Abstract
The growth of thermosensitive Bacillus subtilis lysyl- and tryptophanyl-transfer ribonucleic acid synthetase mutants (lysS1 and trypS1) (l-lysine:transfer ribonucleic acid [tRNA] ligase [AMP], EC 6.1.1.6; and l-tryptophan:tRNA ligase [AMP], EC 6.1.1.2) was terminated when exponential phase cells were shifted from 30 to 43 C in a rich medium. Under these conditions, the temperature-inhibited cells undergo thermal death; they rapidly lose their ability to form colonies at 30 C. Another lysyl-tRNA synthetase mutant (lysS2) is refractory to thermal death even though its growth is inhibited at 43 C. The thermal death response of the lysS1 mutant is affected by the stage of cell development. At periods in spore outgrowth and sporogenesis these cells become refractory to thermal death. The refractory state does not result from the production of an inhibitor, or from the degradation of an activator of thermal death. However, culture medium composition does modify the thermal death response. Rich media enhance the effect, and no thermal death occurs in the lysS1 strain grown in a minimal medium. Temperature-sensitive cells can grow in a lysine- (0.25 mM) or tryptophan- (0.25 mM) supplemented minimal medium at 43 C, but amino acid concentrations of 25 mM only transiently protect trypS1 and lysS1 cells from thermal death in a rich medium. Osmotic agents such as sucrose (0.5 M) and NaCl (0.34 M) completely prevent thermal death in the lysS1 strain, although growth is still arrested. On solid media, sucrose stabilized lysS1 cells can form colonies at the restrictive temperature, but neither sucrose (0.5 M) nor NaCl (0.34 M) stabilized the lysS1 enzyme in vitro. Chloramiphenicol increased the rate of thermal death of the lysS1 strain but decreased the thermal death response of the trypS1 mutant. Considering the nature of the enzyme defect in the lysS1 strain, the common genetic origin of the spore and vegetative lysyl-tRNA synthetase, and the protective effects exerted by lysine and osmotic agents, it is tentatively concluded that thermal death results from irreversible inactivation of the mutant gene product. According to this hypothesis, either the lysS1 enzyme is altered during sporogenesis or some physiological or structural aspect of this developmental phase can stabilize the mutant phenotype and thereby rescue cells from thermal death.
Full text
PDF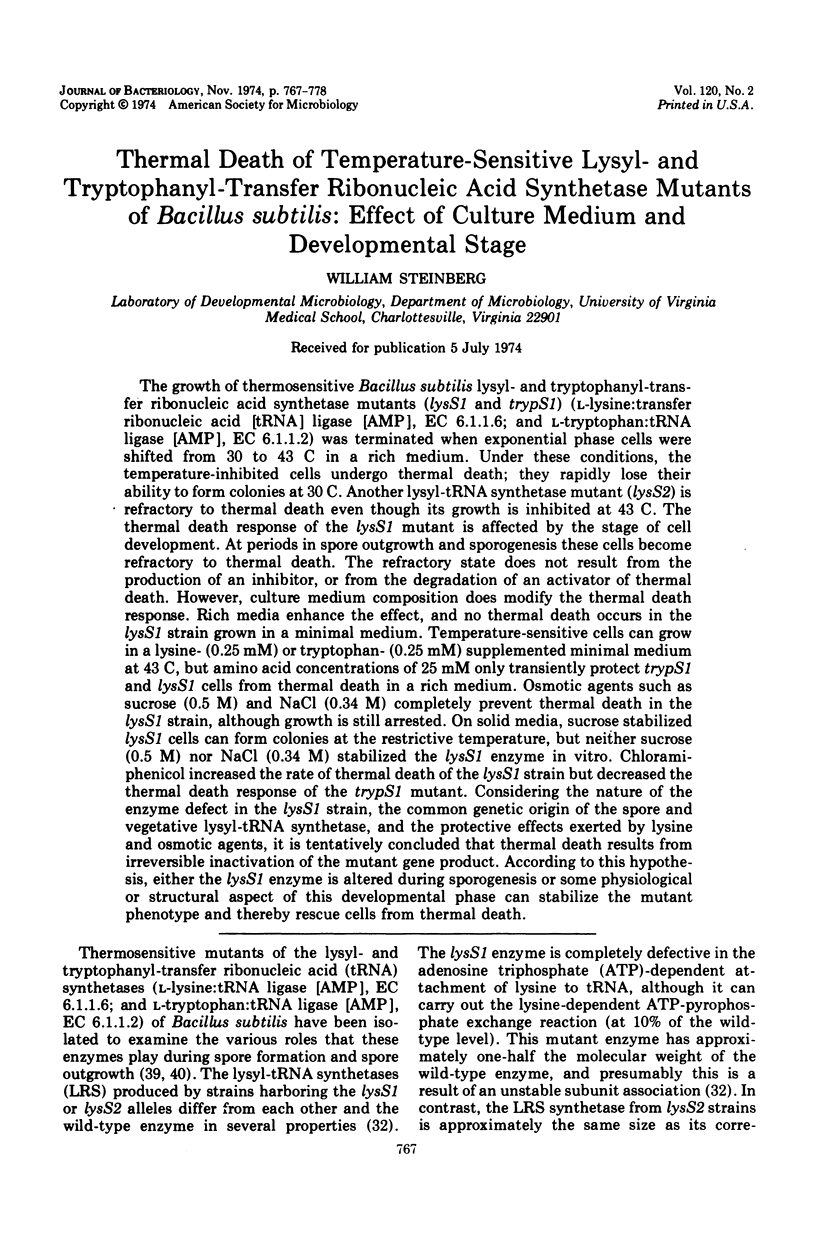
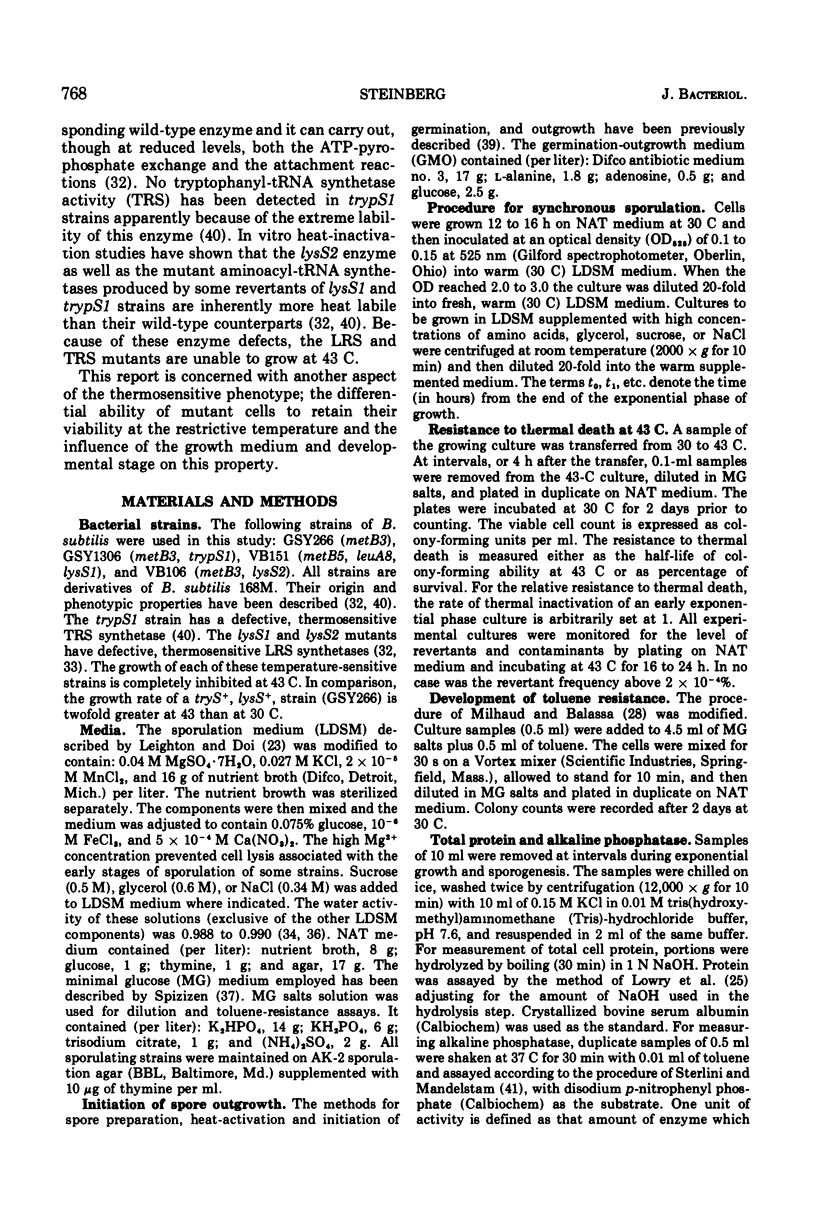
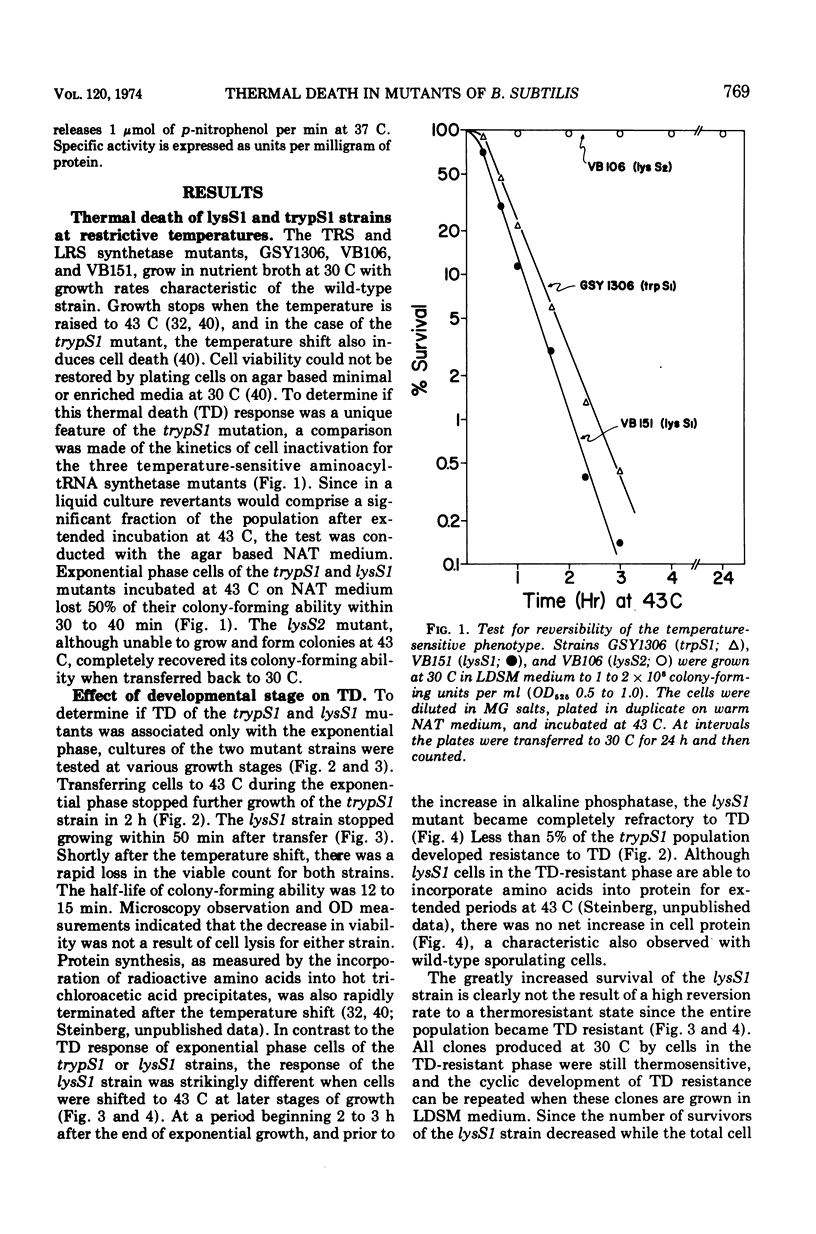
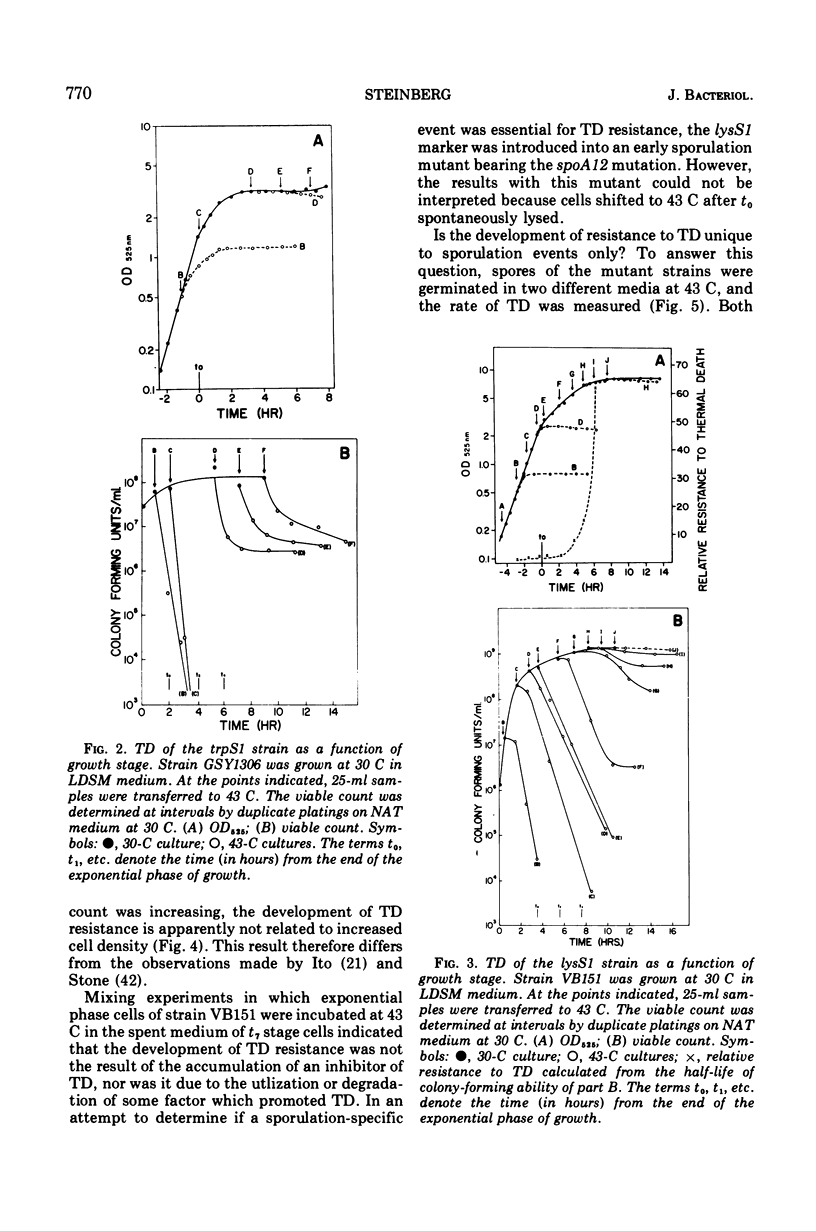
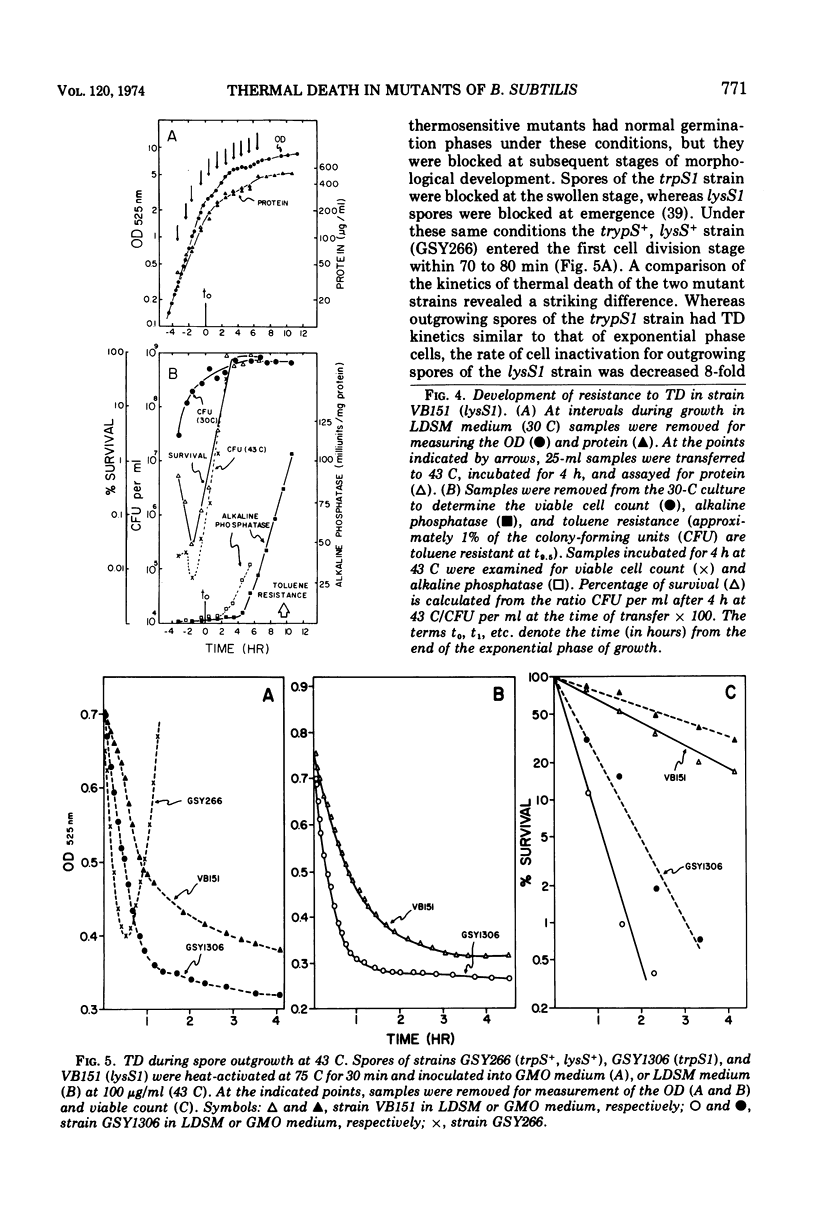
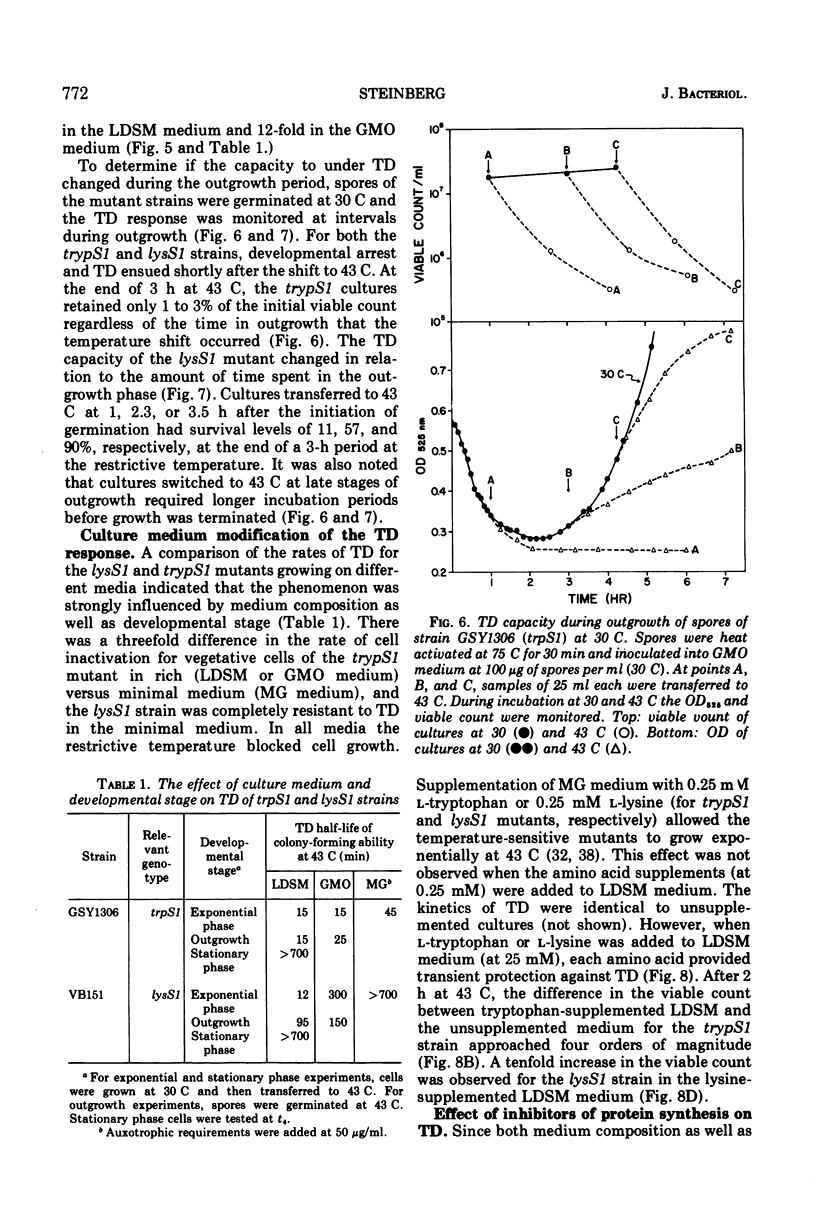
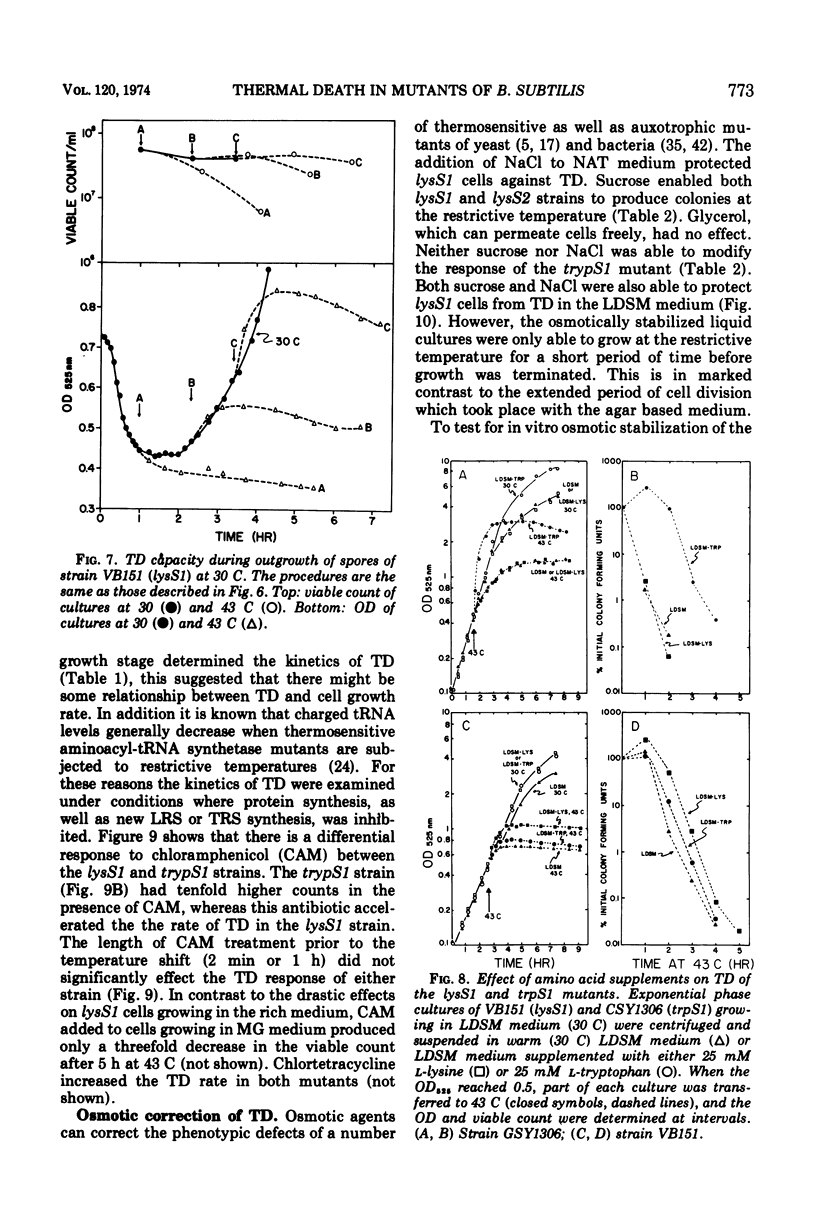
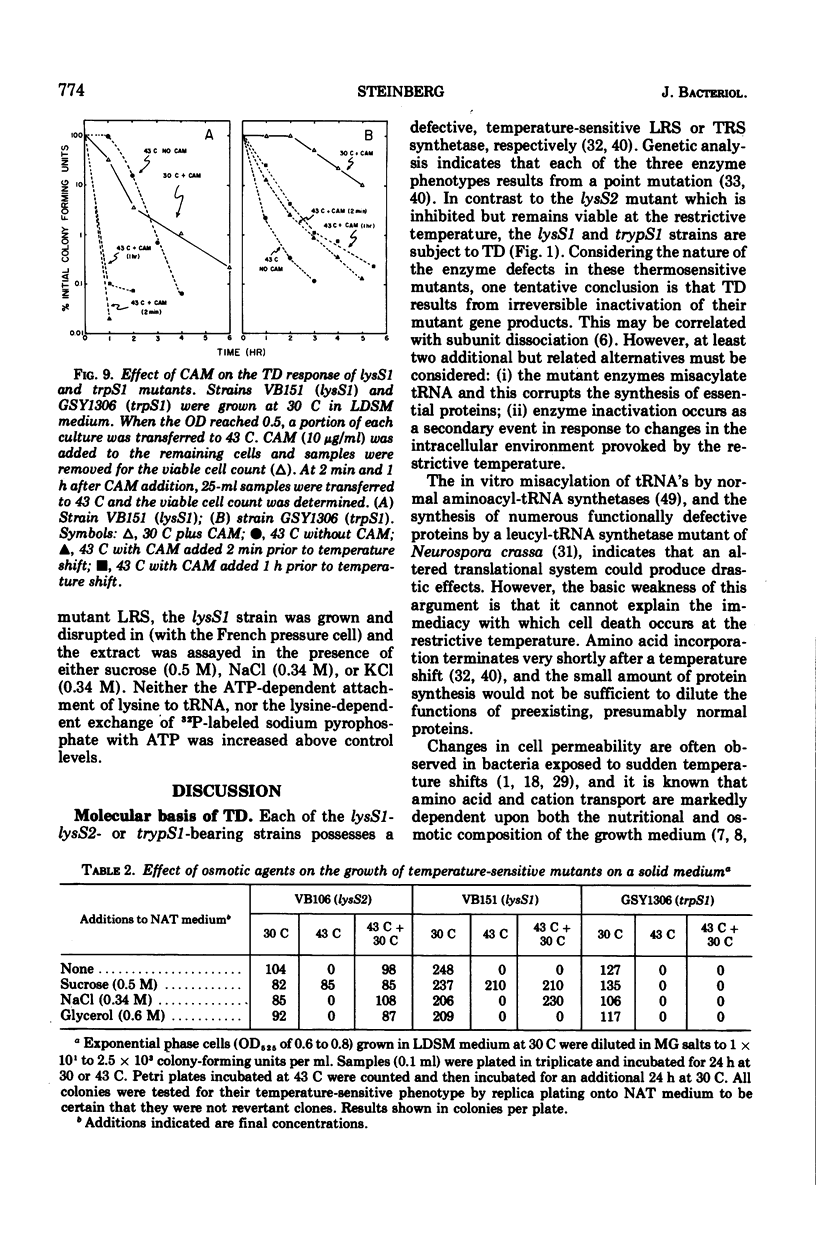
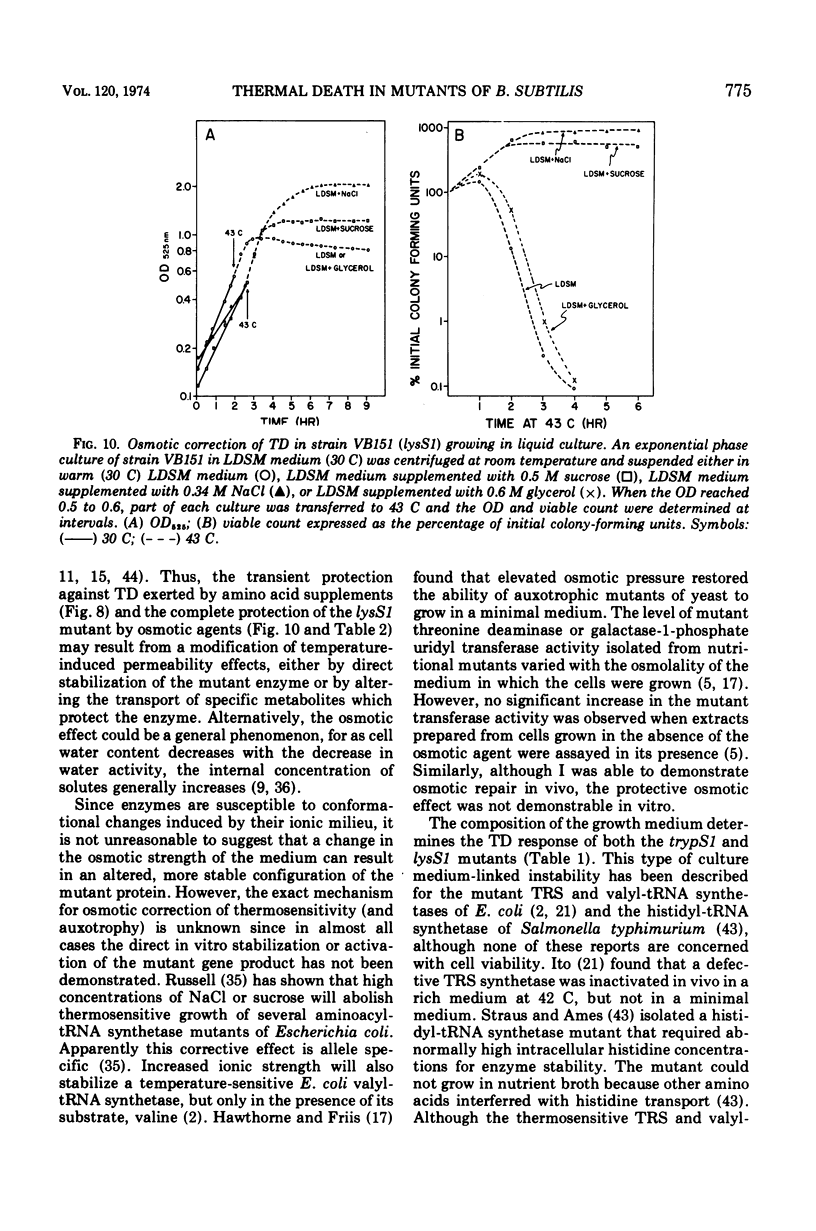
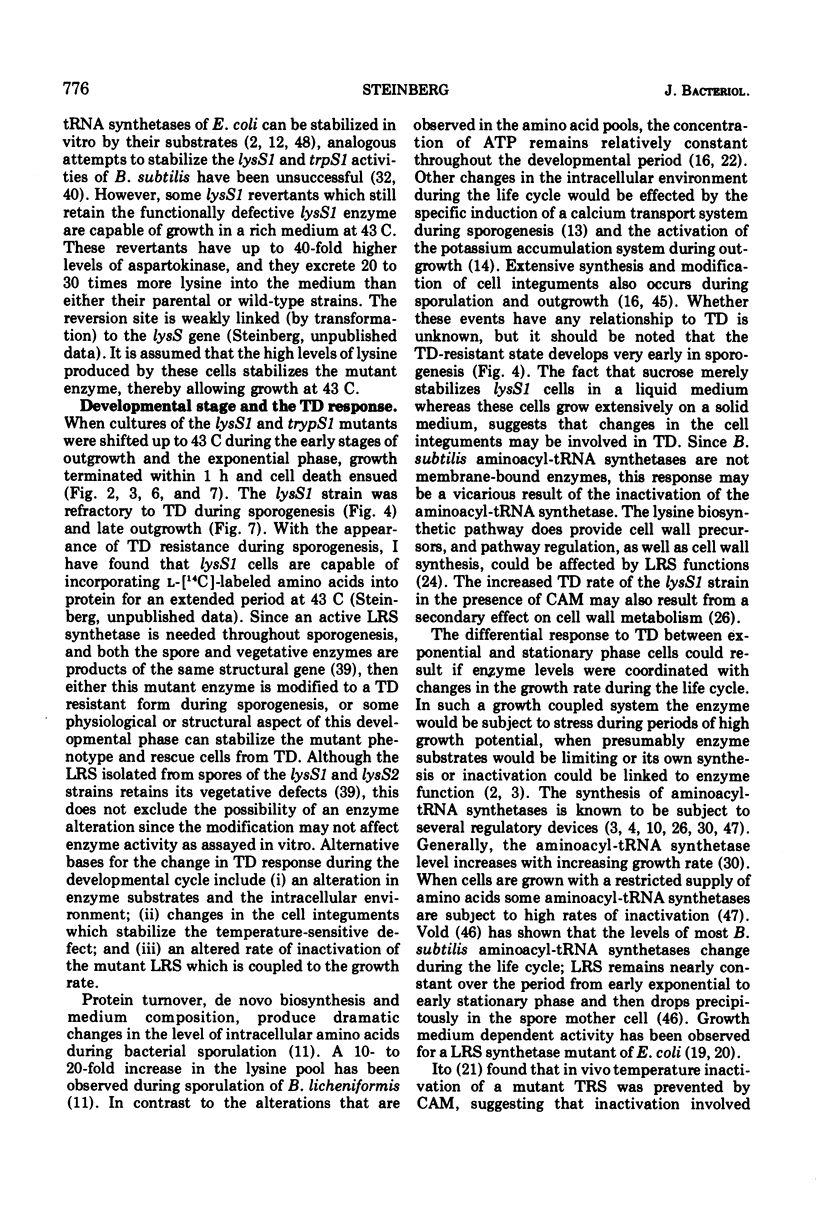
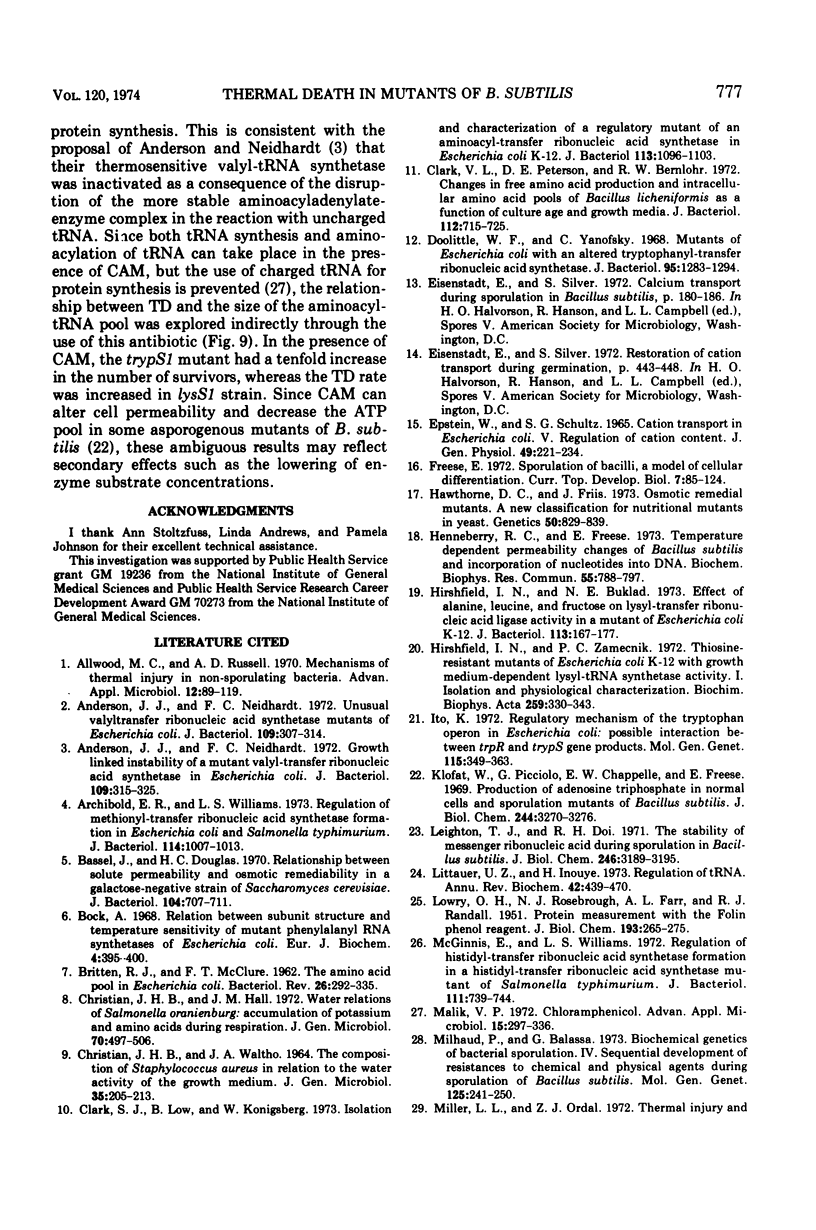
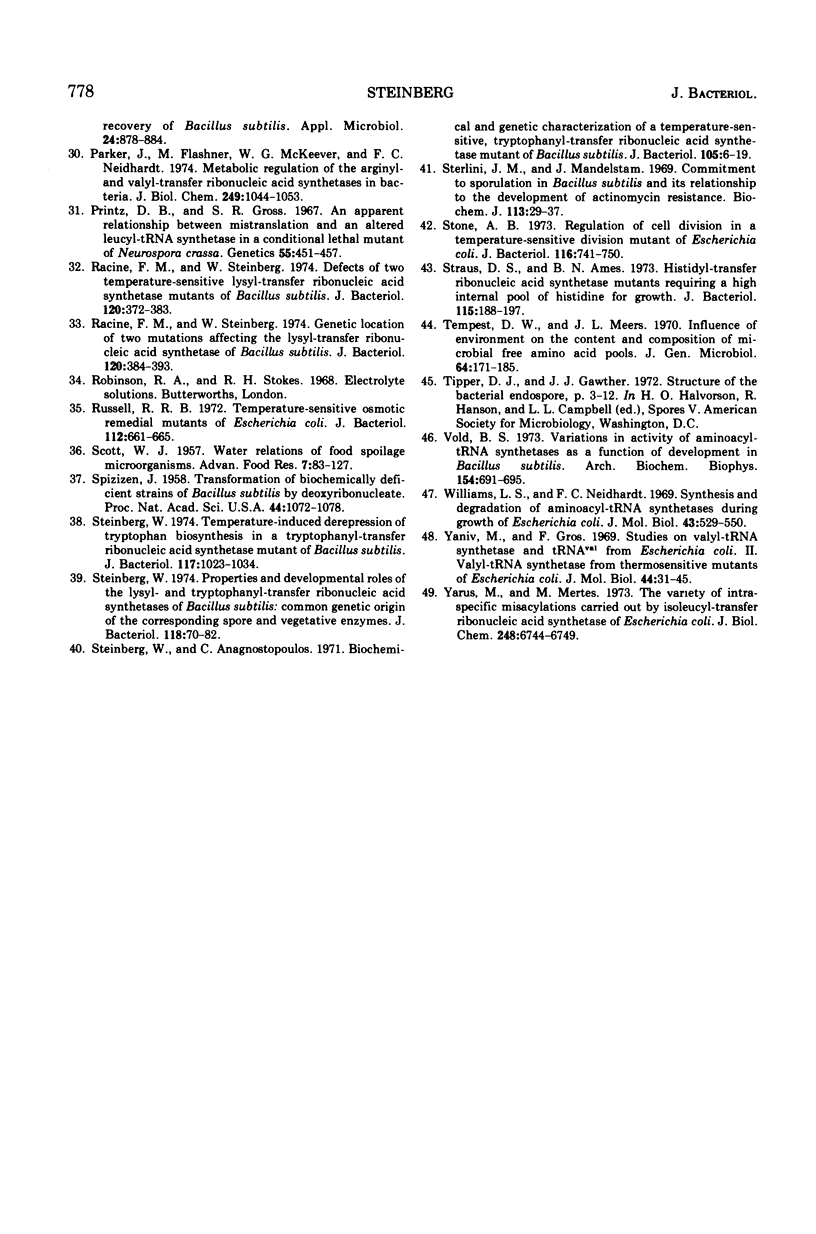
Selected References
These references are in PubMed. This may not be the complete list of references from this article.
- Allwood M. C., Russell A. D. Mechanisms of thermal injury in nonsporulating bacteria. Adv Appl Microbiol. 1970;12:89–119. doi: 10.1016/s0065-2164(08)70583-5. [DOI] [PubMed] [Google Scholar]
- Anderson J. J., Neidhardt F. C. Growth-linked instability of a mutant valyl-transfer ribonucleic acid synthetase in Escherichia coli. J Bacteriol. 1972 Jan;109(1):315–325. doi: 10.1128/jb.109.1.315-325.1972. [DOI] [PMC free article] [PubMed] [Google Scholar]
- Anderson J. J., Neidhardt F. C. Unusual valyl-transfer ribonucleic acid synthetase mutant of Escherichia coli. J Bacteriol. 1972 Jan;109(1):307–314. doi: 10.1128/jb.109.1.307-314.1972. [DOI] [PMC free article] [PubMed] [Google Scholar]
- Archibold E. R., Williams L. S. Regulation of methionyl-transfer ribonucleic acid synthetase formation in Escherichia coli and Salmonella typhimurium. J Bacteriol. 1973 Jun;114(3):1007–1013. doi: 10.1128/jb.114.3.1007-1013.1973. [DOI] [PMC free article] [PubMed] [Google Scholar]
- BRITTEN R. J., McCLURE F. T. The amino acid pool in Escherichia coli. Bacteriol Rev. 1962 Sep;26:292–335. doi: 10.1128/br.26.3.292-335.1962. [DOI] [PMC free article] [PubMed] [Google Scholar]
- Bassel J., Douglas H. C. Relationship between solute permeability and osmotic remediability in a galactose-negative strain of Saccharomyces cerevisiae. J Bacteriol. 1970 Nov;104(2):707–711. doi: 10.1128/jb.104.2.707-711.1970. [DOI] [PMC free article] [PubMed] [Google Scholar]
- Böck A. Relation between subunit structure and temperature-sensitivity of mutant phenylalanyl RNA synthetases of Escherichia coli. Eur J Biochem. 1968 Apr;4(3):395–400. doi: 10.1111/j.1432-1033.1968.tb00225.x. [DOI] [PubMed] [Google Scholar]
- CHRISTIAN J. H., WALTHO J. A. THE COMPOSITION OF STAPHYLOCOCCUS AUREUS IN RELATION TO THE WATER ACTIVITY OF THE GROWTH MEDIUM. J Gen Microbiol. 1964 May;35:205–213. doi: 10.1099/00221287-35-2-205. [DOI] [PubMed] [Google Scholar]
- Christian J. H., Hall J. M. Water relations of Salmonella oranienburg: accumulation of potassium and amino acids during respiration. J Gen Microbiol. 1972 May;70(3):497–506. doi: 10.1099/00221287-70-3-497. [DOI] [PubMed] [Google Scholar]
- Clark V. L., Peterson D. E., Bernlohr R. W. Changes in free amino acid production and intracellular amino acid pools of Bacillus licheniformis as a function of culture age and growth media. J Bacteriol. 1972 Nov;112(2):715–725. doi: 10.1128/jb.112.2.715-725.1972. [DOI] [PMC free article] [PubMed] [Google Scholar]
- Clarke S. J., Low B., Konigsberg W. Isolation and characterization of a regulatory mutant of an aminoacyl-transfer ribonucleic acid synthetase in Escherichia coli K-12. J Bacteriol. 1973 Mar;113(3):1096–1103. doi: 10.1128/jb.113.3.1096-1103.1973. [DOI] [PMC free article] [PubMed] [Google Scholar]
- Doolittle W. F., Yanofsky C. Mutants of Escherichia coli with an altered tryptophanyl-transfer ribonucleic acid synthetase. J Bacteriol. 1968 Apr;95(4):1283–1294. doi: 10.1128/jb.95.4.1283-1294.1968. [DOI] [PMC free article] [PubMed] [Google Scholar]
- Freese E. Sporulation of bacilli, a model of cellular differentiation. Curr Top Dev Biol. 1972;7:85–124. doi: 10.1016/s0070-2153(08)60070-8. [DOI] [PubMed] [Google Scholar]
- HAWTHORNE D. C., FRIIS J. OSMOTIC-REMEDIAL MUTANTS. A NEW CLASSIFICATION FOR NUTRITIONAL MUTANTS IN YEAST. Genetics. 1964 Nov;50:829–839. doi: 10.1093/genetics/50.5.829. [DOI] [PMC free article] [PubMed] [Google Scholar]
- Henneberry R. C., Freese E. Temperature dependent permeability changes of Bacillus subtilis and incorporation of nucleotides into DNA. Biochem Biophys Res Commun. 1973 Dec 10;55(3):788–797. doi: 10.1016/0006-291x(73)91213-8. [DOI] [PubMed] [Google Scholar]
- Hirshfield I. N., Bukald N. E. Effect of alanine, leucine and fructose on lysyl-transfer ribonucleic acid ligase activity in a mutant of Escherichia coli K-12. J Bacteriol. 1973 Jan;113(1):167–177. doi: 10.1128/jb.113.1.167-177.1973. [DOI] [PMC free article] [PubMed] [Google Scholar]
- Hirshfield I. N., Zamecnik P. C. Thiosine-resistant mutants of Escherichia coli K-12 with growth-medium-dependent lysl-tRNA synthetase activity. I. Isolation and physiological characterization. Biochim Biophys Acta. 1972 Feb 15;259(3):330–343. [PubMed] [Google Scholar]
- Ito K. Regulatory mechanism of the tryptophan operon in Escherichia coli: possible interaction between trpR and trpS gene products. Mol Gen Genet. 1972;115(4):349–363. doi: 10.1007/BF00333173. [DOI] [PubMed] [Google Scholar]
- Klofat W., Picciolo G., Chappelle E. W., Freese E. Production of adenosine triphosphate in normal cells and sporulation mutants of Bacillus subtilis. J Biol Chem. 1969 Jun 25;244(12):3270–3276. [PubMed] [Google Scholar]
- LOWRY O. H., ROSEBROUGH N. J., FARR A. L., RANDALL R. J. Protein measurement with the Folin phenol reagent. J Biol Chem. 1951 Nov;193(1):265–275. [PubMed] [Google Scholar]
- Leighton T. J., Doi R. H. The stability of messenger ribonucleic acid during sporulation in Bacillus subtilis. J Biol Chem. 1971 May 25;246(10):3189–3195. [PubMed] [Google Scholar]
- Littauer U. Z., Inouye H. Regulation of tRNA. Annu Rev Biochem. 1973;42:439–470. doi: 10.1146/annurev.bi.42.070173.002255. [DOI] [PubMed] [Google Scholar]
- Malik V. S. Chloramphenicol. Adv Appl Microbiol. 1972;15:297–336. doi: 10.1016/s0065-2164(08)70095-9. [DOI] [PubMed] [Google Scholar]
- McGinnis E., Williams L. S. Regulation of histidyl-transfer ribonucleic acid synthetase formation in a histidyl-transfer ribonucleic acid synthetase mutant of Salmonella typhimurium. J Bacteriol. 1972 Sep;111(3):739–744. doi: 10.1128/jb.111.3.739-744.1972. [DOI] [PMC free article] [PubMed] [Google Scholar]
- Milhaud P., Balassa G. Biochemical genetics of bacterial sporulation. IV. Sequential development of resistances to chemical and physical agents during sporulation of Bacillus subtilis. Mol Gen Genet. 1973 Sep 12;125(3):241–250. doi: 10.1007/BF00270746. [DOI] [PubMed] [Google Scholar]
- Miller L. L., Ordal Z. J. Thermal injury and recovery of Bacillus subtilis. Appl Microbiol. 1972 Dec;24(6):878–884. doi: 10.1128/am.24.6.878-884.1972. [DOI] [PMC free article] [PubMed] [Google Scholar]
- Parker J., Flashner M., Mckeever W. G., Neidhardt F. C. Metabolic regulation of the arginyl and valyl transfer ribonucleic acid synthetases in bacteria. J Biol Chem. 1974 Feb 25;249(4):1044–1053. [PubMed] [Google Scholar]
- Printz D. B., Gross S. R. An apparent relationship between mistranslation and an altered leucyl-tRNA synthetase in a conditional lethal mutant of Neurospora crassa. Genetics. 1967 Mar;55(3):451–467. doi: 10.1093/genetics/55.3.451. [DOI] [PMC free article] [PubMed] [Google Scholar]
- Racine F. M., Steinberg W. Defects of two temperature-sensitive lysyl-transfer ribonucleic acid synthetase mutants of Bacillus subtilis. J Bacteriol. 1974 Oct;120(1):372–383. doi: 10.1128/jb.120.1.372-383.1974. [DOI] [PMC free article] [PubMed] [Google Scholar]
- Racine F. M., Steinberg W. Genetic location of two mutations affecting the lysyl-transfer ribonucleic acid synthetase of Bacillus subtilis. J Bacteriol. 1974 Oct;120(1):384–389. doi: 10.1128/jb.120.1.384-389.1974. [DOI] [PMC free article] [PubMed] [Google Scholar]
- Russell R. R. Temperature-sensitive osmotic remedial mutants of Escherichia coli. J Bacteriol. 1972 Nov;112(2):661–665. doi: 10.1128/jb.112.2.661-665.1972. [DOI] [PMC free article] [PubMed] [Google Scholar]
- Spizizen J. TRANSFORMATION OF BIOCHEMICALLY DEFICIENT STRAINS OF BACILLUS SUBTILIS BY DEOXYRIBONUCLEATE. Proc Natl Acad Sci U S A. 1958 Oct 15;44(10):1072–1078. doi: 10.1073/pnas.44.10.1072. [DOI] [PMC free article] [PubMed] [Google Scholar]
- Steinberg W., Anagnostopoulos C. Biochemical and genetic characterization of a temperature-sensitive, tryptophanyl-transfer ribonucleic acid synthetase mutant of Bacillus subtilis. J Bacteriol. 1971 Jan;105(1):6–19. doi: 10.1128/jb.105.1.6-19.1971. [DOI] [PMC free article] [PubMed] [Google Scholar]
- Steinberg W. Properties and developmental roles of the lysyl- and tryptophanyl-transfer ribonucleic acid synthetases of Bacillus subtilis: common genetic origin of the corresponding spore and vegetative enzymes. J Bacteriol. 1974 Apr;118(1):70–82. doi: 10.1128/jb.118.1.70-82.1974. [DOI] [PMC free article] [PubMed] [Google Scholar]
- Steinberg W. Temperature-induced derepression of tryptophan biosynthesis in a tryptophanyl-transfer ribonucleic acid synthetase mutant of Bacillus subtilis. J Bacteriol. 1974 Mar;117(3):1023–1034. doi: 10.1128/jb.117.3.1023-1034.1974. [DOI] [PMC free article] [PubMed] [Google Scholar]
- Sterlini J. M., Mandelstam J. Commitment to sporulation in Bacillus subtilis and its relationship to development of actinomycin resistance. Biochem J. 1969 Jun;113(1):29–37. doi: 10.1042/bj1130029. [DOI] [PMC free article] [PubMed] [Google Scholar]
- Stone A. B. Regulation of cell division in a temperature-sensitive division mutant of Escherichia coli. J Bacteriol. 1973 Nov;116(2):741–750. doi: 10.1128/jb.116.2.741-750.1973. [DOI] [PMC free article] [PubMed] [Google Scholar]
- Straus D. S., Ames B. N. Histidyl-transfer ribonucleic acid synthetase mutants requiring a high internal pool of histidine for growth. J Bacteriol. 1973 Jul;115(1):188–197. doi: 10.1128/jb.115.1.188-197.1973. [DOI] [PMC free article] [PubMed] [Google Scholar]
- Tempest D. W., Meers J. L., Brown C. M. Influence of environment on the content and composition of microbial free amino acid pools. J Gen Microbiol. 1970 Dec;64(2):171–185. doi: 10.1099/00221287-64-2-171. [DOI] [PubMed] [Google Scholar]
- Vold B. S. Variations in activity of aminoacyl-tRNA synthetases as a function of development in Bacillus subtilis. Arch Biochem Biophys. 1973 Feb;154(2):691–695. doi: 10.1016/0003-9861(73)90024-6. [DOI] [PubMed] [Google Scholar]
- Williams L. S., Neidhardt F. C. Synthesis and inactivation of aminoacyl-transfer RNA synthetases during growth of Escherichia coli. J Mol Biol. 1969 Aug 14;43(3):529–550. doi: 10.1016/0022-2836(69)90357-x. [DOI] [PubMed] [Google Scholar]
- Yaniv M., Gros F. Studies on valyl-tRNA synthetase and tRNA from Escherichia coli. 3. Valyl-tRNA synthetases from thermosensitive mutants of Escherichia coli. J Mol Biol. 1969 Aug 28;44(1):31–45. doi: 10.1016/0022-2836(69)90403-3. [DOI] [PubMed] [Google Scholar]
- Yarus M., Mertes M. The variety of intraspecific misacylations carried out by isoleucyl transfer ribonucleic acid synthetase of Escherichia coli. J Biol Chem. 1973 Oct 10;248(19):6744–6749. [PubMed] [Google Scholar]