Abstract
Two temperature-sensitive mutants (lysS1 and lysS2) of the lysyl-transfer ribonucleic acid synthetase (l-lysine:tRNA ligase [adenosine 5′-monophosphate], EC 6.1.1.6) of Bacillus subtilis have been isolated. Although protein synthesis is inhibited in both mutants at the restrictive temperature (42 to 45 C), the mutants remain viable in a minimal medium. In comparison with the wild-type lysyl-tRNA synthetase, the l-lysine-dependent exchange of [32P]pyrophosphate with adenosine 5′-triphosphate (ATP) for both mutant enzymes is decreased. The lysS1 enzyme is completely defective in the ATP-dependent attachment of l-lysine to tRNA, whereas the lysS2 enzyme has 3- to 10-fold reduced levels of this activity. Temperature-resistant transformants have wild-type enzyme levels, whereas partial revertants to temperature resistance have varied levels of enzyme activity. The attachment and exchange activities of the lysS2 enzyme are more heat labile in vitro than the wild-type enzyme, as is the attachment activity of a partial revertant of the lysS1 mutant. The lysS1 and the lysS2 lysyl-tRNA synthetases have higher apparent Km values for lysine and ATP, in both the activation and the attachment reactions. The lysS2 enzyme has a Vmax for tRNAlys one-third that of the wild-type enzyme. Molecular weights of ∼150,000 for the wild-type and lysS2 enzymes and ∼76,000 for the lysS1 enzyme were estimated from sedimentation positions in sucrose density gradients assayed by the ATP-pyrophosphate exchange activity. We propose that the two mutations (lysS1 and lysS2) directly affect the sites for exchange activity, but indirectly alter attachment activity as a consequence of defective subunit association.
Full text
PDF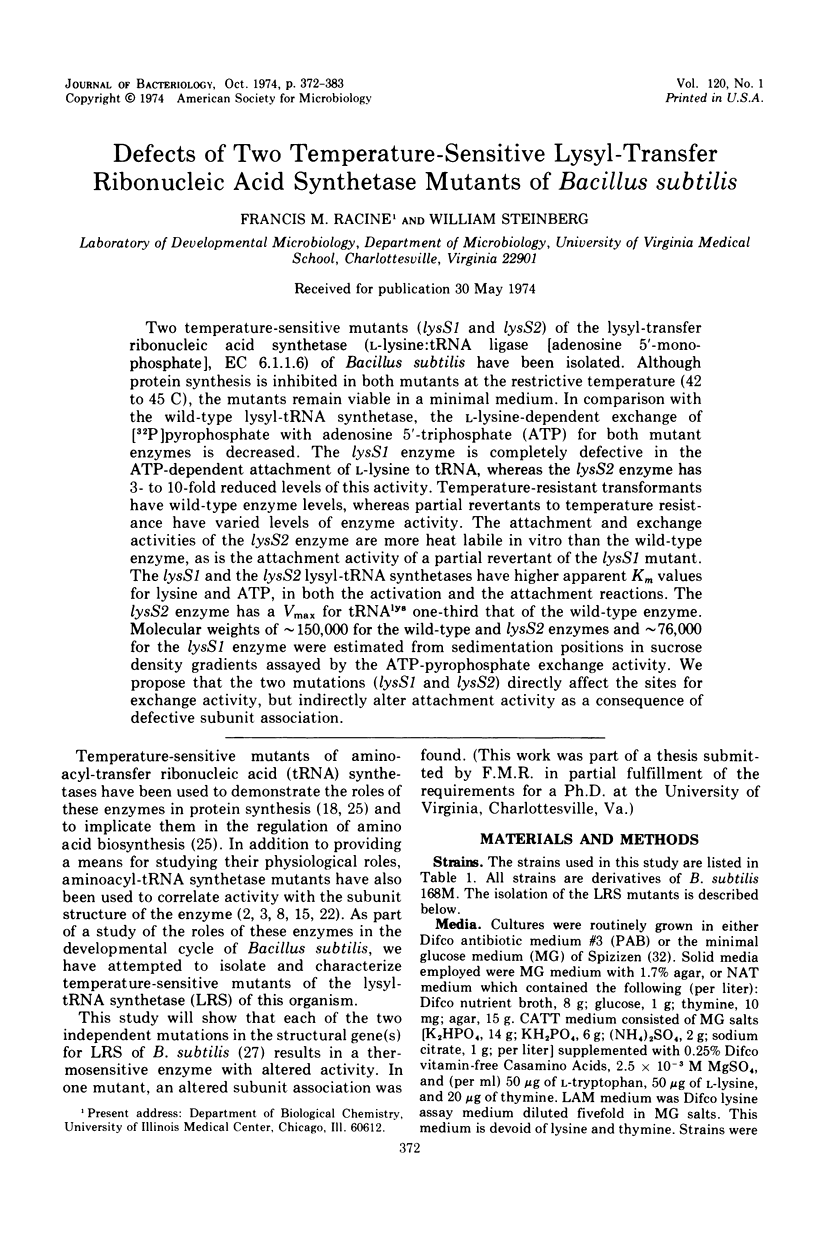
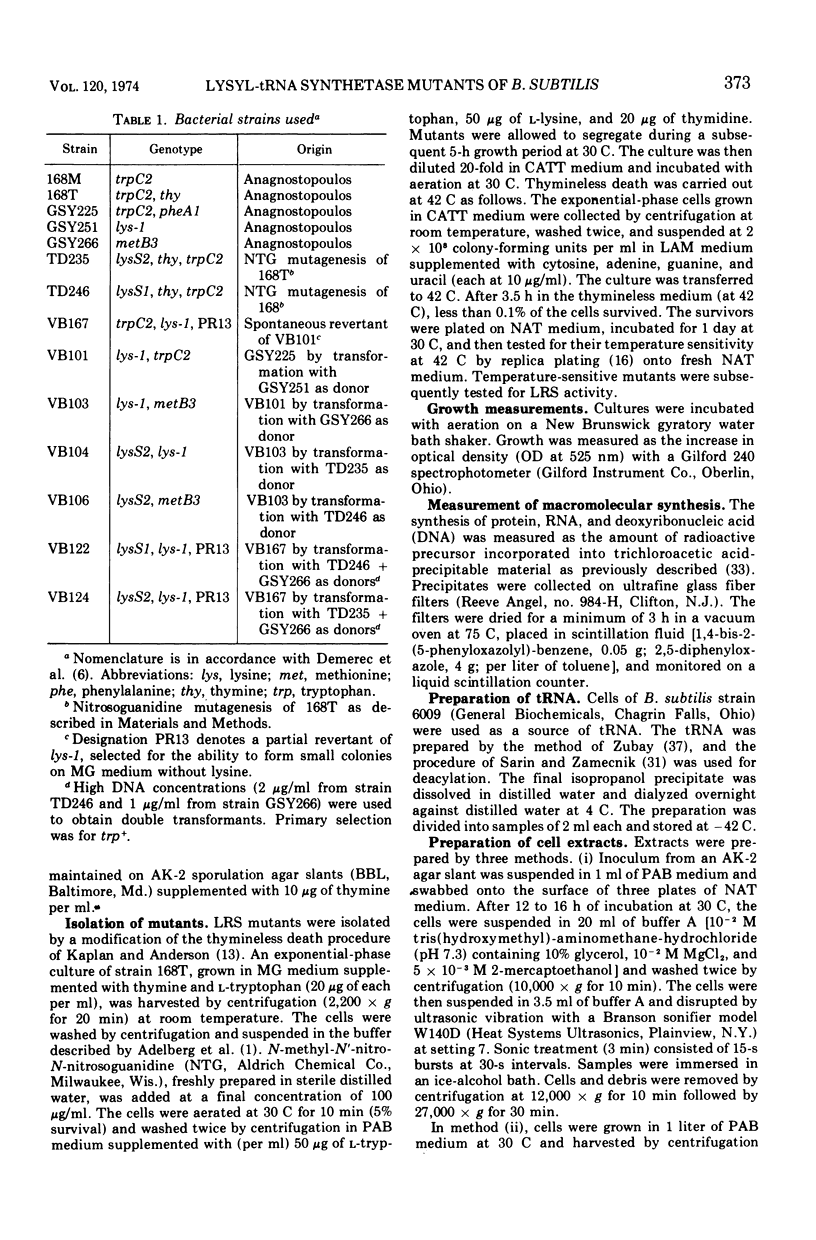
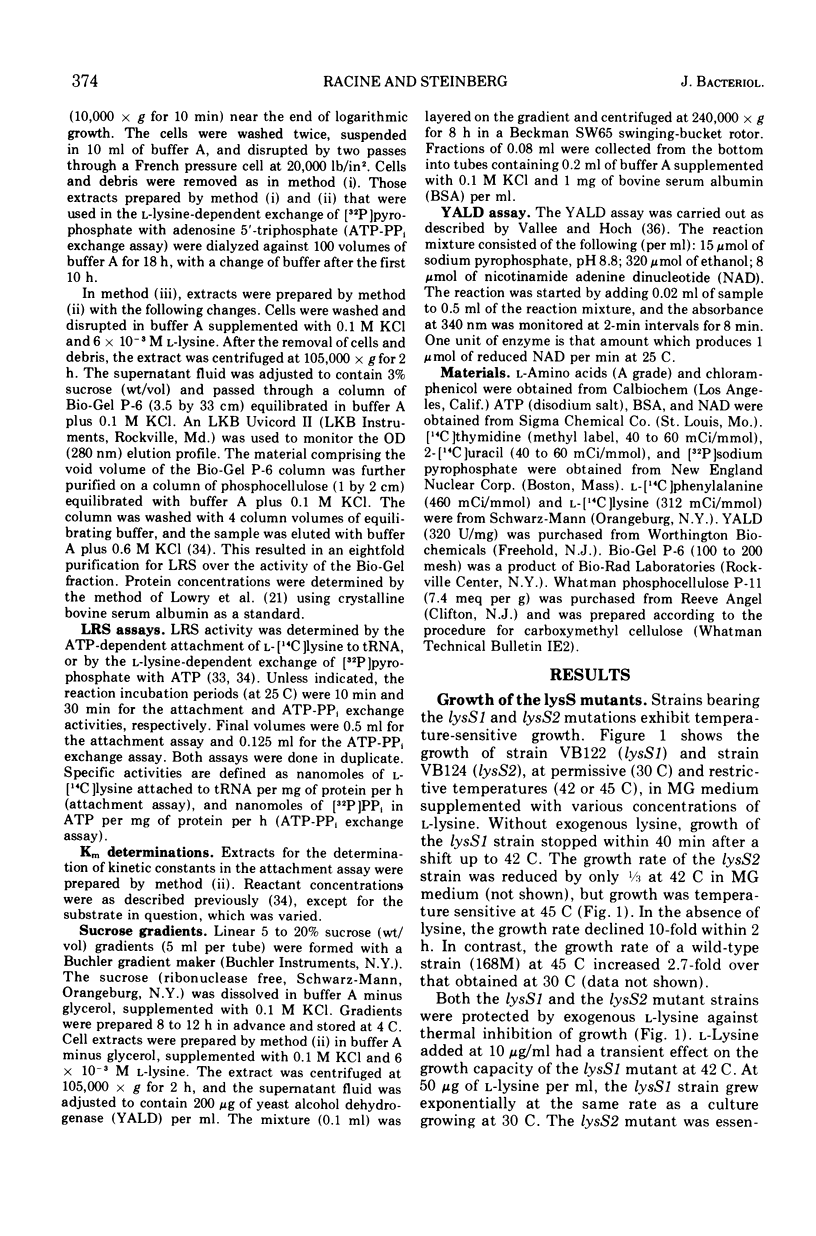
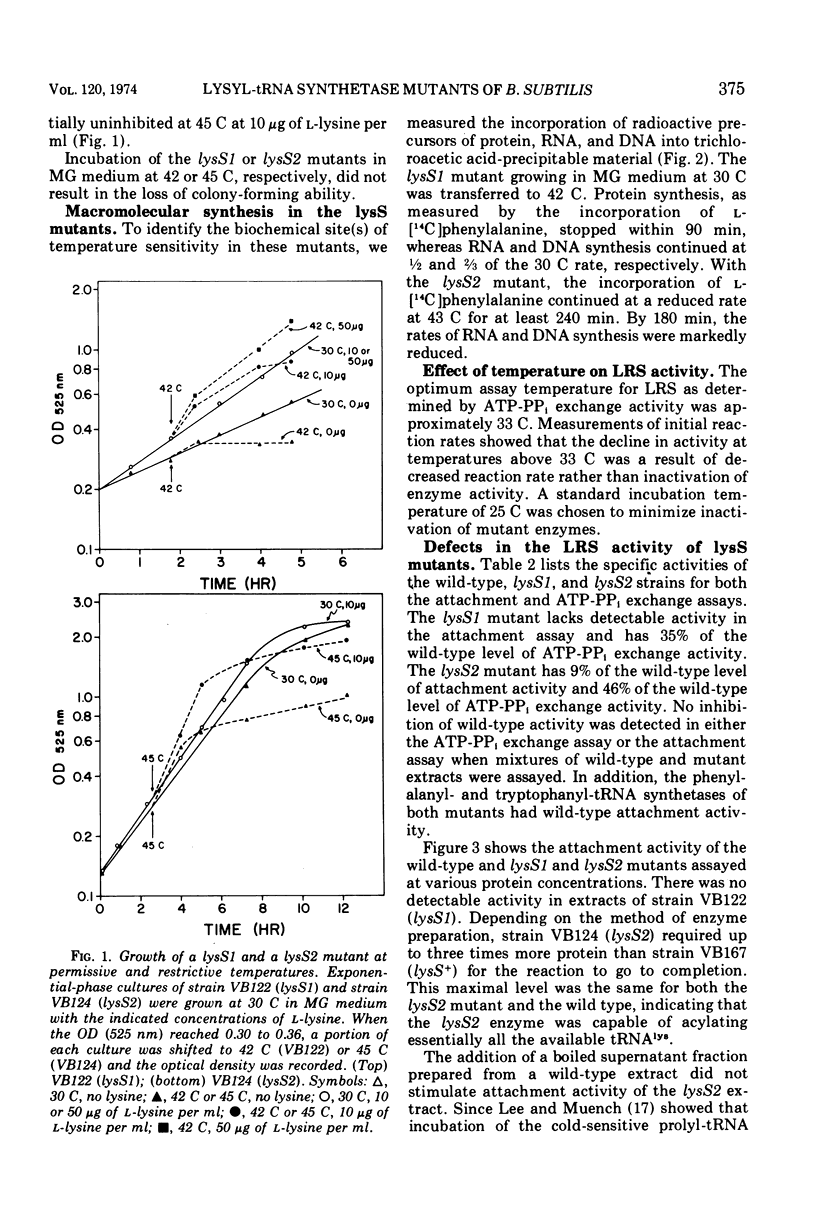
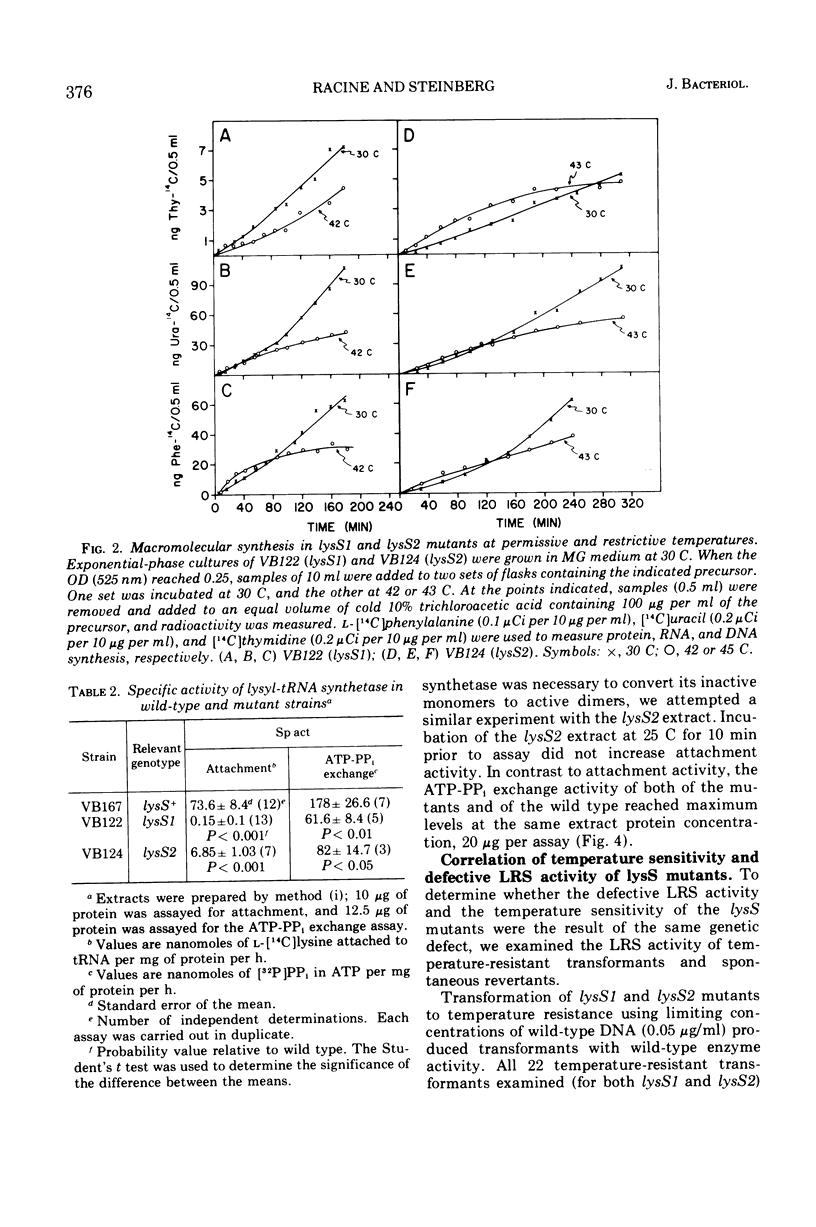
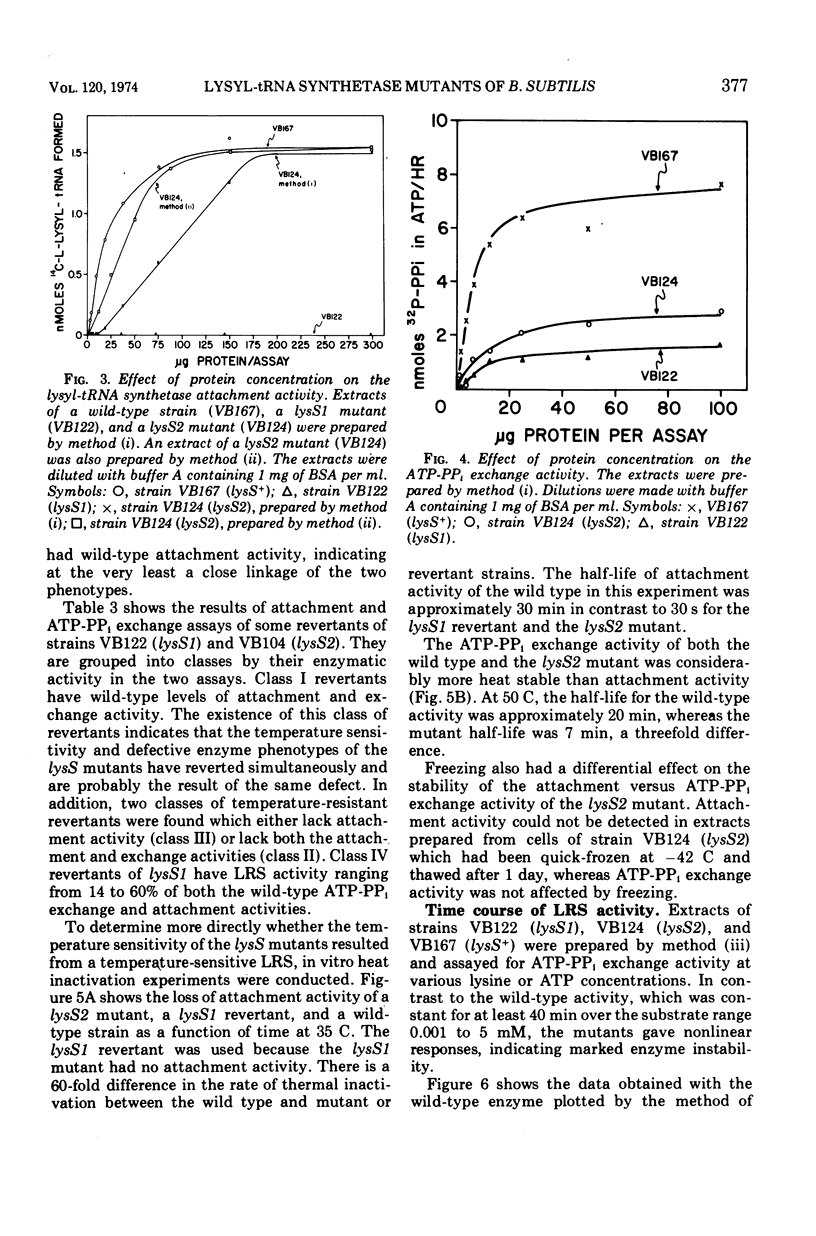
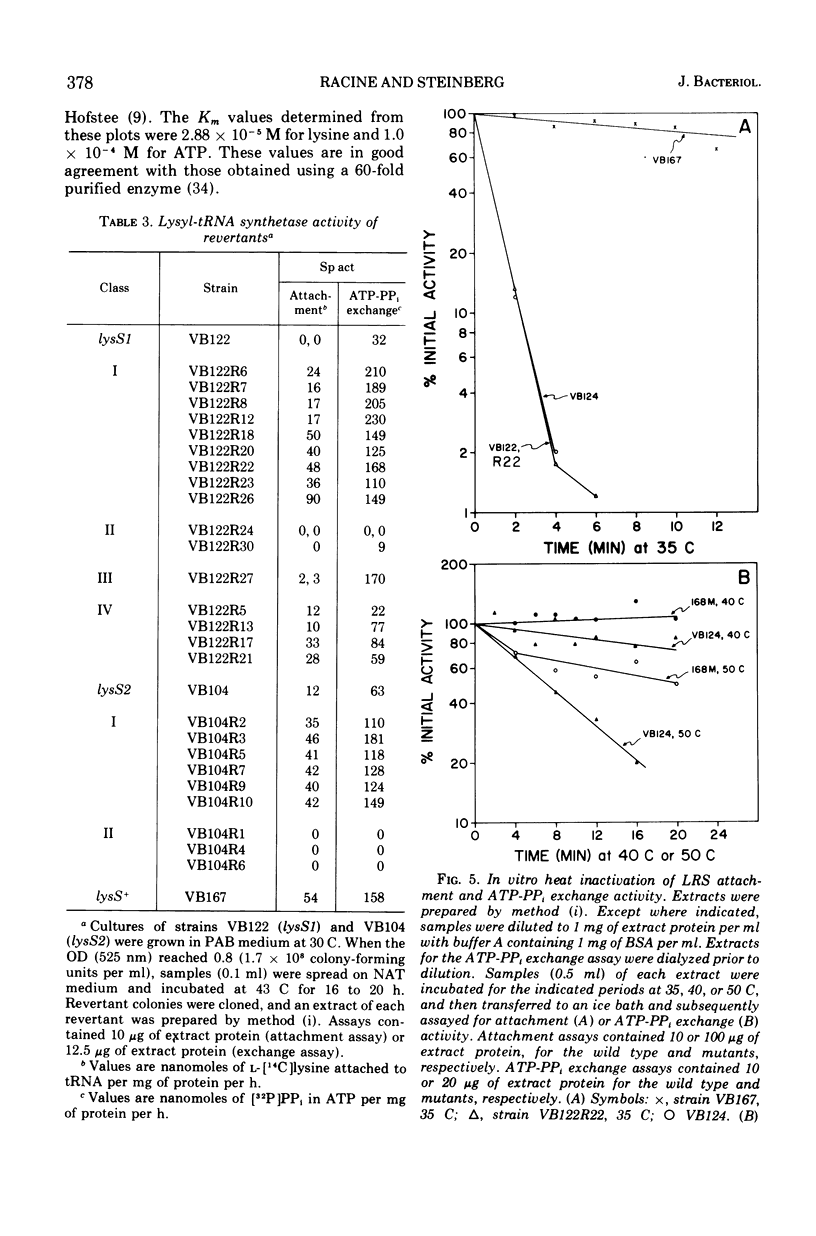
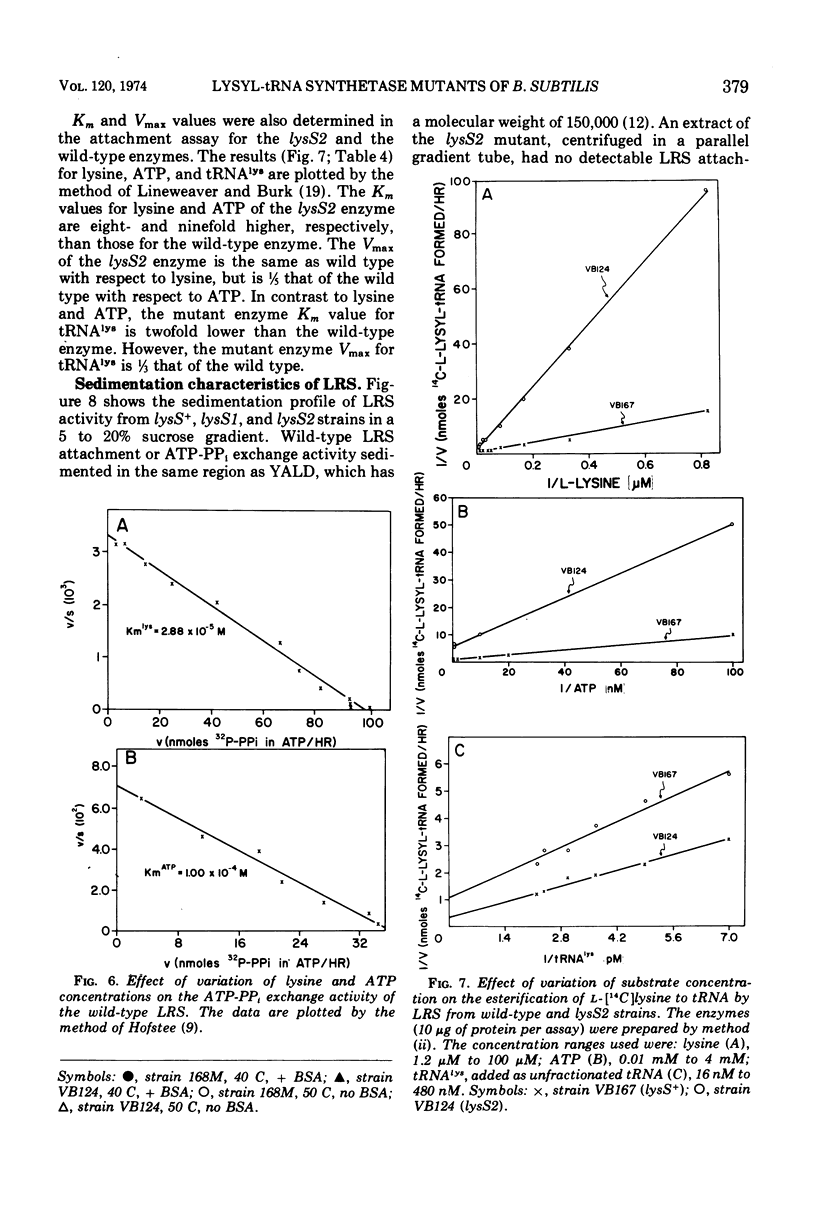
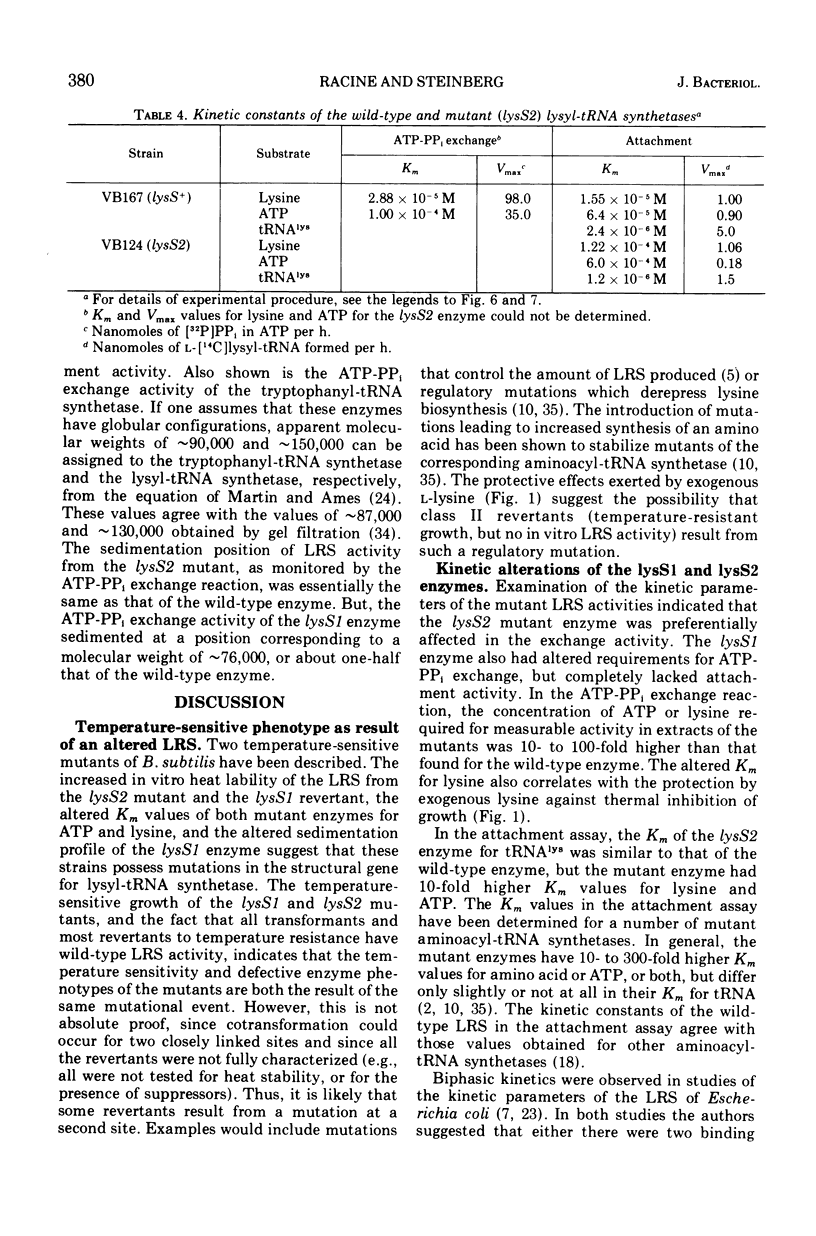
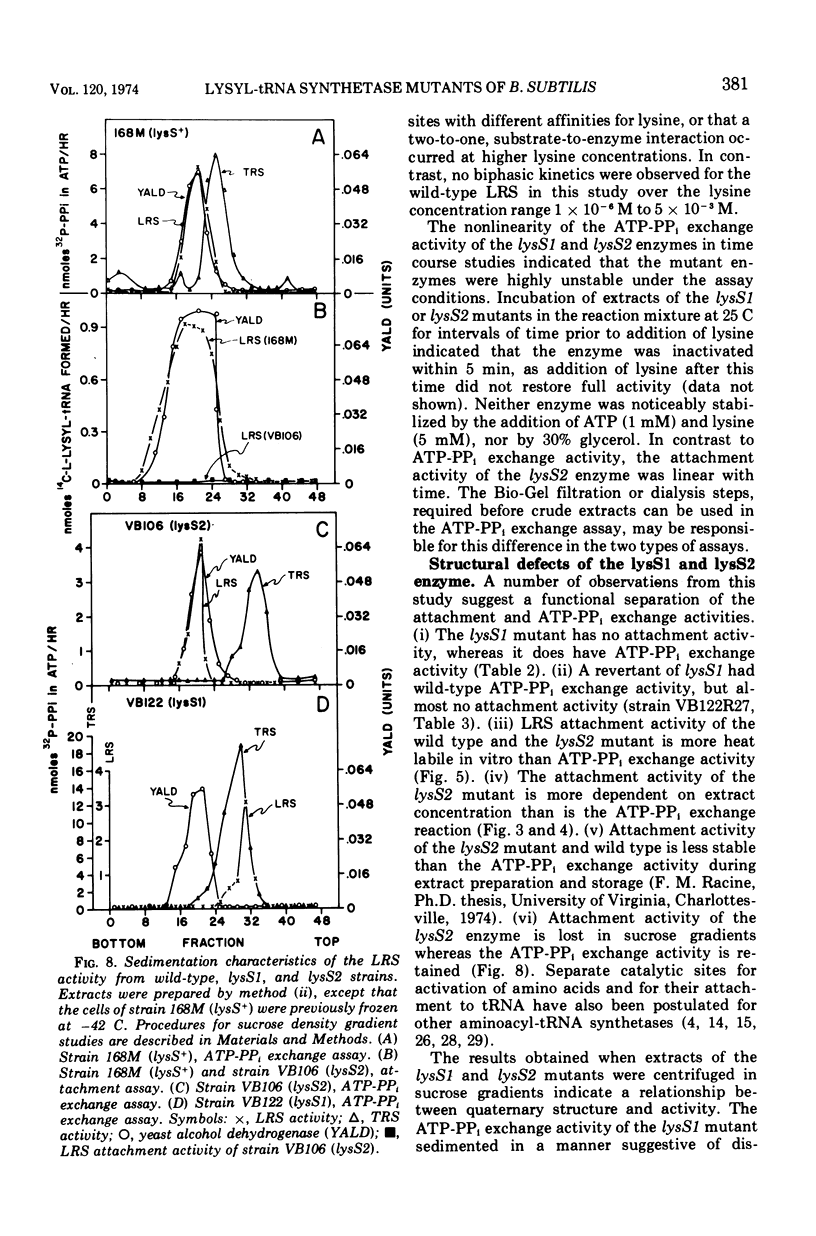
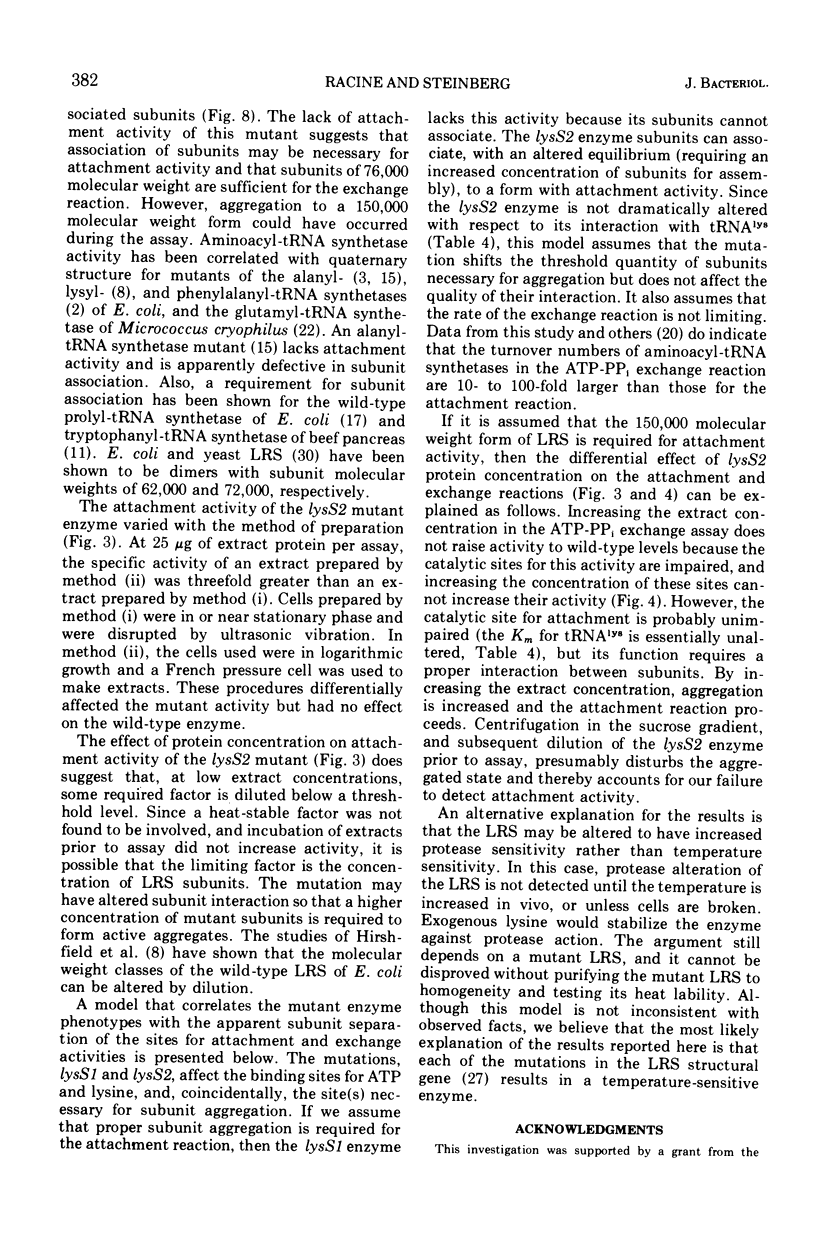
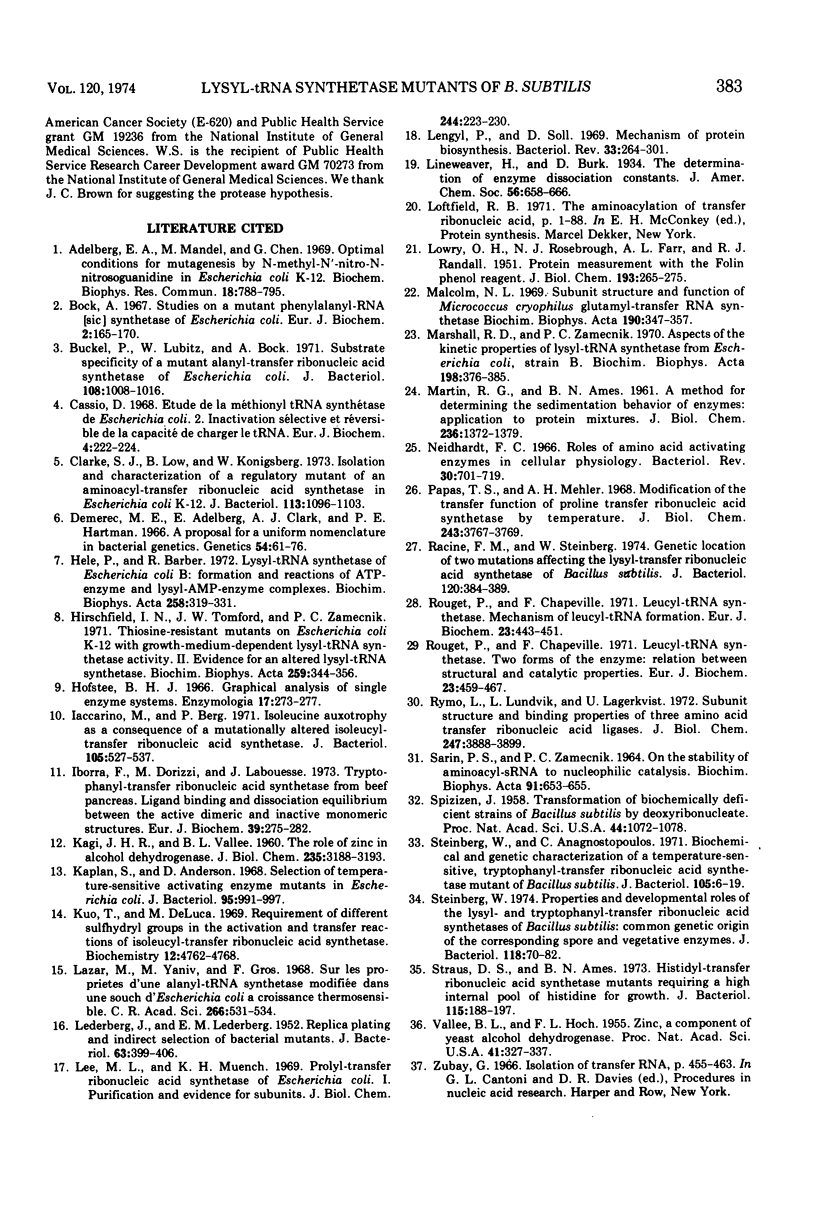
Selected References
These references are in PubMed. This may not be the complete list of references from this article.
- Buckel P., Lubitz W., Böck A. Substrate specificity of a mutant alanyl-transfer ribonucleic acid synthetase of Escherichia coli. J Bacteriol. 1971 Dec;108(3):1008–1016. doi: 10.1128/jb.108.3.1008-1016.1971. [DOI] [PMC free article] [PubMed] [Google Scholar]
- Böck A. Studies on mutant phenylalanyl RNA synthetases of Escherichia coli. Eur J Biochem. 1967 Sep;2(2):165–170. doi: 10.1111/j.1432-1033.1967.tb00121.x. [DOI] [PubMed] [Google Scholar]
- Cassio D. Etude de la méthionyl tRNA synthétase de Escherichia coli. 2. Inactivation sélective et réversible de la capacité de charger le tRNA. Eur J Biochem. 1968 Apr 3;4(2):222–224. doi: 10.1111/j.1432-1033.1968.tb00197.x. [DOI] [PubMed] [Google Scholar]
- Clarke S. J., Low B., Konigsberg W. Isolation and characterization of a regulatory mutant of an aminoacyl-transfer ribonucleic acid synthetase in Escherichia coli K-12. J Bacteriol. 1973 Mar;113(3):1096–1103. doi: 10.1128/jb.113.3.1096-1103.1973. [DOI] [PMC free article] [PubMed] [Google Scholar]
- Demerec M., Adelberg E. A., Clark A. J., Hartman P. E. A proposal for a uniform nomenclature in bacterial genetics. Genetics. 1966 Jul;54(1):61–76. doi: 10.1093/genetics/54.1.61. [DOI] [PMC free article] [PubMed] [Google Scholar]
- HOFSTEE B. H. Graphical analysis of single enzyme systems. Enzymologia. 1956 Jul 15;17(5-6):273–278. [PubMed] [Google Scholar]
- Hele P., Barber R. Lysyl tRNA synthetase of Escherichia coli B: formation and reactions of ATP-enzyme and lysyl-AMP-enzyme complexes. Biochim Biophys Acta. 1972 Jan 20;258(1):319–331. doi: 10.1016/0005-2744(72)90989-8. [DOI] [PubMed] [Google Scholar]
- Hirshfield I. N., Tomford J. W., Zamecnik P. C. Thiosine-resistant mutants of Escherichia coli K-12 with growth-medium-dependent lysyl-tRNA synthetase activity.II. Evidence for an altered lysyl-tRNA synthetase. Biochim Biophys Acta. 1972 Feb 15;259(3):344–356. doi: 10.1016/0005-2787(72)90309-7. [DOI] [PubMed] [Google Scholar]
- Iaccarino M., Berg P. Isoleucine auxotrophy as a consequence of a mutationally altered isoleucyl-transfer ribonucleic acid synthetase. J Bacteriol. 1971 Feb;105(2):527–537. doi: 10.1128/jb.105.2.527-537.1971. [DOI] [PMC free article] [PubMed] [Google Scholar]
- Iborra F., Dorizzi M., Labouesse J. Tryptophanyl-transfer ribonucleic-acid synthetase from beef pancreas. Ligand binding and dissociation equilibrium between the active dimeric and inactive monomeric structures. Eur J Biochem. 1973 Nov 1;39(1):275–282. doi: 10.1111/j.1432-1033.1973.tb03124.x. [DOI] [PubMed] [Google Scholar]
- KAGI J. H., VALLEE B. L. The role of zinc in alcohol dehydrogenase. V. The effect of metal-binding agents on thestructure of the yeast alcohol dehydrogenase molecule. J Biol Chem. 1960 Nov;235:3188–3192. [PubMed] [Google Scholar]
- Kaplan S., Anderson D. Selection of temperature-sensitive activating enzyme mutants in Escherichia coli. J Bacteriol. 1968 Mar;95(3):991–997. doi: 10.1128/jb.95.3.991-997.1968. [DOI] [PMC free article] [PubMed] [Google Scholar]
- Kuo T., DeLuca M. Requirement of different sulfhydryl groups in the activation and transfer reactions of isoleucyl transfer ribonucleic acid synthetase. Biochemistry. 1969 Dec;8(12):4762–4768. doi: 10.1021/bi00840a017. [DOI] [PubMed] [Google Scholar]
- LEDERBERG J., LEDERBERG E. M. Replica plating and indirect selection of bacterial mutants. J Bacteriol. 1952 Mar;63(3):399–406. doi: 10.1128/jb.63.3.399-406.1952. [DOI] [PMC free article] [PubMed] [Google Scholar]
- LOWRY O. H., ROSEBROUGH N. J., FARR A. L., RANDALL R. J. Protein measurement with the Folin phenol reagent. J Biol Chem. 1951 Nov;193(1):265–275. [PubMed] [Google Scholar]
- Lazar M., Yaniv M., Gros F. Sur les propriétés d'une alanyl-t-RNA synthétase modifiée dans une souche d'Escherichia coli à croissance thermosensible. C R Acad Sci Hebd Seances Acad Sci D. 1968 Jan 29;266(5):531–534. [PubMed] [Google Scholar]
- Lee M. L., Muench K. H. Prolyl transfer ribonucleic acid synthetase of Escherichia coli. I. Purification and evidence for subunits. J Biol Chem. 1969 Jan 25;244(2):223–230. [PubMed] [Google Scholar]
- Lengyel P., Söll D. Mechanism of protein biosynthesis. Bacteriol Rev. 1969 Jun;33(2):264–301. doi: 10.1128/br.33.2.264-301.1969. [DOI] [PMC free article] [PubMed] [Google Scholar]
- MARTIN R. G., AMES B. N. A method for determining the sedimentation behavior of enzymes: application to protein mixtures. J Biol Chem. 1961 May;236:1372–1379. [PubMed] [Google Scholar]
- Malcolm N. L. Subunit structure and function of Micrococcus cryophilus glutamyl transfer RNA synthetase. Biochim Biophys Acta. 1969 Oct 22;190(2):347–357. doi: 10.1016/0005-2787(69)90085-9. [DOI] [PubMed] [Google Scholar]
- Marshall R. D., Zamecnik P. C. Aspects of the kinetic properties of lysyl-tRNA synthetase from Escherichia coli, strain B. Biochim Biophys Acta. 1970 Feb 11;198(2):376–385. doi: 10.1016/0005-2744(70)90070-7. [DOI] [PubMed] [Google Scholar]
- Neidhardt F. C. Roles of amino acid activating enzymes in cellular physiology. Bacteriol Rev. 1966 Dec;30(4):701–719. doi: 10.1128/br.30.4.701-719.1966. [DOI] [PMC free article] [PubMed] [Google Scholar]
- Papas T. S., Mehler A. H. Modification of the transfer function of proline transfer ribonucleic acid synthetase by temperature. J Biol Chem. 1968 Jul 10;243(13):3767–3769. [PubMed] [Google Scholar]
- Racine F. M., Steinberg W. Genetic location of two mutations affecting the lysyl-transfer ribonucleic acid synthetase of Bacillus subtilis. J Bacteriol. 1974 Oct;120(1):384–389. doi: 10.1128/jb.120.1.384-389.1974. [DOI] [PMC free article] [PubMed] [Google Scholar]
- Rouget P., Chapeville F. Leucyl-tRNA synthetase. Mechanism of leucyl-tRNA formation. Eur J Biochem. 1971 Dec 10;23(3):443–451. doi: 10.1111/j.1432-1033.1971.tb01639.x. [DOI] [PubMed] [Google Scholar]
- Rouget P., Chapeville F. Leucyl-tRNA synthetase. Two forms of the enzyme: relation between structural and catalytic properties. Eur J Biochem. 1971 Dec 10;23(3):459–467. doi: 10.1111/j.1432-1033.1971.tb01641.x. [DOI] [PubMed] [Google Scholar]
- Rymo L., Lundvik L., Lagerkvist U. Subunit structure and binding properties of three amino acid transfer ribonucleic acid ligases. J Biol Chem. 1972 Jun 25;247(12):3888–3897. [PubMed] [Google Scholar]
- SARIN P. S., ZAMECNIK P. C. ON THE STABILITY OF AMINOACYL-S-RNA TO NUCLEOPHILIC CATALYSIS. Biochim Biophys Acta. 1964 Dec 16;91:653–655. doi: 10.1016/0926-6550(64)90018-0. [DOI] [PubMed] [Google Scholar]
- Spizizen J. TRANSFORMATION OF BIOCHEMICALLY DEFICIENT STRAINS OF BACILLUS SUBTILIS BY DEOXYRIBONUCLEATE. Proc Natl Acad Sci U S A. 1958 Oct 15;44(10):1072–1078. doi: 10.1073/pnas.44.10.1072. [DOI] [PMC free article] [PubMed] [Google Scholar]
- Steinberg W., Anagnostopoulos C. Biochemical and genetic characterization of a temperature-sensitive, tryptophanyl-transfer ribonucleic acid synthetase mutant of Bacillus subtilis. J Bacteriol. 1971 Jan;105(1):6–19. doi: 10.1128/jb.105.1.6-19.1971. [DOI] [PMC free article] [PubMed] [Google Scholar]
- Steinberg W. Properties and developmental roles of the lysyl- and tryptophanyl-transfer ribonucleic acid synthetases of Bacillus subtilis: common genetic origin of the corresponding spore and vegetative enzymes. J Bacteriol. 1974 Apr;118(1):70–82. doi: 10.1128/jb.118.1.70-82.1974. [DOI] [PMC free article] [PubMed] [Google Scholar]
- Straus D. S., Ames B. N. Histidyl-transfer ribonucleic acid synthetase mutants requiring a high internal pool of histidine for growth. J Bacteriol. 1973 Jul;115(1):188–197. doi: 10.1128/jb.115.1.188-197.1973. [DOI] [PMC free article] [PubMed] [Google Scholar]
- Vallee B. L., Hoch F. L. ZINC, A COMPONENT OF YEAST ALCOHOL DEHYDROGENASE. Proc Natl Acad Sci U S A. 1955 Jun 15;41(6):327–338. doi: 10.1073/pnas.41.6.327. [DOI] [PMC free article] [PubMed] [Google Scholar]