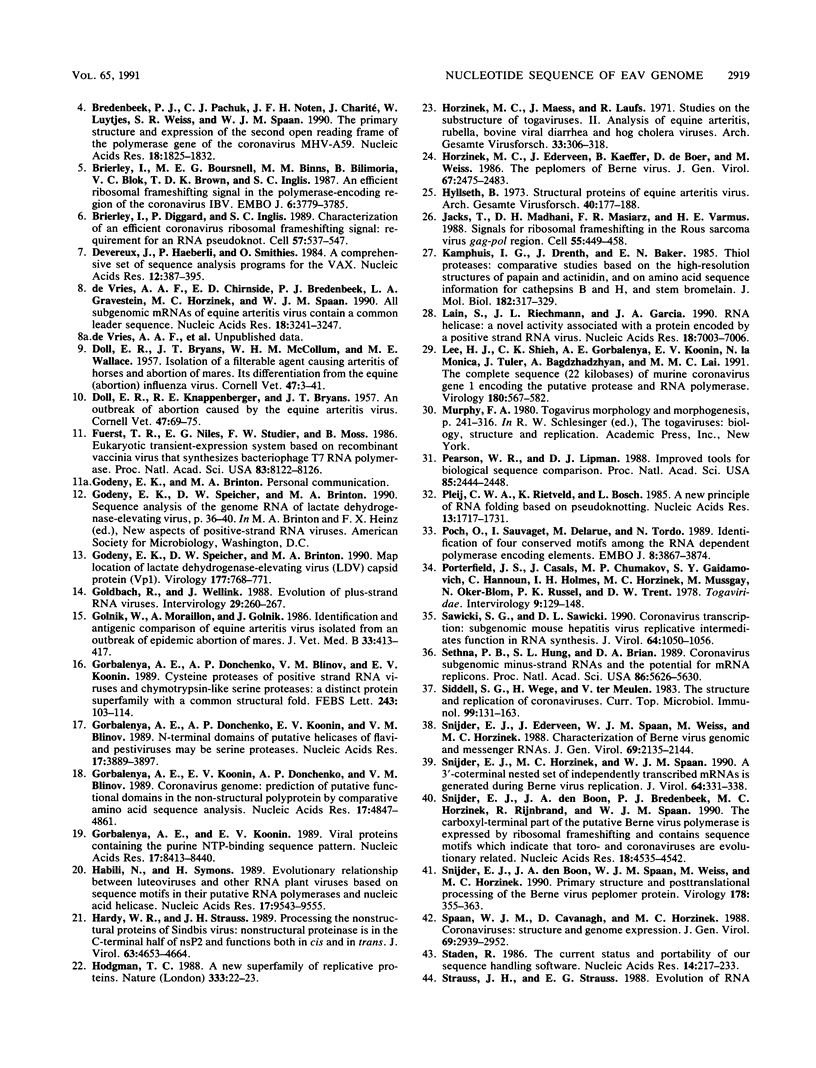
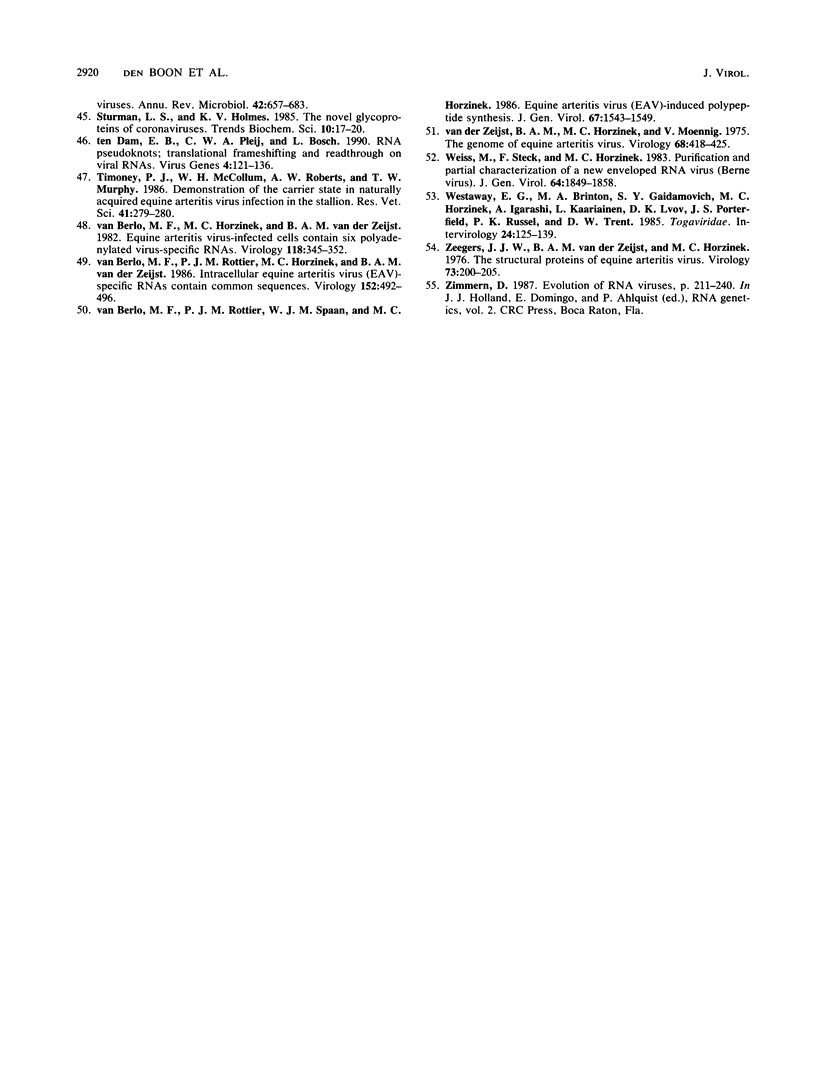
Images in this article
Selected References
These references are in PubMed. This may not be the complete list of references from this article.
- BRYANS J. T., CROWE M. E., DOLL E. R., MCCOLLUM W. H. Isolation of a filterable agent causing arteritis of horses and abortion by mares; its differentiation from the equine abortion (influenza) virus. Cornell Vet. 1957 Jan;47(1):3–41. [PubMed] [Google Scholar]
- BRYANS J. T., DOLL E. R., KNAPPENBERGER R. E. An outbreak of abortion caused by the equine arteritis virus. Cornell Vet. 1957 Jan;47(1):69–75. [PubMed] [Google Scholar]
- Bazan J. F., Fletterick R. J. Detection of a trypsin-like serine protease domain in flaviviruses and pestiviruses. Virology. 1989 Aug;171(2):637–639. doi: 10.1016/0042-6822(89)90639-9. [DOI] [PubMed] [Google Scholar]
- Bazan J. F., Fletterick R. J. Viral cysteine proteases are homologous to the trypsin-like family of serine proteases: structural and functional implications. Proc Natl Acad Sci U S A. 1988 Nov;85(21):7872–7876. doi: 10.1073/pnas.85.21.7872. [DOI] [PMC free article] [PubMed] [Google Scholar]
- Boursnell M. E., Brown T. D., Foulds I. J., Green P. F., Tomley F. M., Binns M. M. Completion of the sequence of the genome of the coronavirus avian infectious bronchitis virus. J Gen Virol. 1987 Jan;68(Pt 1):57–77. doi: 10.1099/0022-1317-68-1-57. [DOI] [PubMed] [Google Scholar]
- Bredenbeek P. J., Pachuk C. J., Noten A. F., Charité J., Luytjes W., Weiss S. R., Spaan W. J. The primary structure and expression of the second open reading frame of the polymerase gene of the coronavirus MHV-A59; a highly conserved polymerase is expressed by an efficient ribosomal frameshifting mechanism. Nucleic Acids Res. 1990 Apr 11;18(7):1825–1832. doi: 10.1093/nar/18.7.1825. [DOI] [PMC free article] [PubMed] [Google Scholar]
- Brierley I., Boursnell M. E., Binns M. M., Bilimoria B., Blok V. C., Brown T. D., Inglis S. C. An efficient ribosomal frame-shifting signal in the polymerase-encoding region of the coronavirus IBV. EMBO J. 1987 Dec 1;6(12):3779–3785. doi: 10.1002/j.1460-2075.1987.tb02713.x. [DOI] [PMC free article] [PubMed] [Google Scholar]
- Brierley I., Digard P., Inglis S. C. Characterization of an efficient coronavirus ribosomal frameshifting signal: requirement for an RNA pseudoknot. Cell. 1989 May 19;57(4):537–547. doi: 10.1016/0092-8674(89)90124-4. [DOI] [PMC free article] [PubMed] [Google Scholar]
- Devereux J., Haeberli P., Smithies O. A comprehensive set of sequence analysis programs for the VAX. Nucleic Acids Res. 1984 Jan 11;12(1 Pt 1):387–395. doi: 10.1093/nar/12.1part1.387. [DOI] [PMC free article] [PubMed] [Google Scholar]
- Fuerst T. R., Niles E. G., Studier F. W., Moss B. Eukaryotic transient-expression system based on recombinant vaccinia virus that synthesizes bacteriophage T7 RNA polymerase. Proc Natl Acad Sci U S A. 1986 Nov;83(21):8122–8126. doi: 10.1073/pnas.83.21.8122. [DOI] [PMC free article] [PubMed] [Google Scholar]
- Godeny E. K., Speicher D. W., Brinton M. A. Map location of lactate dehydrogenase-elevating virus (LDV) capsid protein (Vp1) gene. Virology. 1990 Aug;177(2):768–771. doi: 10.1016/0042-6822(90)90546-4. [DOI] [PMC free article] [PubMed] [Google Scholar]
- Goldbach R., Wellink J. Evolution of plus-strand RNA viruses. Intervirology. 1988;29(5):260–267. doi: 10.1159/000150054. [DOI] [PubMed] [Google Scholar]
- Golnik W., Moraillon A., Golnik J. Identification and antigenic comparison of equine arteritis virus isolated from an outbreak of epidemic abortion of mares. Zentralbl Veterinarmed B. 1986 Aug;33(6):413–417. doi: 10.1111/j.1439-0450.1986.tb00051.x. [DOI] [PubMed] [Google Scholar]
- Gorbalenya A. E., Donchenko A. P., Blinov V. M., Koonin E. V. Cysteine proteases of positive strand RNA viruses and chymotrypsin-like serine proteases. A distinct protein superfamily with a common structural fold. FEBS Lett. 1989 Jan 30;243(2):103–114. doi: 10.1016/0014-5793(89)80109-7. [DOI] [PubMed] [Google Scholar]
- Gorbalenya A. E., Donchenko A. P., Koonin E. V., Blinov V. M. N-terminal domains of putative helicases of flavi- and pestiviruses may be serine proteases. Nucleic Acids Res. 1989 May 25;17(10):3889–3897. doi: 10.1093/nar/17.10.3889. [DOI] [PMC free article] [PubMed] [Google Scholar]
- Gorbalenya A. E., Koonin E. V., Donchenko A. P., Blinov V. M. Coronavirus genome: prediction of putative functional domains in the non-structural polyprotein by comparative amino acid sequence analysis. Nucleic Acids Res. 1989 Jun 26;17(12):4847–4861. doi: 10.1093/nar/17.12.4847. [DOI] [PMC free article] [PubMed] [Google Scholar]
- Gorbalenya A. E., Koonin E. V. Viral proteins containing the purine NTP-binding sequence pattern. Nucleic Acids Res. 1989 Nov 11;17(21):8413–8440. doi: 10.1093/nar/17.21.8413. [DOI] [PMC free article] [PubMed] [Google Scholar]
- Habili N., Symons R. H. Evolutionary relationship between luteoviruses and other RNA plant viruses based on sequence motifs in their putative RNA polymerases and nucleic acid helicases. Nucleic Acids Res. 1989 Dec 11;17(23):9543–9555. doi: 10.1093/nar/17.23.9543. [DOI] [PMC free article] [PubMed] [Google Scholar]
- Hardy W. R., Strauss J. H. Processing the nonstructural polyproteins of sindbis virus: nonstructural proteinase is in the C-terminal half of nsP2 and functions both in cis and in trans. J Virol. 1989 Nov;63(11):4653–4664. doi: 10.1128/jvi.63.11.4653-4664.1989. [DOI] [PMC free article] [PubMed] [Google Scholar]
- Hodgman T. C. A new superfamily of replicative proteins. Nature. 1988 May 5;333(6168):22–23. doi: 10.1038/333022b0. [DOI] [PubMed] [Google Scholar]
- Horzinek M. C., Ederveen J., Kaeffer B., de Boer D., Weiss M. The peplomers of Berne virus. J Gen Virol. 1986 Nov;67(Pt 11):2475–2483. doi: 10.1099/0022-1317-67-11-2475. [DOI] [PubMed] [Google Scholar]
- Horzinek M., Maess J., Laufs R. Studies on the substructure of togaviruses. II. Analysis of equine arteritis, rubella, bovine viral diarrhea, and hog cholera viruses. Arch Gesamte Virusforsch. 1971;33(3):306–318. [PubMed] [Google Scholar]
- Hyllseth B. Structural proteins of equine arteritis virus. Arch Gesamte Virusforsch. 1973;40(3):177–188. doi: 10.1007/BF01242536. [DOI] [PubMed] [Google Scholar]
- Jacks T., Madhani H. D., Masiarz F. R., Varmus H. E. Signals for ribosomal frameshifting in the Rous sarcoma virus gag-pol region. Cell. 1988 Nov 4;55(3):447–458. doi: 10.1016/0092-8674(88)90031-1. [DOI] [PMC free article] [PubMed] [Google Scholar]
- Kamphuis I. G., Drenth J., Baker E. N. Thiol proteases. Comparative studies based on the high-resolution structures of papain and actinidin, and on amino acid sequence information for cathepsins B and H, and stem bromelain. J Mol Biol. 1985 Mar 20;182(2):317–329. doi: 10.1016/0022-2836(85)90348-1. [DOI] [PubMed] [Google Scholar]
- Laín S., Riechmann J. L., García J. A. RNA helicase: a novel activity associated with a protein encoded by a positive strand RNA virus. Nucleic Acids Res. 1990 Dec 11;18(23):7003–7006. doi: 10.1093/nar/18.23.7003. [DOI] [PMC free article] [PubMed] [Google Scholar]
- Lee H. J., Shieh C. K., Gorbalenya A. E., Koonin E. V., La Monica N., Tuler J., Bagdzhadzhyan A., Lai M. M. The complete sequence (22 kilobases) of murine coronavirus gene 1 encoding the putative proteases and RNA polymerase. Virology. 1991 Feb;180(2):567–582. doi: 10.1016/0042-6822(91)90071-I. [DOI] [PMC free article] [PubMed] [Google Scholar]
- Pearson W. R., Lipman D. J. Improved tools for biological sequence comparison. Proc Natl Acad Sci U S A. 1988 Apr;85(8):2444–2448. doi: 10.1073/pnas.85.8.2444. [DOI] [PMC free article] [PubMed] [Google Scholar]
- Pleij C. W., Rietveld K., Bosch L. A new principle of RNA folding based on pseudoknotting. Nucleic Acids Res. 1985 Mar 11;13(5):1717–1731. doi: 10.1093/nar/13.5.1717. [DOI] [PMC free article] [PubMed] [Google Scholar]
- Poch O., Sauvaget I., Delarue M., Tordo N. Identification of four conserved motifs among the RNA-dependent polymerase encoding elements. EMBO J. 1989 Dec 1;8(12):3867–3874. doi: 10.1002/j.1460-2075.1989.tb08565.x. [DOI] [PMC free article] [PubMed] [Google Scholar]
- Porterfield J. S., Casals J., Chumakov M. P., Gaidamovich S. Y., Hannoun C., Holmes I. H., Horzinek M. C., Mussgay M., Oker-Blom N., Russell P. K. Togaviridae. Intervirology. 1978;9(3):129–148. doi: 10.1159/000148930. [DOI] [PubMed] [Google Scholar]
- Sawicki S. G., Sawicki D. L. Coronavirus transcription: subgenomic mouse hepatitis virus replicative intermediates function in RNA synthesis. J Virol. 1990 Mar;64(3):1050–1056. doi: 10.1128/jvi.64.3.1050-1056.1990. [DOI] [PMC free article] [PubMed] [Google Scholar]
- Sethna P. B., Hung S. L., Brian D. A. Coronavirus subgenomic minus-strand RNAs and the potential for mRNA replicons. Proc Natl Acad Sci U S A. 1989 Jul;86(14):5626–5630. doi: 10.1073/pnas.86.14.5626. [DOI] [PMC free article] [PubMed] [Google Scholar]
- Siddell S., Wege H., ter Meulen V. The structure and replication of coronaviruses. Curr Top Microbiol Immunol. 1982;99:131–163. doi: 10.1007/978-3-642-68528-6_4. [DOI] [PubMed] [Google Scholar]
- Snijder E. J., Den Boon J. A., Spaan W. J., Weiss M., Horzinek M. C. Primary structure and post-translational processing of the Berne virus peplomer protein. Virology. 1990 Oct;178(2):355–363. doi: 10.1016/0042-6822(90)90332-L. [DOI] [PMC free article] [PubMed] [Google Scholar]
- Snijder E. J., Ederveen J., Spaan W. J., Weiss M., Horzinek M. C. Characterization of Berne virus genomic and messenger RNAs. J Gen Virol. 1988 Sep;69(Pt 9):2135–2144. doi: 10.1099/0022-1317-69-9-2135. [DOI] [PubMed] [Google Scholar]
- Snijder E. J., Horzinek M. C., Spaan W. J. A 3'-coterminal nested set of independently transcribed mRNAs is generated during Berne virus replication. J Virol. 1990 Jan;64(1):331–338. doi: 10.1128/jvi.64.1.331-338.1990. [DOI] [PMC free article] [PubMed] [Google Scholar]
- Snijder E. J., den Boon J. A., Bredenbeek P. J., Horzinek M. C., Rijnbrand R., Spaan W. J. The carboxyl-terminal part of the putative Berne virus polymerase is expressed by ribosomal frameshifting and contains sequence motifs which indicate that toro- and coronaviruses are evolutionarily related. Nucleic Acids Res. 1990 Aug 11;18(15):4535–4542. doi: 10.1093/nar/18.15.4535. [DOI] [PMC free article] [PubMed] [Google Scholar]
- Spaan W., Cavanagh D., Horzinek M. C. Coronaviruses: structure and genome expression. J Gen Virol. 1988 Dec;69(Pt 12):2939–2952. doi: 10.1099/0022-1317-69-12-2939. [DOI] [PubMed] [Google Scholar]
- Staden R. The current status and portability of our sequence handling software. Nucleic Acids Res. 1986 Jan 10;14(1):217–231. doi: 10.1093/nar/14.1.217. [DOI] [PMC free article] [PubMed] [Google Scholar]
- Timoney P. J., McCollum W. H., Roberts A. W., Murphy T. W. Demonstration of the carrier state in naturally acquired equine arteritis virus infection in the stallion. Res Vet Sci. 1986 Sep;41(2):279–280. [PubMed] [Google Scholar]
- Weiss M., Steck F., Horzinek M. C. Purification and partial characterization of a new enveloped RNA virus (Berne virus). J Gen Virol. 1983 Sep;64(Pt 9):1849–1858. doi: 10.1099/0022-1317-64-9-1849. [DOI] [PubMed] [Google Scholar]
- Westaway E. G., Brinton M. A., Gaidamovich SYa, Horzinek M. C., Igarashi A., Käriäinen L., Lvov D. K., Porterfield J. S., Russell P. K., Trent D. W. Togaviridae. Intervirology. 1985;24(3):125–139. doi: 10.1159/000149632. [DOI] [PubMed] [Google Scholar]
- Zeegers J. J., Van der Zeijst B. A., Horzinek M. C. The structural proteins of equine arteritis virus. Virology. 1976 Aug;73(1):200–205. doi: 10.1016/0042-6822(76)90074-x. [DOI] [PubMed] [Google Scholar]
- de Vries A. A., Chirnside E. D., Bredenbeek P. J., Gravestein L. A., Horzinek M. C., Spaan W. J. All subgenomic mRNAs of equine arteritis virus contain a common leader sequence. Nucleic Acids Res. 1990 Jun 11;18(11):3241–3247. doi: 10.1093/nar/18.11.3241. [DOI] [PMC free article] [PubMed] [Google Scholar]
- ten Dam E. B., Pleij C. W., Bosch L. RNA pseudoknots: translational frameshifting and readthrough on viral RNAs. Virus Genes. 1990 Jul;4(2):121–136. doi: 10.1007/BF00678404. [DOI] [PMC free article] [PubMed] [Google Scholar]
- van Berlo M. F., Horzinek M. C., van der Zeijst B. A. Equine arteritis virus-infected cells contain six polyadenylated virus-specific RNAs. Virology. 1982 Apr 30;118(2):345–352. doi: 10.1016/0042-6822(82)90354-3. [DOI] [PMC free article] [PubMed] [Google Scholar]
- van Berlo M. F., Rottier P. J., Horzinek M. C., van der Zeijst B. A. Intracellular equine arteritis virus (EAV)-specific RNAs contain common sequences. Virology. 1986 Jul 30;152(2):492–496. doi: 10.1016/0042-6822(86)90154-6. [DOI] [PubMed] [Google Scholar]
- van Berlo M. F., Rottier P. J., Spaan W. J., Horzinek M. C. Equine arteritis virus-induced polypeptide synthesis. J Gen Virol. 1986 Aug;67(Pt 8):1543–1549. doi: 10.1099/0022-1317-67-8-1543. [DOI] [PubMed] [Google Scholar]
- van der Zeijst B. A., Horzinek M. C. The genome of equine arteritis virus. Virology. 1975 Dec;68(2):418–425. doi: 10.1016/0042-6822(75)90283-4. [DOI] [PubMed] [Google Scholar]