Abstract
This research documents the multiplicity of L-aspartate transport in thin wastewater biofilms. A Line-weaver-Burk analysis of incorporation produced a curvilinear plot (concave down) that suggested active transport by two distinct systems (1 and 2). The inactivation of system 2 with AsO4 or osmotic shock resolved system 1, which was a high-affinity, low-capacity system with an apparent Kt (Michaelis-Menten constant) of 4.3 microM (AsO4) or 4.6 microM (osmotic shock). The inactivation of system 1 with dinitrophenol resolved system 2, which was a low-affinity, high-capacity system with an apparent Kt of 116.7 microM. System 1 was more specific for aspartate than system 2 in the presence of aspartate analogs. Sodium had no discernible effect on the incorporation velocities by either system. These results indicate that system 1 is a membrane-bound proton symport coupled to the proton gradient component of the proton motive force and that system 2 is a binding protein-mediated system coupled to phosphate bond energy. Analyses of diffusional limitations on the derived transport constants indicated that internal resistances were present but that the apparent constants were close to the intrinsic values, especially for system 1. Metabolic inactivation of the biofilm with dinitrophenol and AsO4 did not completely inactivate aspartate incorporation, which indicated that some simple adsorption of the aspartate anion by the biofilm had occurred. These results show that aspartate is transported by wastewater biofilm bacteria via systems with different affinities, specificities, and mechanisms of energy coupling.
Full text
PDF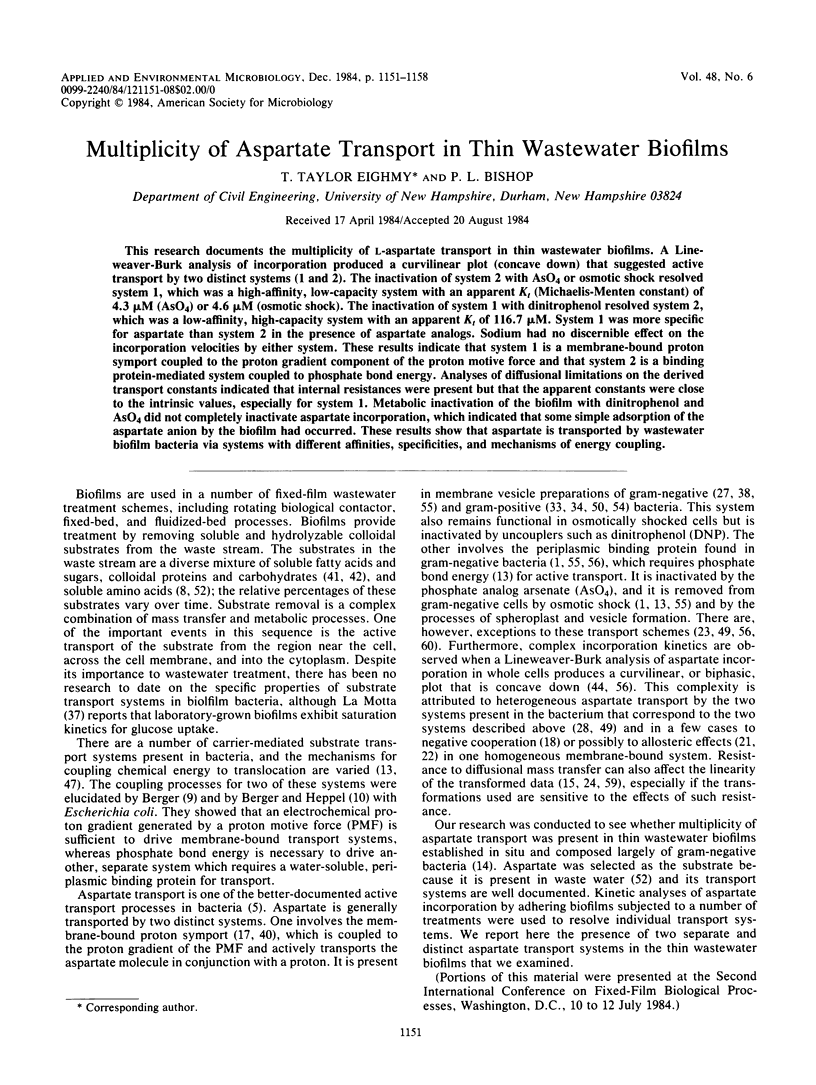
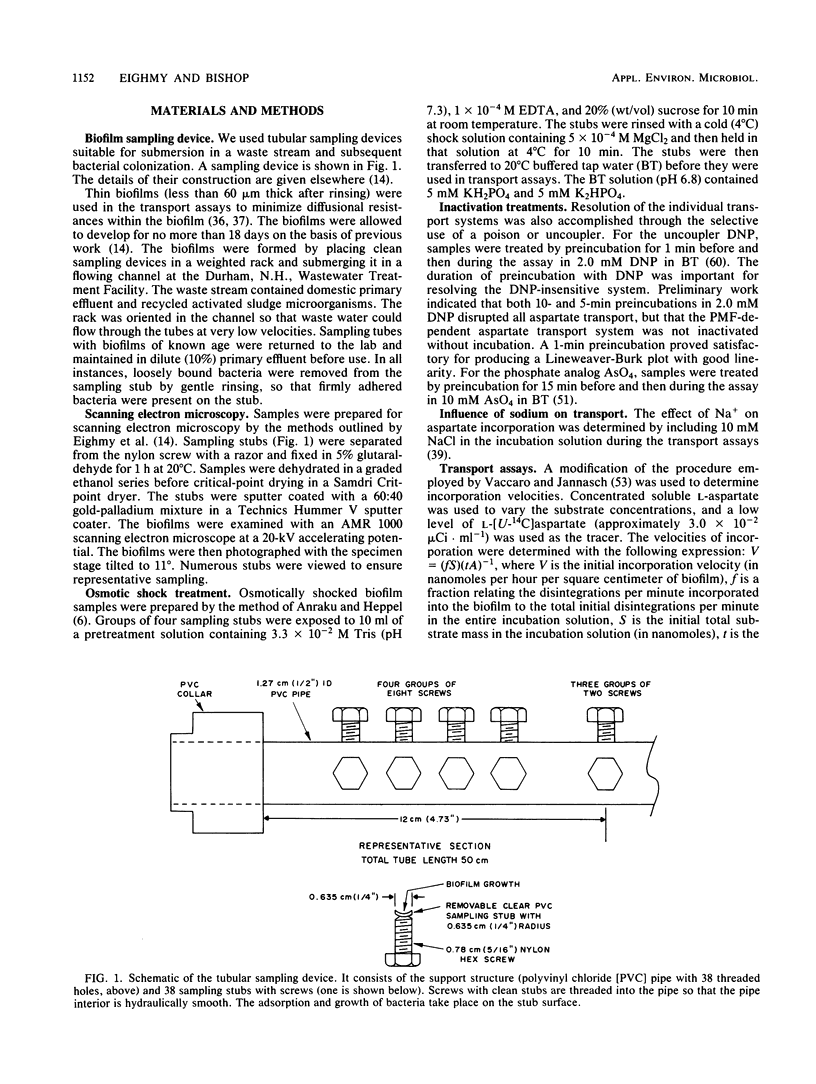
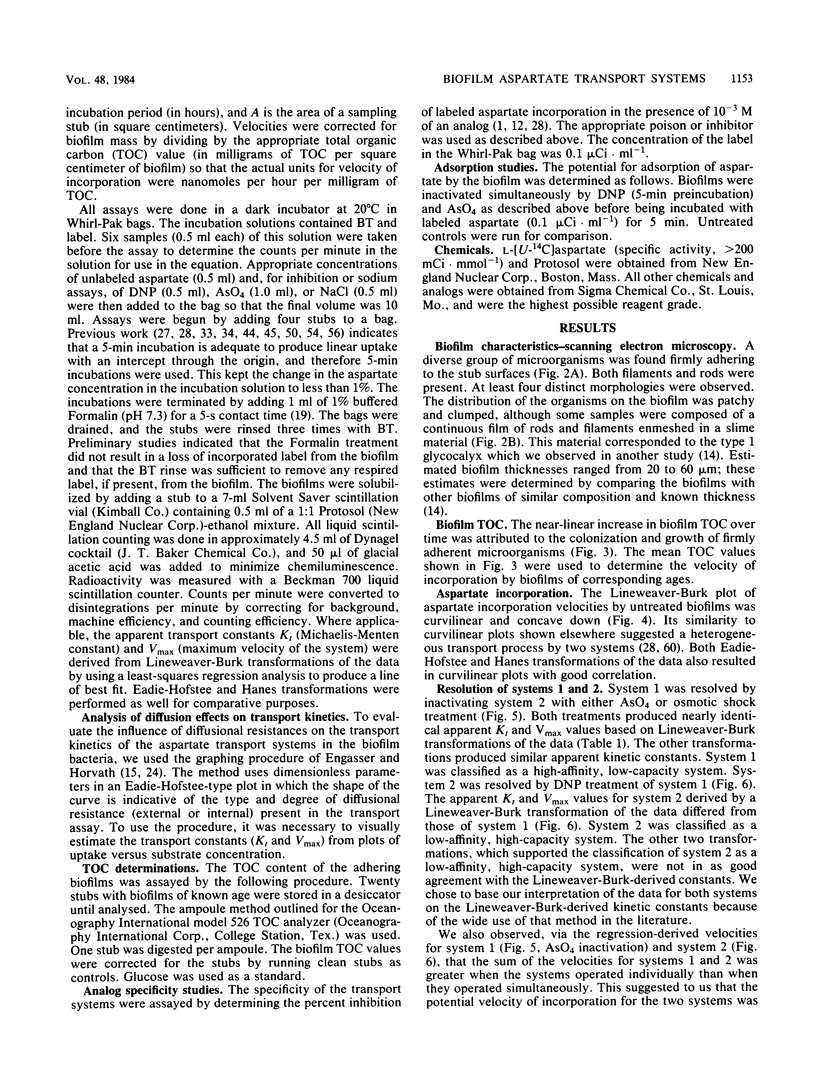
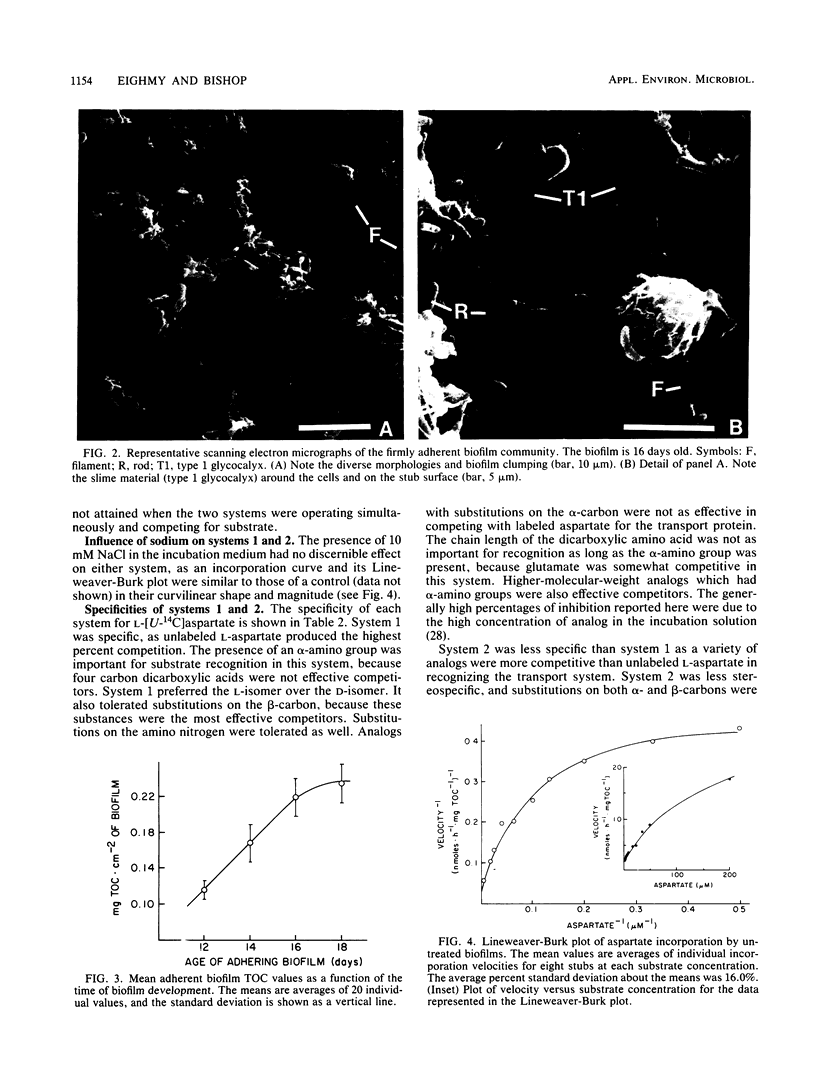
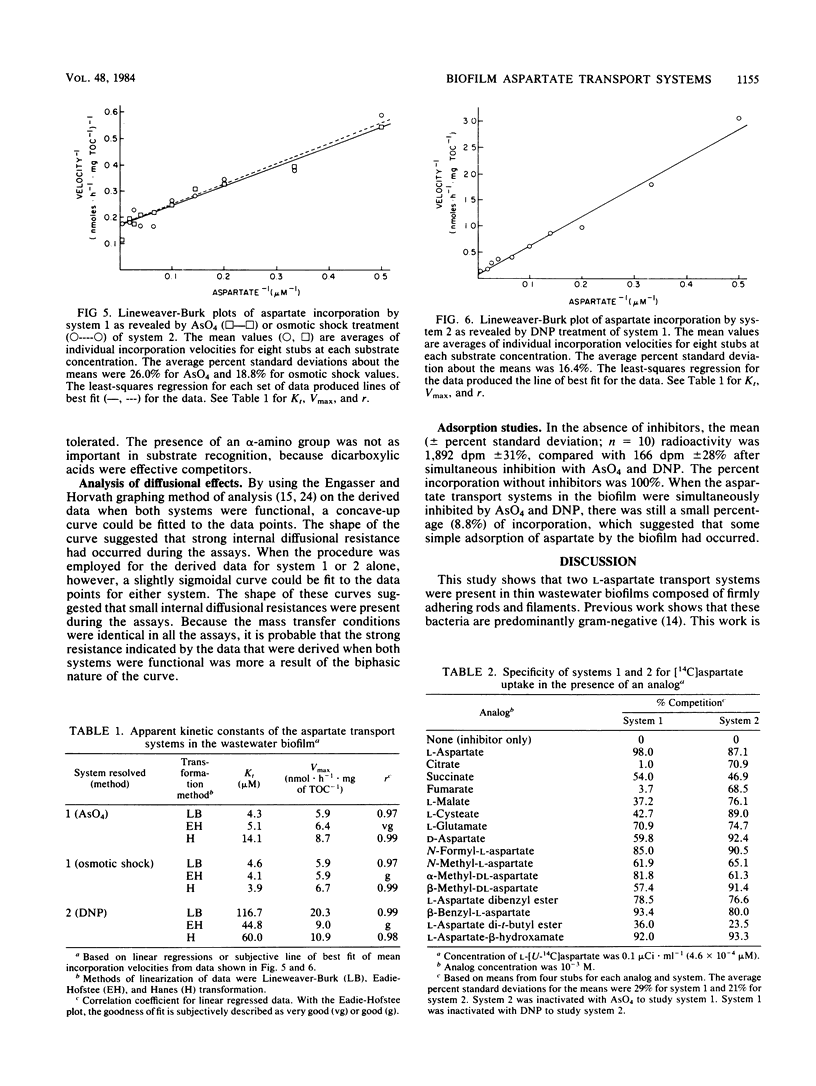
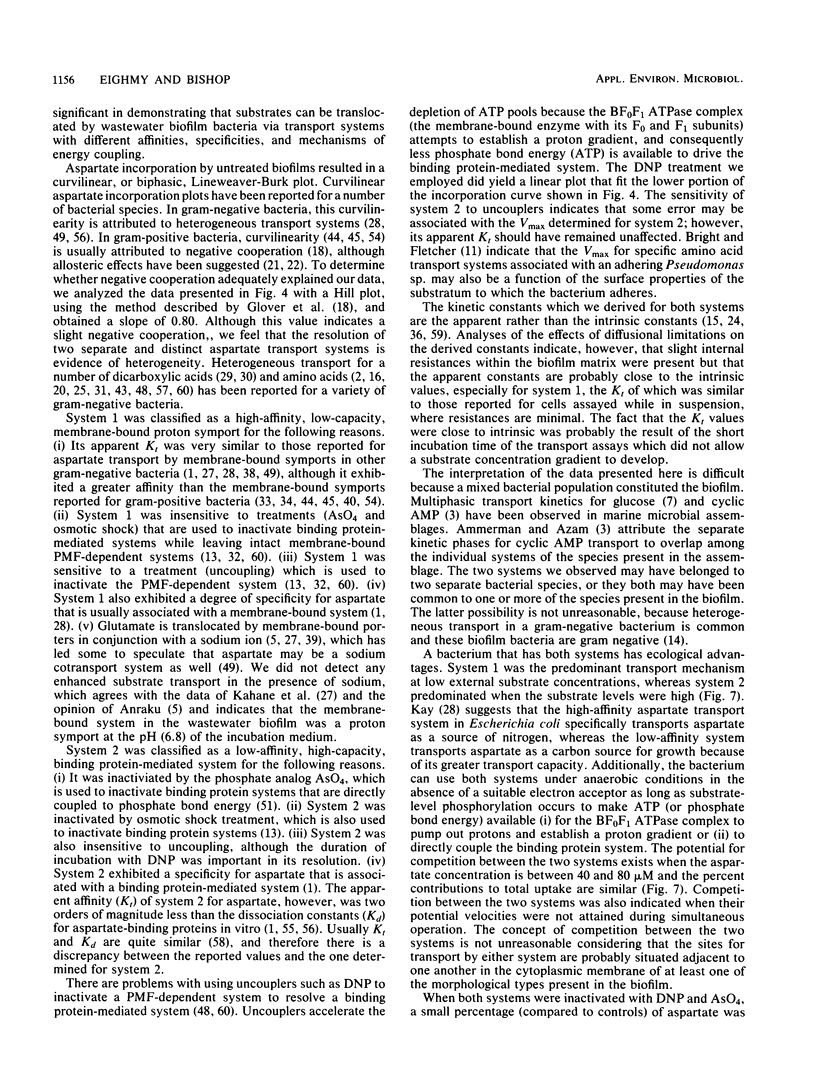
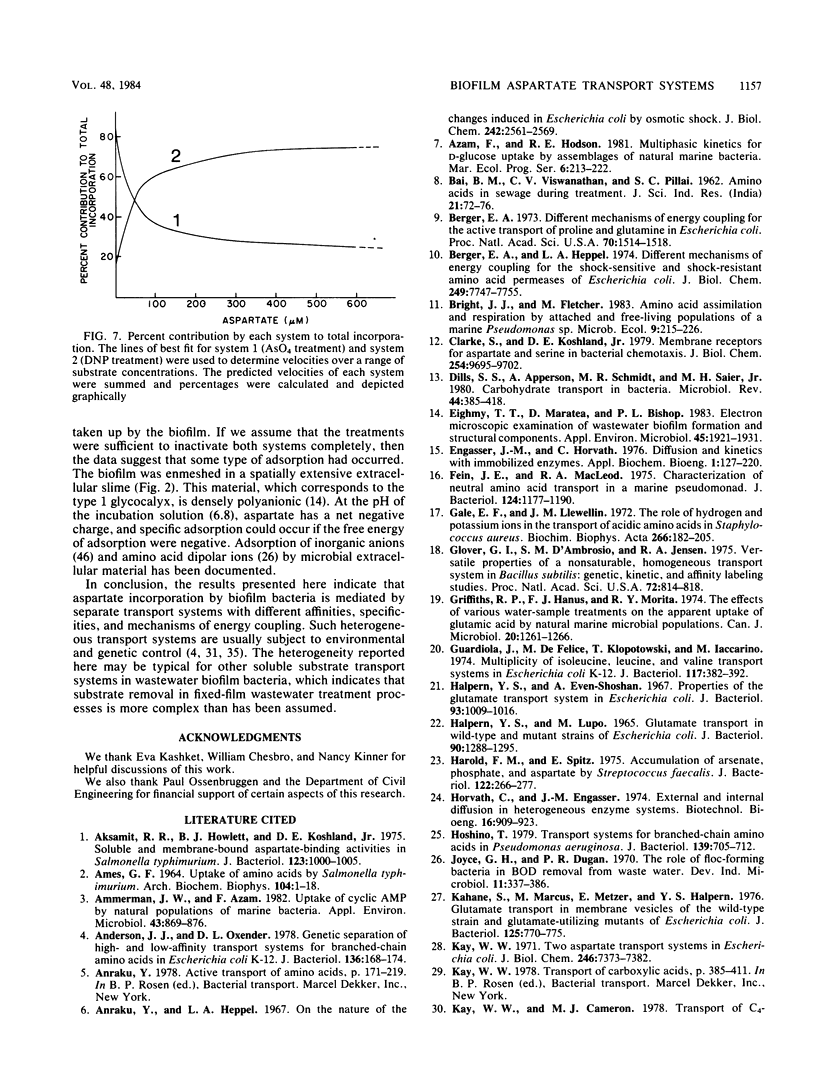
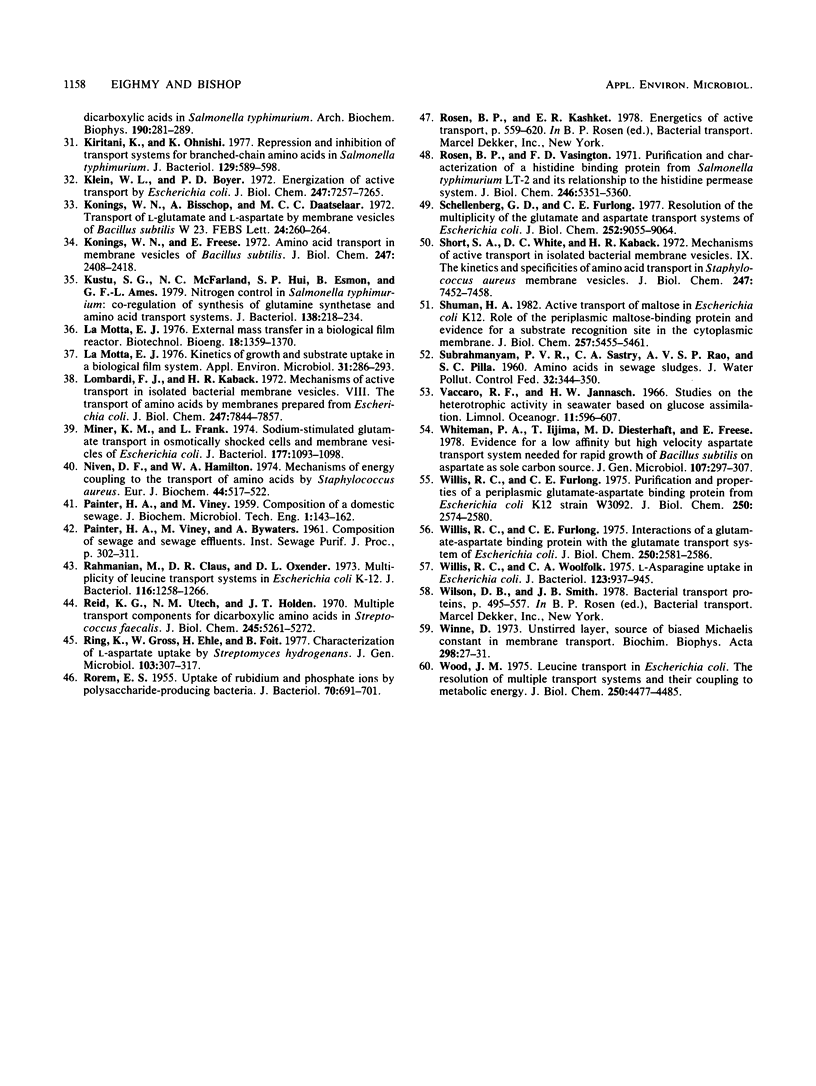
Images in this article
Selected References
These references are in PubMed. This may not be the complete list of references from this article.
- AMES G. F. UPTAKE OF AMINO ACIDS BY SALMONELLA TYPHIMURIUM. Arch Biochem Biophys. 1964 Jan;104:1–18. doi: 10.1016/s0003-9861(64)80028-x. [DOI] [PubMed] [Google Scholar]
- Aksamit R. R., Howlett B. J., Koshland D. E., Jr Soluble and membrane-bound aspartate-binding activities in Salmonella typhimurium. J Bacteriol. 1975 Sep;123(3):1000–1005. doi: 10.1128/jb.123.3.1000-1005.1975. [DOI] [PMC free article] [PubMed] [Google Scholar]
- Ammerman J. W., Azam F. Uptake of Cyclic AMP by Natural Populations of Marine Bacteria. Appl Environ Microbiol. 1982 Apr;43(4):869–876. doi: 10.1128/aem.43.4.869-876.1982. [DOI] [PMC free article] [PubMed] [Google Scholar]
- Anderson J. J., Oxender D. L. Genetic separation of high- and low-affinity transport systems for branched-chain amino acids in Escherichia coli K-12. J Bacteriol. 1978 Oct;136(1):168–174. doi: 10.1128/jb.136.1.168-174.1978. [DOI] [PMC free article] [PubMed] [Google Scholar]
- Anraku Y., Heppel L. A. On the nature of the changes induced in Escherichia coli by osmotic shock. J Biol Chem. 1967 May 25;242(10):2561–2569. [PubMed] [Google Scholar]
- Berger E. A. Different mechanisms of energy coupling for the active transport of proline and glutamine in Escherichia coli. Proc Natl Acad Sci U S A. 1973 May;70(5):1514–1518. doi: 10.1073/pnas.70.5.1514. [DOI] [PMC free article] [PubMed] [Google Scholar]
- Berger E. A., Heppel L. A. Different mechanisms of energy coupling for the shock-sensitive and shock-resistant amino acid permeases of Escherichia coli. J Biol Chem. 1974 Dec 25;249(24):7747–7755. [PubMed] [Google Scholar]
- Clarke S., Koshland D. E., Jr Membrane receptors for aspartate and serine in bacterial chemotaxis. J Biol Chem. 1979 Oct 10;254(19):9695–9702. [PubMed] [Google Scholar]
- Dills S. S., Apperson A., Schmidt M. R., Saier M. H., Jr Carbohydrate transport in bacteria. Microbiol Rev. 1980 Sep;44(3):385–418. doi: 10.1128/mr.44.3.385-418.1980. [DOI] [PMC free article] [PubMed] [Google Scholar]
- Eighmy T. T., Maratea D., Bishop P. L. Electron microscopic examination of wastewater biofilm formation and structural components. Appl Environ Microbiol. 1983 Jun;45(6):1921–1931. doi: 10.1128/aem.45.6.1921-1931.1983. [DOI] [PMC free article] [PubMed] [Google Scholar]
- Fein J. E., MacLeod R. A. Characterization of neutral amino acid transport in a marine pseudomonad. J Bacteriol. 1975 Dec;124(3):1177–1190. doi: 10.1128/jb.124.3.1177-1190.1975. [DOI] [PMC free article] [PubMed] [Google Scholar]
- Gale E. F., Llewellin J. M. The role of hydrogen and potassium ions in the transport of acidic amino acids in Staphylococcus aureus. Biochim Biophys Acta. 1972 Apr 14;266(1):182–205. doi: 10.1016/0005-2736(72)90134-4. [DOI] [PubMed] [Google Scholar]
- Glover G. I., D'Ambrosio S. M., Jensen R. A. Versatile properties of a nonsaturable, homogeneous transport system in Bacilus subtilis: genetic, kinetic, and affinity labeling studies. Proc Natl Acad Sci U S A. 1975 Mar;72(3):814–818. doi: 10.1073/pnas.72.3.814. [DOI] [PMC free article] [PubMed] [Google Scholar]
- Griffiths R. P., Hanus F. J., Morita R. Y. The effects of various water-sample treatments on the apparent uptake of glutamic acid by natural marine microbial populations. Can J Microbiol. 1974 Sep;20(9):1261–1266. doi: 10.1139/m74-194. [DOI] [PubMed] [Google Scholar]
- Guardiola J., De Felice M., Klopotowski T., Iaccarino M. Multiplicity of isoleucine, leucine, and valine transport systems in Escherichia coli K-12. J Bacteriol. 1974 Feb;117(2):382–392. doi: 10.1128/jb.117.2.382-392.1974. [DOI] [PMC free article] [PubMed] [Google Scholar]
- Halpern Y. S., Even-Shoshan A. Properties of the glutamate transport system in Escherichia coli. J Bacteriol. 1967 Mar;93(3):1009–1016. doi: 10.1128/jb.93.3.1009-1016.1967. [DOI] [PMC free article] [PubMed] [Google Scholar]
- Halpern Y. S., Lupo M. Glutamate transport in wild-type and mutant strains of Escherichia coli. J Bacteriol. 1965 Nov;90(5):1288–1295. doi: 10.1128/jb.90.5.1288-1295.1965. [DOI] [PMC free article] [PubMed] [Google Scholar]
- Harold F. M., Spitz E. Accumulation of arsenate, phosphate, and aspartate by Sreptococcus faecalis. J Bacteriol. 1975 Apr;122(1):266–277. doi: 10.1128/jb.122.1.266-277.1975. [DOI] [PMC free article] [PubMed] [Google Scholar]
- Horvath C., Engasser J. M. External and internal diffusion in heterogeneous enzymes systems. Biotechnol Bioeng. 1974 Jul;16(7):909–923. doi: 10.1002/bit.260160705. [DOI] [PubMed] [Google Scholar]
- Hoshino T. Transport systems for branched-chain amino acids in Pseudomonas aeruginosa. J Bacteriol. 1979 Sep;139(3):705–712. doi: 10.1128/jb.139.3.705-712.1979. [DOI] [PMC free article] [PubMed] [Google Scholar]
- Kahane S., Marcus M., Metzer E., Halpern Y. S. Glutamate transport in membrane vesicles of the wild-type strain and glutamate-utilizing mutants of Escherichia coli. J Bacteriol. 1976 Mar;125(3):770–775. doi: 10.1128/jb.125.3.770-775.1976. [DOI] [PMC free article] [PubMed] [Google Scholar]
- Kay W. W., Cameron M. J. Transport of C4-dicarboxylic acids in salmonella typhimurium. Arch Biochem Biophys. 1978 Sep;190(1):281–289. doi: 10.1016/0003-9861(78)90277-1. [DOI] [PubMed] [Google Scholar]
- Kay W. W. Two aspartate transport systems in Escherichia coli. J Biol Chem. 1971 Dec 10;246(23):7373–7382. [PubMed] [Google Scholar]
- Kiritani K., Ohnishi K. Repression and inhibition of transport systems for branched-chain amino acids in Salmonella typhimurium. J Bacteriol. 1977 Feb;129(2):589–598. doi: 10.1128/jb.129.2.589-598.1977. [DOI] [PMC free article] [PubMed] [Google Scholar]
- Klein W. L., Boyer P. D. Energization of active transport by Escherichia coli. J Biol Chem. 1972 Nov 25;247(22):7257–7265. [PubMed] [Google Scholar]
- Konings W. N., Bisschop A., Daatselaar M. C.C. Transport of L-glutamate and L-aspartate by membrane vesicles of Bacillus subtilis W 23. FEBS Lett. 1972 Aug 15;24(3):260–264. doi: 10.1016/0014-5793(72)80368-5. [DOI] [PubMed] [Google Scholar]
- Konings W. N., Freese E. Amino acid transport in membrane vesicles of Bacillus subtilis. J Biol Chem. 1972 Apr 25;247(8):2408–2418. [PubMed] [Google Scholar]
- Kustu S. G., McFarland N. C., Hui S. P., Esmon B., Ames G. F. Nitrogen control of Salmonella typhimurium: co-regulation of synthesis of glutamine synthetase and amino acid transport systems. J Bacteriol. 1979 Apr;138(1):218–234. doi: 10.1128/jb.138.1.218-234.1979. [DOI] [PMC free article] [PubMed] [Google Scholar]
- La Motta E. J. Kinetics of growth and substrate uptake in a biological film system. Appl Environ Microbiol. 1976 Feb;31(2):286–293. doi: 10.1128/aem.31.2.286-293.1976. [DOI] [PMC free article] [PubMed] [Google Scholar]
- Lombardi F. J., Kaback H. R. Mechanisms of active transport in isolated bacterial membrane vesicles. 8. The transport of amino acids by membranes prepared from Escherichia coli. J Biol Chem. 1972 Dec 25;247(24):7844–7857. [PubMed] [Google Scholar]
- Miner K. M., Frank L. Sodium-stimulated glutamate transport in osmotically shocked cells and membrane vesicles of Escherichia coli. J Bacteriol. 1974 Mar;117(3):1093–1098. doi: 10.1128/jb.117.3.1093-1098.1974. [DOI] [PMC free article] [PubMed] [Google Scholar]
- Niven D. F., Hamilton W. A. Mechanisms of energy coupling to the transport of amino acids by Staphylococcus aureus. Eur J Biochem. 1974 May 15;44(2):517–522. doi: 10.1111/j.1432-1033.1974.tb03510.x. [DOI] [PubMed] [Google Scholar]
- ROREM E. S. Uptake of rubidium and phosphate ions by polysaccharide-producing bacteria. J Bacteriol. 1955 Dec;70(6):691–701. doi: 10.1128/jb.70.6.691-701.1955. [DOI] [PMC free article] [PubMed] [Google Scholar]
- Rahmanian M., Claus D. R., Oxender D. L. Multiplicity of leucine transport systems in Escherichia coli K-12. J Bacteriol. 1973 Dec;116(3):1258–1266. doi: 10.1128/jb.116.3.1258-1266.1973. [DOI] [PMC free article] [PubMed] [Google Scholar]
- Reid K. G., Utech N. M., Holden J. T. Multiple transport components for dicarboxylic amino acids in Streptococcus faecalis. J Biol Chem. 1970 Oct 25;245(20):5261–5272. [PubMed] [Google Scholar]
- Ring K., Gross W., Ehle H., Foit B. Characterization of L-aspartate uptake by Streptomyces hydrogenans. J Gen Microbiol. 1977 Dec;103(2):307–317. doi: 10.1099/00221287-103-2-307. [DOI] [PubMed] [Google Scholar]
- Rosen B. P., Vasington F. D. Purification and characterization of a histidine-binding protein from Salmonella typhimurium LT-2 and its relationship to the histidine permease system. J Biol Chem. 1971 Sep 10;246(17):5351–5360. [PubMed] [Google Scholar]
- Schellenberg G. D., Furlong C. E. Resolution of the multiplicity of the glutamate and aspartate transport systems of Escherichia coli. J Biol Chem. 1977 Dec 25;252(24):9055–9064. [PubMed] [Google Scholar]
- Short S. A., White D. C., Kaback H. R. Mechanisms of active transport in isolated bacterial membrane vesicles. IX. The kinetics and specificity of amino acid transport in Staphylococcus aureus membrane vesicles. J Biol Chem. 1972 Dec 10;247(23):7452–7458. [PubMed] [Google Scholar]
- Shuman H. A. Active transport of maltose in Escherichia coli K12. Role of the periplasmic maltose-binding protein and evidence for a substrate recognition site in the cytoplasmic membrane. J Biol Chem. 1982 May 25;257(10):5455–5461. [PubMed] [Google Scholar]
- Willis R. C., Furlong C. E. Interactions of a glutamate-aspartate binding protein with the glutamate transport system of Escherichia coli. J Biol Chem. 1975 Apr 10;250(7):2581–2586. [PubMed] [Google Scholar]
- Willis R. C., Furlong C. E. Purification and properties of a periplasmic glutamate-aspartate binding protein from Escherichia coli K12 strain W3092. J Biol Chem. 1975 Apr 10;250(7):2574–2580. [PubMed] [Google Scholar]
- Willis R. C., Woolfolk C. A. L-asparagine uptake in Escherichia coli. J Bacteriol. 1975 Sep;123(3):937–945. doi: 10.1128/jb.123.3.937-945.1975. [DOI] [PMC free article] [PubMed] [Google Scholar]
- Winne D. Unstirred layer, source of biased Michaelis constant in membrane transport. Biochim Biophys Acta. 1973 Feb 27;298(1):27–31. doi: 10.1016/0005-2736(73)90005-9. [DOI] [PubMed] [Google Scholar]
- Wood J. M. Leucine transport in Escherichia coli. The resolution of multiple transport systems and their coupling to metabolic energy. J Biol Chem. 1975 Jun 25;250(12):4477–4485. [PubMed] [Google Scholar]