Abstract
Levels of DNA, RNA, protein, ATP, glutathione, and radioactivity associated with [35S]methionine-labeled cellular protein were estimated at various times during the starvation-survival process of a marine psychrophilic heterotrophic Vibrio sp., Ant-300. Values for the macromolecules were analyzed in terms of total, viable, and respiring cells. Electron micrographs (thin sections) were made on log-phase and 5.5-week-starved cells. On a per-cell basis, the levels of protein and DNA rapidly decreased until a constant level was attained. A second method in which radioactive sulfur was used for monitoring protein demonstrated that the cellular protein level decreased for approximately 2.5 weeks and then remained constant. An initial decrease in the RNA level with starvation was noted, but with time the RNA (orcinol-positive material) level increased to 2.5 times the minimum level. After 6 weeks of starvation, 45 to 60% of the cells remained capable of respiration, as determined by iodonitrotetrazolium violet-formazan granule production. Potential respiration and endogenous respiration levels fell, with an intervening 1-week peak, until at 2 weeks no endogenous respiration could be measured; respiratory potential remained high. The cell glutathione level fell during starvation, but when the cells were starved in the presence of the appropriate amino acids, glutathione was resynthesized to its original level, beginning after 1 week of starvation. The cells used much of their stored products and became ultramicrocells during the 6-week starvation-survival process. Ant-300 underwent many physiological changes in the first week of starvation that relate to the utilization or production of ATP. After that period, a stable pattern for long-term starvation was demonstrated.
Full text
PDF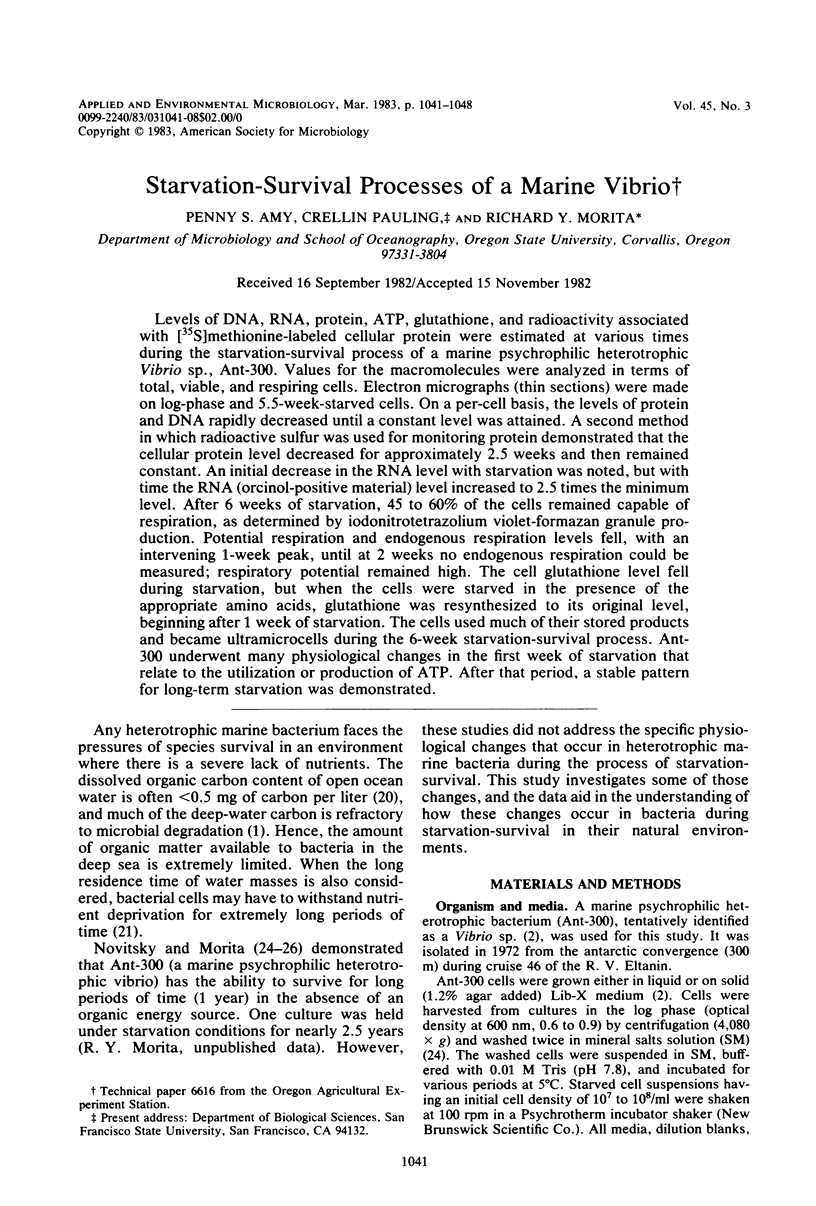
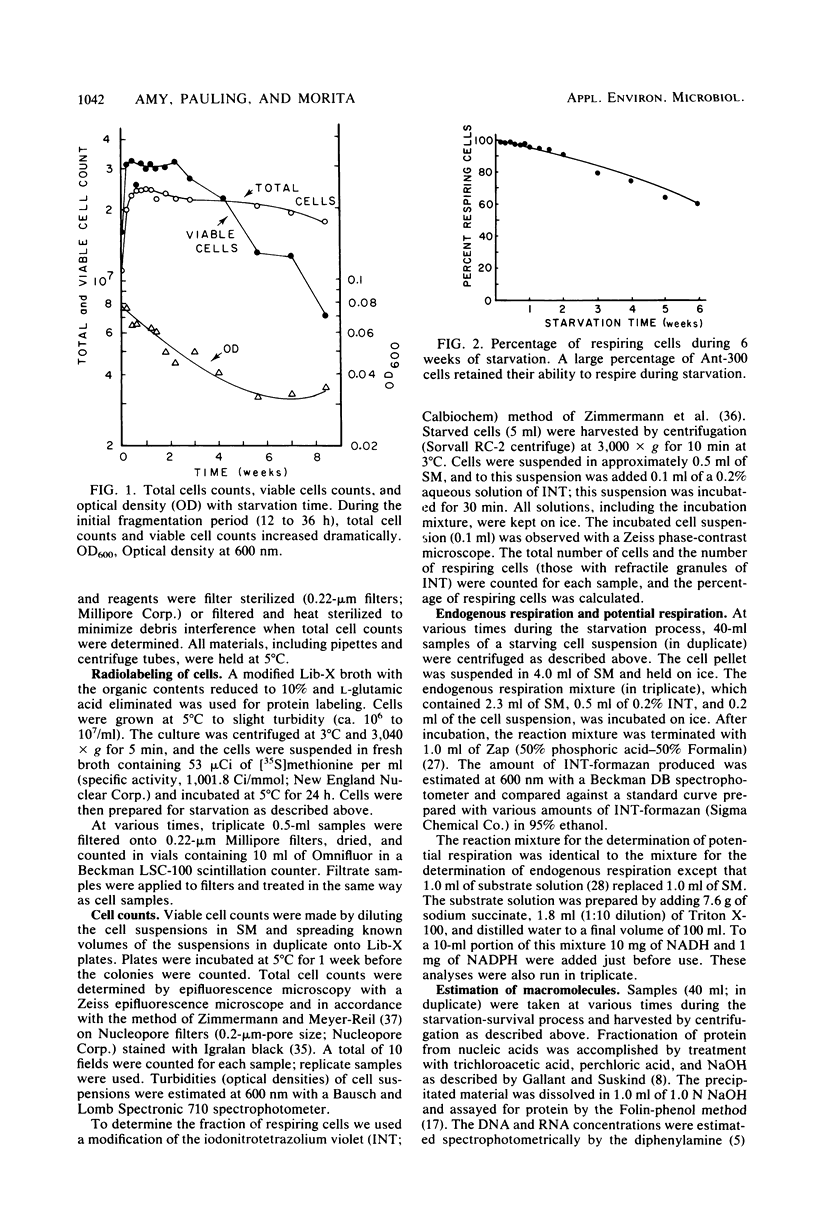
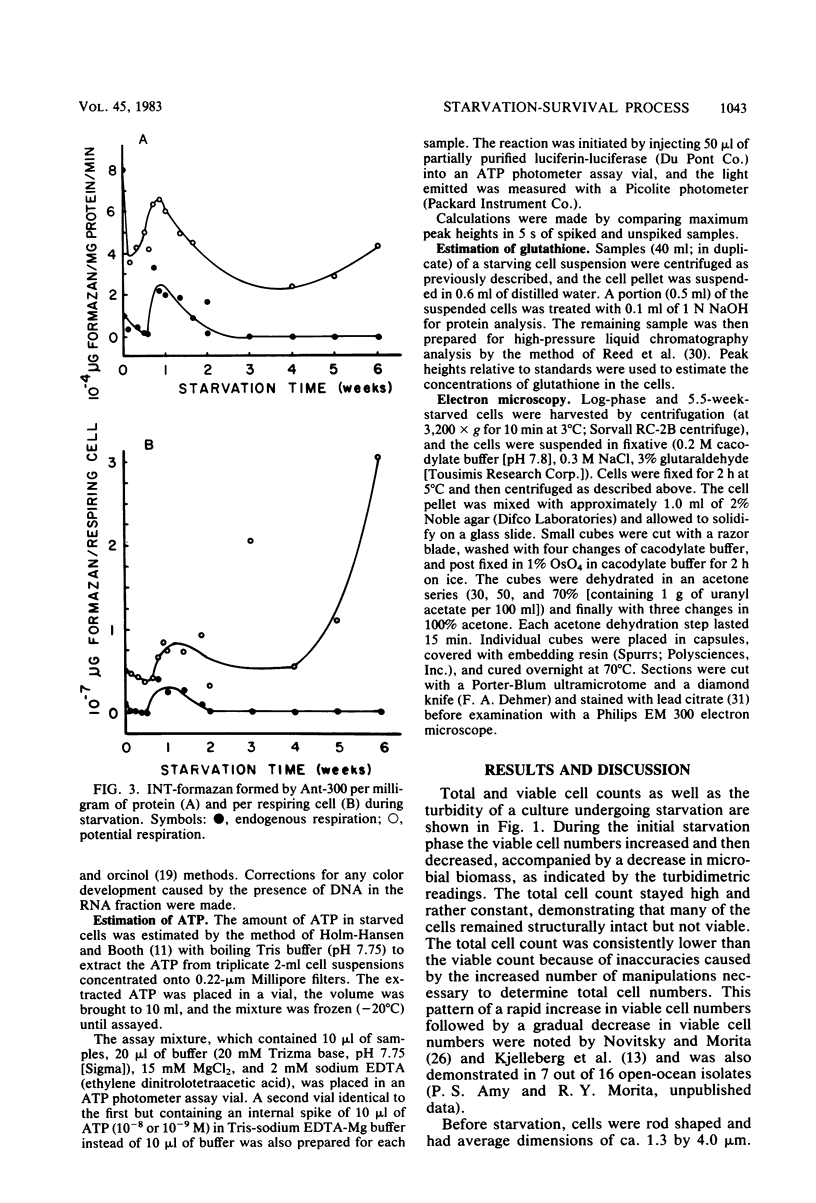
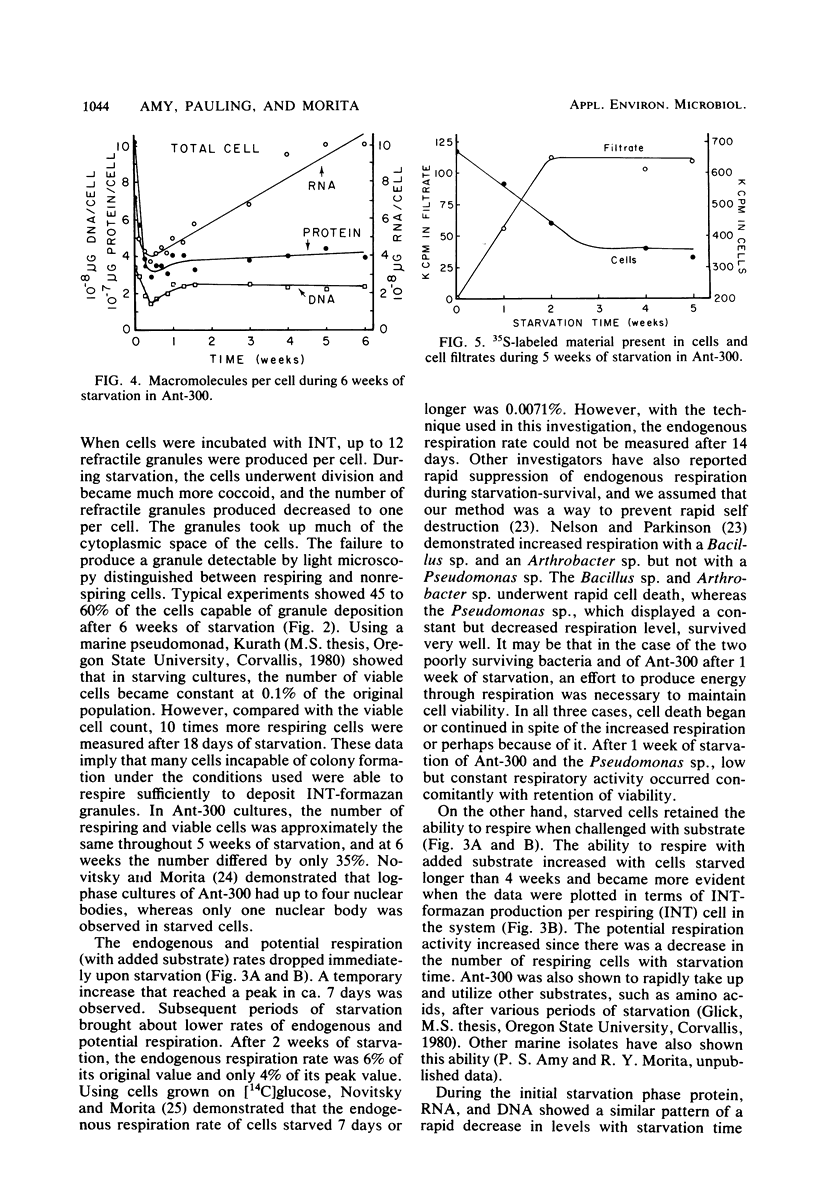
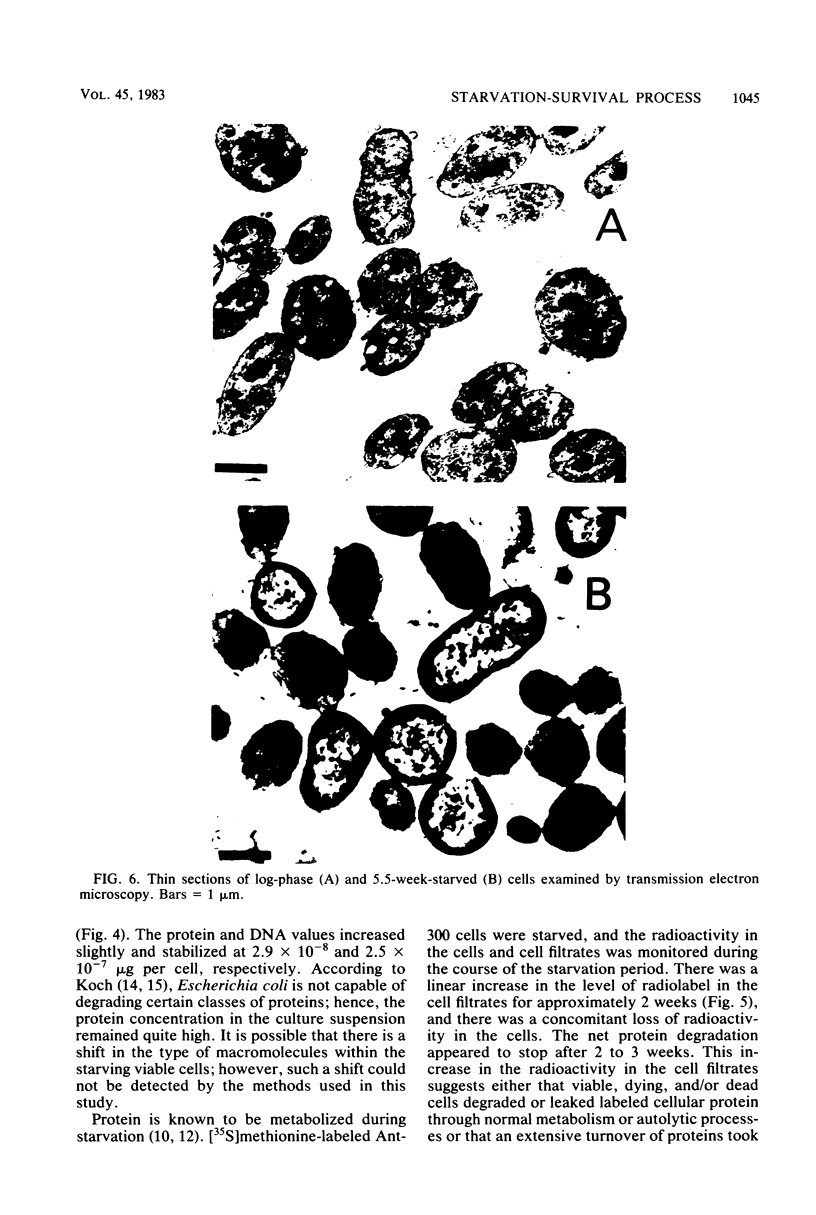
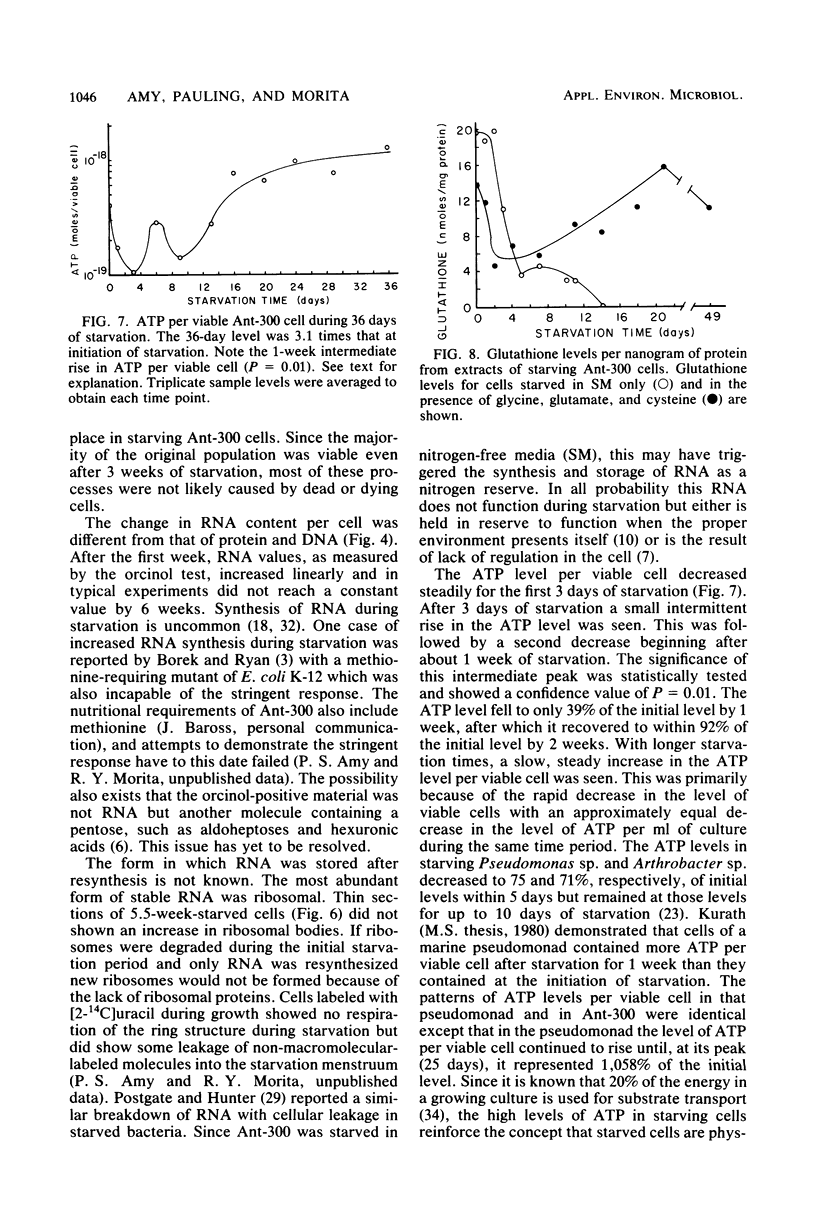
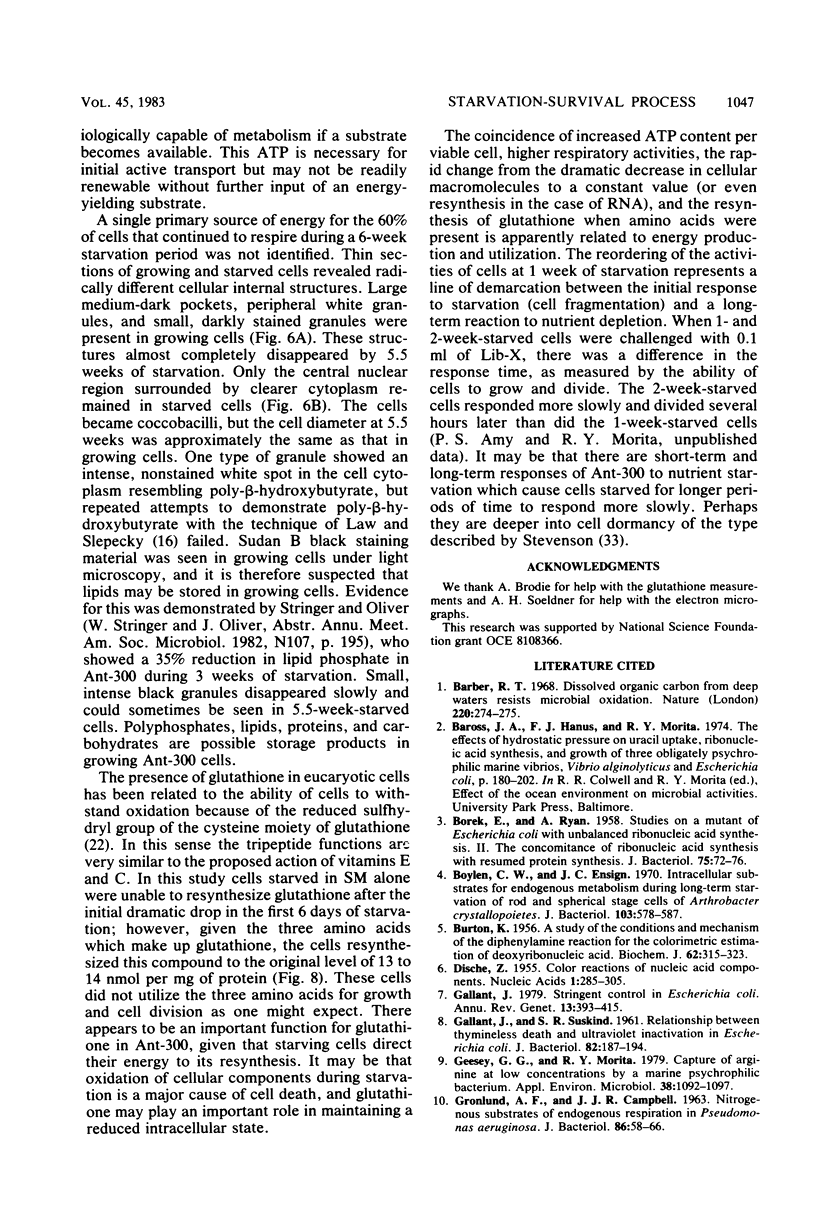
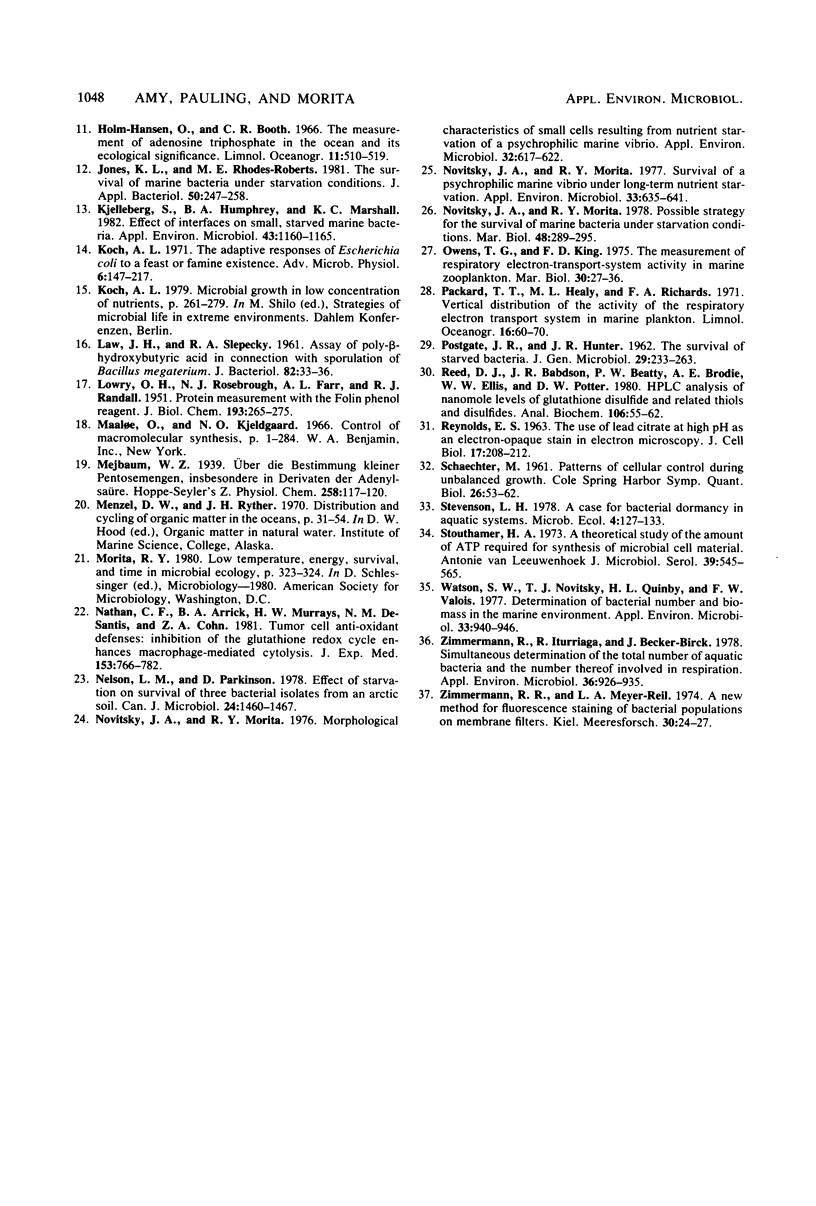
Images in this article
Selected References
These references are in PubMed. This may not be the complete list of references from this article.
- BOREK E., RYAN A. Studies on a mutant of Escherichia coli with unbalanced ribonucleic acid synthesis. II. The concomitance of ribonucleic acid synthesis with resumed protein synthesis. J Bacteriol. 1958 Jan;75(1):72–76. doi: 10.1128/jb.75.1.72-76.1958. [DOI] [PMC free article] [PubMed] [Google Scholar]
- BURTON K. A study of the conditions and mechanism of the diphenylamine reaction for the colorimetric estimation of deoxyribonucleic acid. Biochem J. 1956 Feb;62(2):315–323. doi: 10.1042/bj0620315. [DOI] [PMC free article] [PubMed] [Google Scholar]
- Barber R. T. Dissolved organic carbon from deep waters resists microbial oxidation. Nature. 1968 Oct 19;220(5164):274–275. doi: 10.1038/220274a0. [DOI] [PubMed] [Google Scholar]
- Boylen C. W., Ensign J. C. Intracellular substrates for endogenous metabolism during long-term starvation of rod and spherical cells of Arthrobacter crystallopoietes. J Bacteriol. 1970 Sep;103(3):578–587. doi: 10.1128/jb.103.3.578-587.1970. [DOI] [PMC free article] [PubMed] [Google Scholar]
- Christian R. R., Hanson R. B., Newell S. Y. Comparison of methods for measurement of bacterial growth rates in mixed batch cultures. Appl Environ Microbiol. 1982 May;43(5):1160–1165. doi: 10.1128/aem.43.5.1160-1165.1982. [DOI] [PMC free article] [PubMed] [Google Scholar]
- GALLANT J., SUSKIND S. R. Relationship between thymineless death and ultraviolet inactivation in Escherichia coli. J Bacteriol. 1961 Aug;82:187–194. doi: 10.1128/jb.82.2.187-194.1961. [DOI] [PMC free article] [PubMed] [Google Scholar]
- GRONLUND A. F., CAMPBELL J. J. NITROGENOUS SUBSTRATES OF ENDOGENOUS RESPIRATION IN PSEUDOMONAS AERUGINOSA. J Bacteriol. 1963 Jul;86:58–66. doi: 10.1128/jb.86.1.58-66.1963. [DOI] [PMC free article] [PubMed] [Google Scholar]
- Gallant J. A. Stringent control in E. coli. Annu Rev Genet. 1979;13:393–415. doi: 10.1146/annurev.ge.13.120179.002141. [DOI] [PubMed] [Google Scholar]
- Geesey G. G., Morita R. Y. Capture of arginine at low concentrations by a marine psychrophilic bacterium. Appl Environ Microbiol. 1979 Dec;38(6):1092–1097. doi: 10.1128/aem.38.6.1092-1097.1979. [DOI] [PMC free article] [PubMed] [Google Scholar]
- Koch A. L. The adaptive responses of Escherichia coli to a feast and famine existence. Adv Microb Physiol. 1971;6:147–217. doi: 10.1016/s0065-2911(08)60069-7. [DOI] [PubMed] [Google Scholar]
- LAW J. H., SLEPECKY R. A. Assay of poly-beta-hydroxybutyric acid. J Bacteriol. 1961 Jul;82:33–36. doi: 10.1128/jb.82.1.33-36.1961. [DOI] [PMC free article] [PubMed] [Google Scholar]
- LOWRY O. H., ROSEBROUGH N. J., FARR A. L., RANDALL R. J. Protein measurement with the Folin phenol reagent. J Biol Chem. 1951 Nov;193(1):265–275. [PubMed] [Google Scholar]
- Nathan C. F., Arrick B. A., Murray H. W., DeSantis N. M., Cohn Z. A. Tumor cell anti-oxidant defenses. Inhibition of the glutathione redox cycle enhances macrophage-mediated cytolysis. J Exp Med. 1981 Apr 1;153(4):766–782. doi: 10.1084/jem.153.4.766. [DOI] [PMC free article] [PubMed] [Google Scholar]
- Nelson L. M., Parkinson D. Effect of starvation on survival of three bacterial isolates from an arctic soil. Can J Microbiol. 1978 Dec;24(12):1460–1467. doi: 10.1139/m78-235. [DOI] [PubMed] [Google Scholar]
- Novitsky J. A., Morita R. Y. Morphological characterization of small cells resulting from nutrient starvation of a psychrophilic marine vibrio. Appl Environ Microbiol. 1976 Oct;32(4):617–622. doi: 10.1128/aem.32.4.617-622.1976. [DOI] [PMC free article] [PubMed] [Google Scholar]
- Novitsky J. A., Morita R. Y. Survival of a psychrophilic marine Vibrio under long-term nutrient starvation. Appl Environ Microbiol. 1977 Mar;33(3):635–641. doi: 10.1128/aem.33.3.635-641.1977. [DOI] [PMC free article] [PubMed] [Google Scholar]
- POSTGATE J. R., HUNTER J. R. The survival of starved bacteria. J Gen Microbiol. 1962 Oct;29:233–263. doi: 10.1099/00221287-29-2-233. [DOI] [PubMed] [Google Scholar]
- REYNOLDS E. S. The use of lead citrate at high pH as an electron-opaque stain in electron microscopy. J Cell Biol. 1963 Apr;17:208–212. doi: 10.1083/jcb.17.1.208. [DOI] [PMC free article] [PubMed] [Google Scholar]
- Reed D. J., Babson J. R., Beatty P. W., Brodie A. E., Ellis W. W., Potter D. W. High-performance liquid chromatography analysis of nanomole levels of glutathione, glutathione disulfide, and related thiols and disulfides. Anal Biochem. 1980 Jul 15;106(1):55–62. doi: 10.1016/0003-2697(80)90118-9. [DOI] [PubMed] [Google Scholar]
- SCHAECHTER M. Patterns of cellular control during unbalanced growth. Cold Spring Harb Symp Quant Biol. 1961;26:53–62. doi: 10.1101/sqb.1961.026.01.011. [DOI] [PubMed] [Google Scholar]
- Stouthamer A. H. A theoretical study on the amount of ATP required for synthesis of microbial cell material. Antonie Van Leeuwenhoek. 1973;39(3):545–565. doi: 10.1007/BF02578899. [DOI] [PubMed] [Google Scholar]
- Watson S. W., Novitsky T. J., Quinby H. L., Valois F. W. Determination of bacterial number and biomass in the marine environment. Appl Environ Microbiol. 1977 Apr;33(4):940–946. doi: 10.1128/aem.33.4.940-946.1977. [DOI] [PMC free article] [PubMed] [Google Scholar]
- Zimmermann R., Iturriaga R., Becker-Birck J. Simultaneous determination of the total number of aquatic bacteria and the number thereof involved in respiration. Appl Environ Microbiol. 1978 Dec;36(6):926–935. doi: 10.1128/aem.36.6.926-935.1978. [DOI] [PMC free article] [PubMed] [Google Scholar]