Abstract
The two species of 6-phosphogluconate dehydrogenase (EC 1.1.1.43) from Pseudomonas multivorans were resolved from extracts of gluconate-grown bacteria and purified to homogeneity. Each enzyme comprised between 0.1 and 0.2% of the total cellular protein. Separation of the two enzymes, one which is specific for nicotinamide adenine dinucleotide phosphate and the other which is active with nicotinamide adenine dinucleotide or nicotinamide adenine dinucleotide phosphate was facilitated by the marked difference in their respective isoelectric points, which were at pH 5.0 and 6.9. Comparison of the subunit compositions of the two enzymes indicated that they do not share common peptide chains. The enzyme active with nicotinamide adenine dinucleotide was composed of two subunits of about 40,000 molecular weight, and the nicotinamide adenine dinucleotide phosphate-specific enzyme was composed of two subunits of about 60,000 molecular weight. Immunological studies indicated that the two enzymes do not share common antigenic determinants. Reduced nicotinamide adenine dinucleotide phosphate strongly inhibited the 6-phosphogluconate dehydrogenase active with nicotinamide adenine dinucleotide by decreasing its affinity for 6-phosphogluconate. Guanosine-5'-triphosphate had a similar influence on the nicotinamide adenine dinucleotide phosphate-specific 6-phosphogluconate dehydrogenase. These results in conjunction with other data indicating that reduced nicotinamide adenine dinucleotide phosphate stimulates the conversion of 6-phosphogluconate to pyruvate by crude bacterial extracts suggest that in P. multivorans, the relative distribution of 6-phosphogluconate into the pentose phosphate and Entner-Doudoroff pathways might be determined by the intracellular concentrations of reduced nicotinamide adenine dinucleotide phosphate and purine nucleotides.
Full text
PDF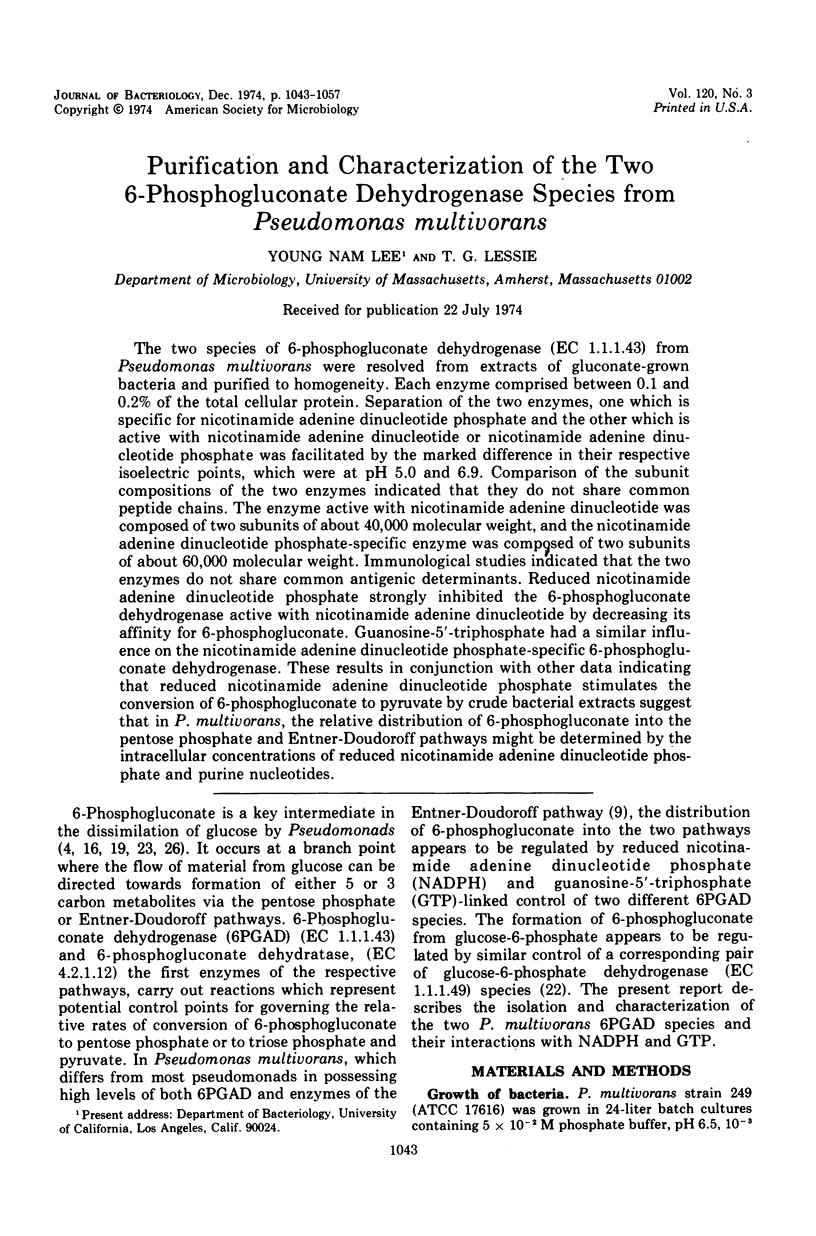
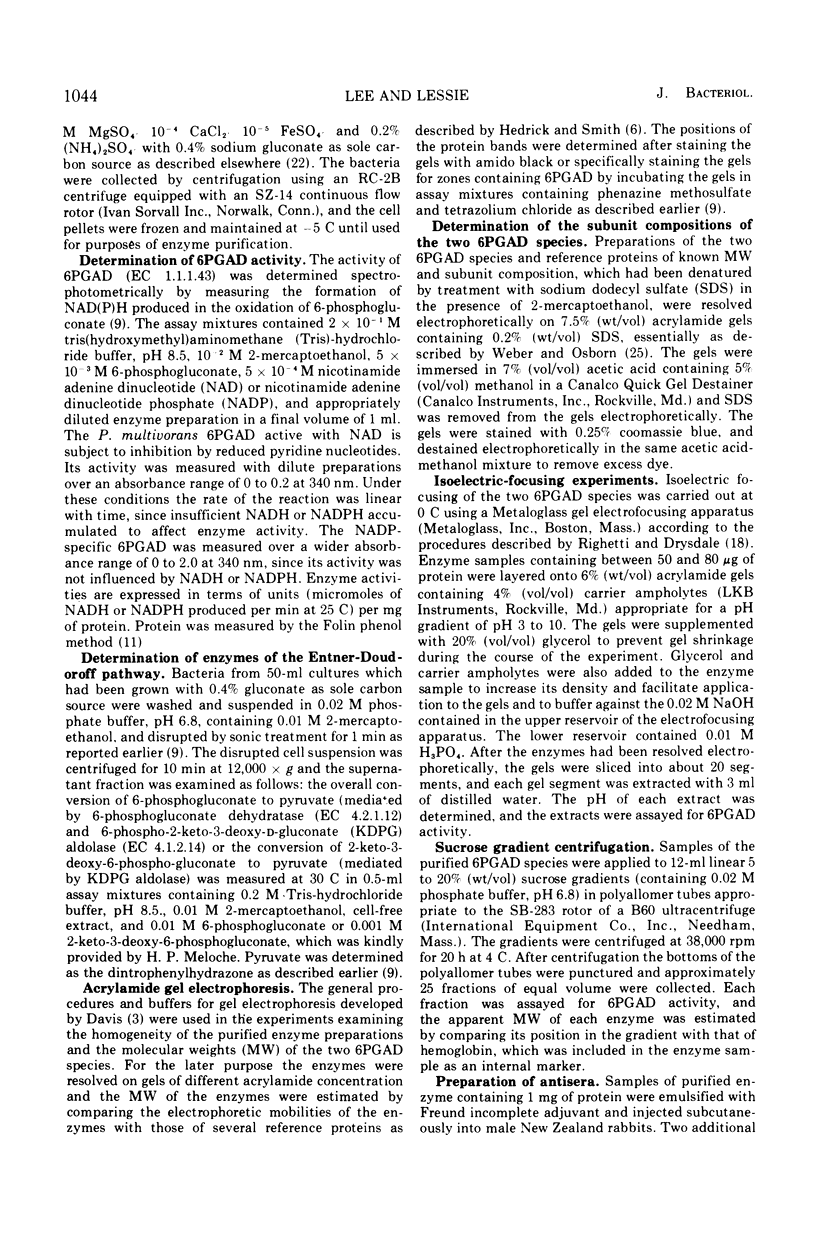
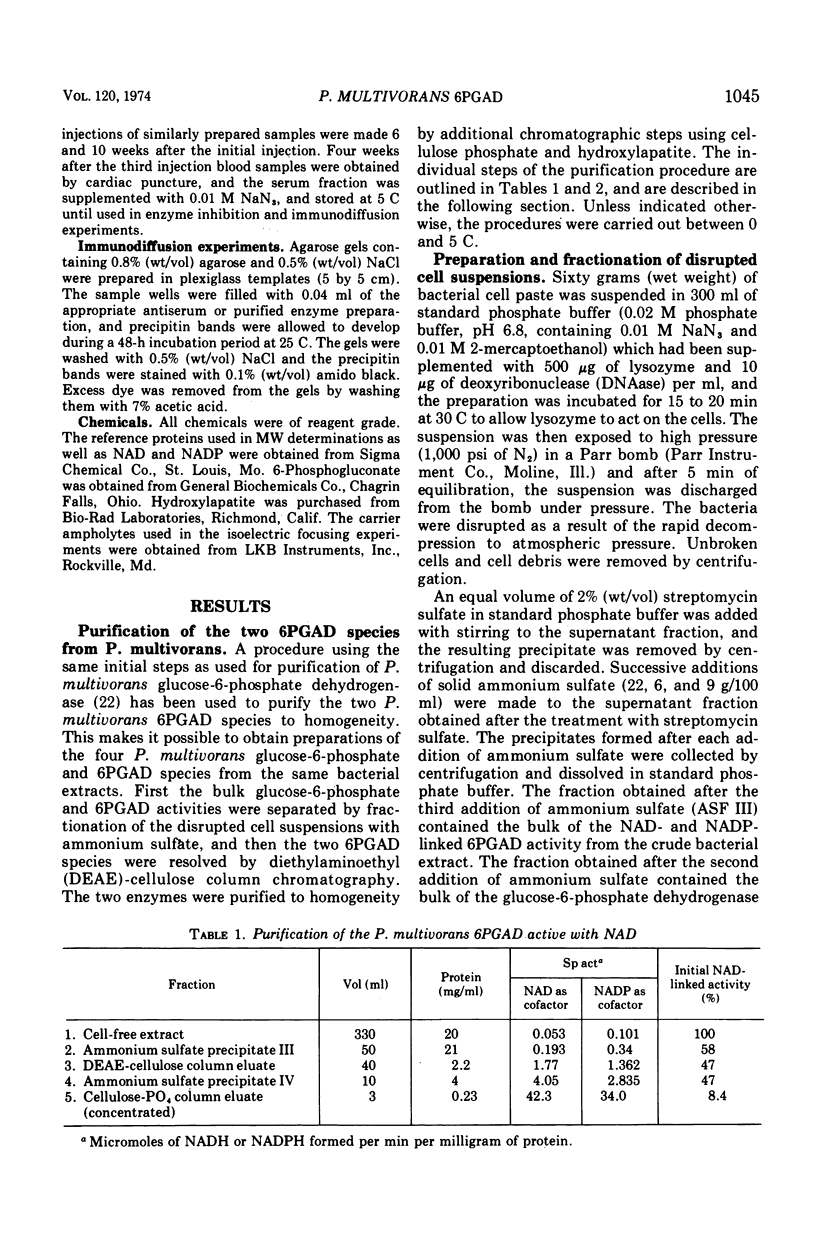
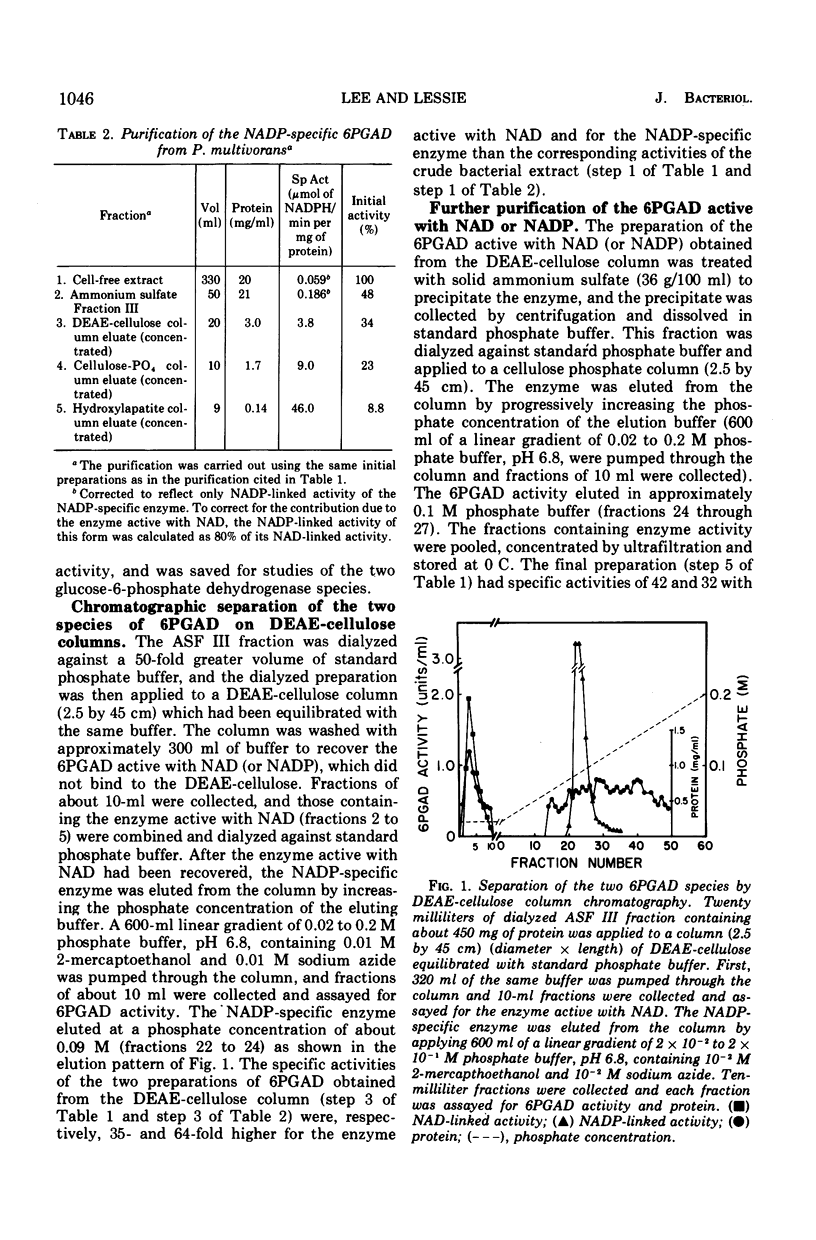
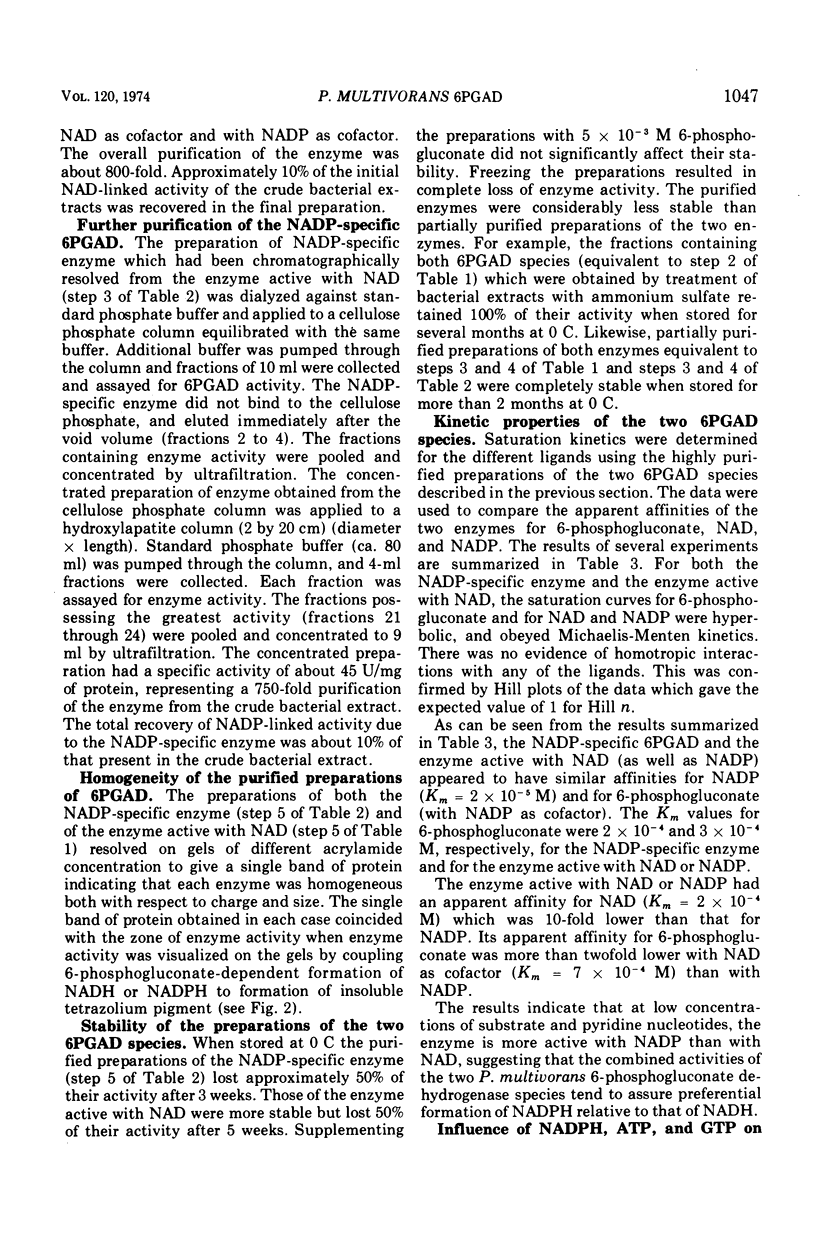
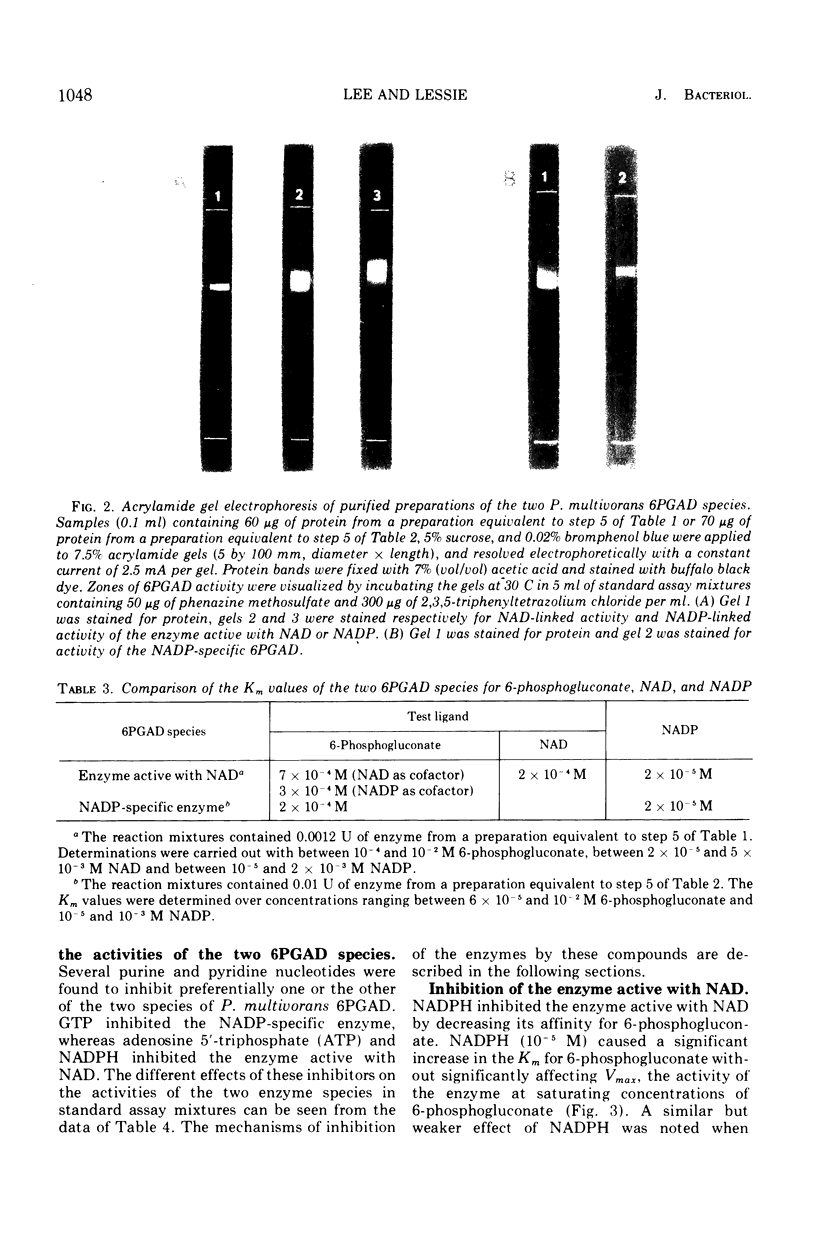
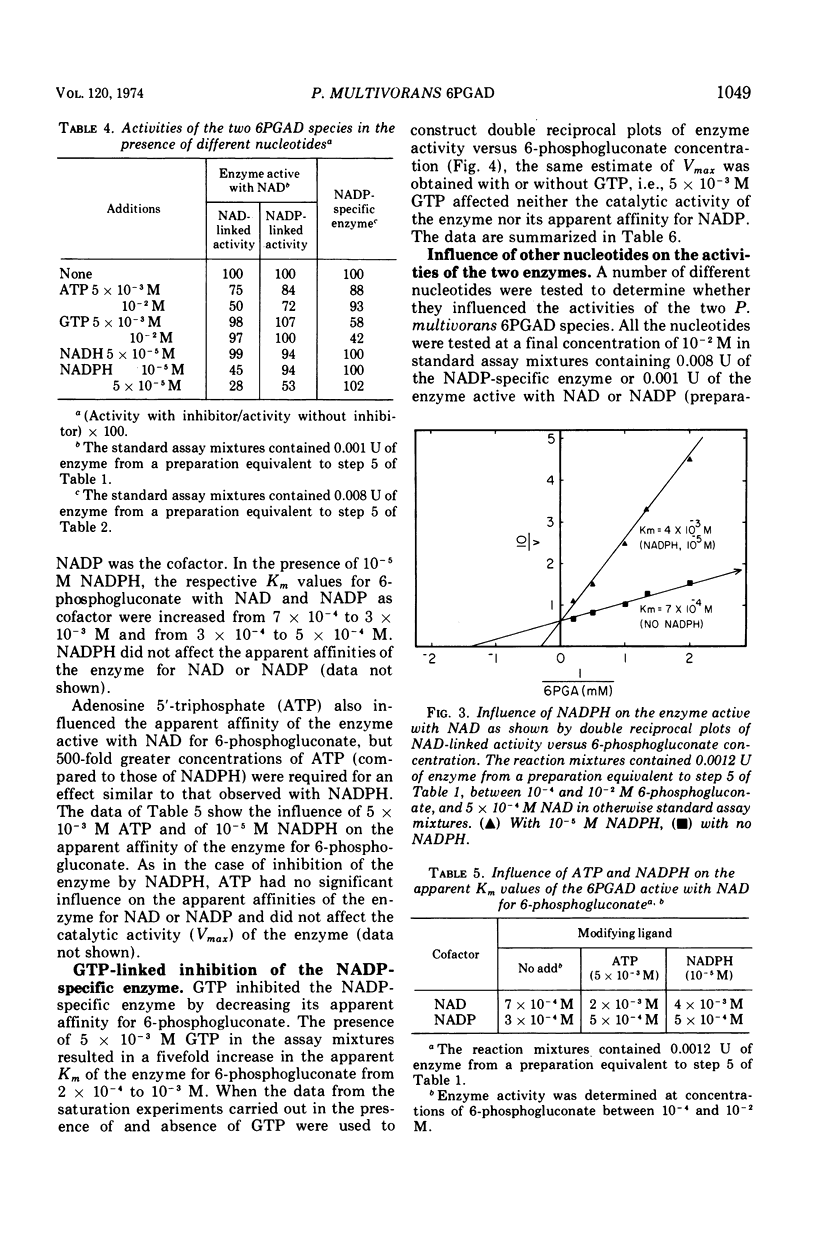
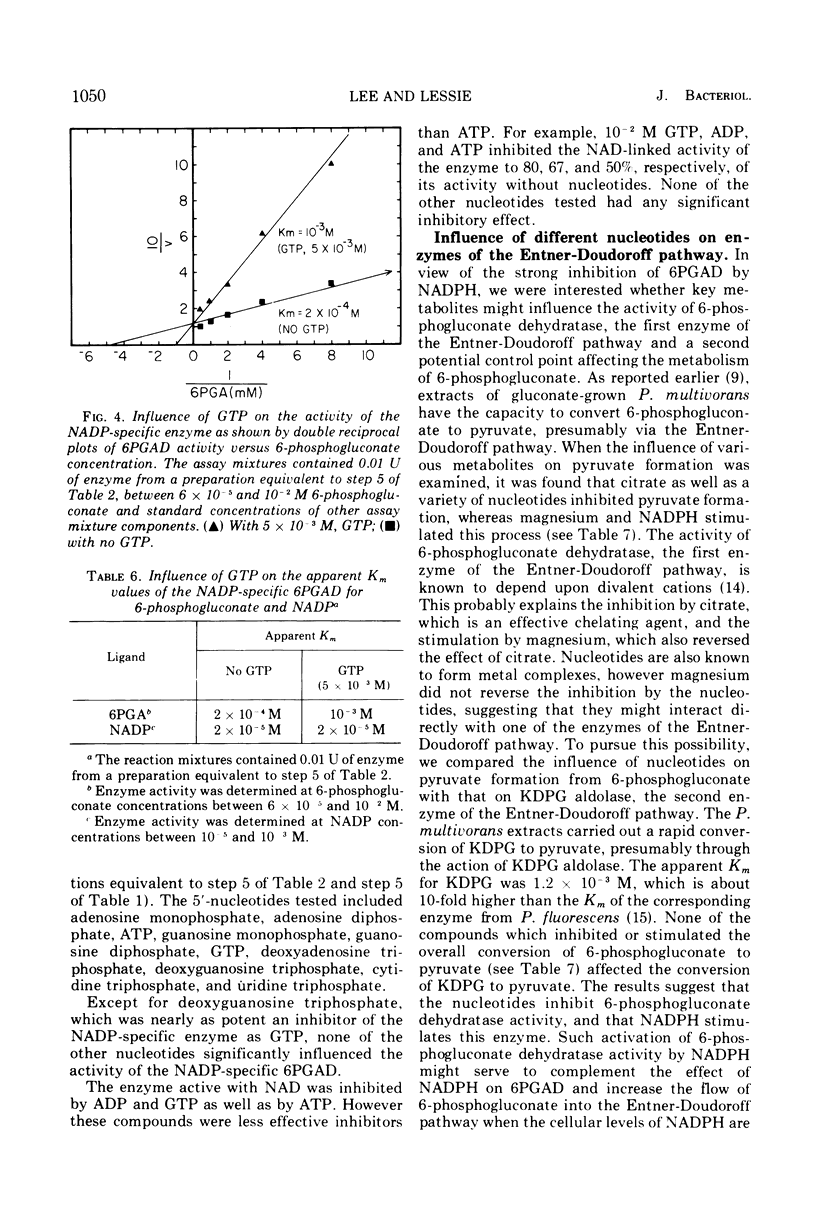
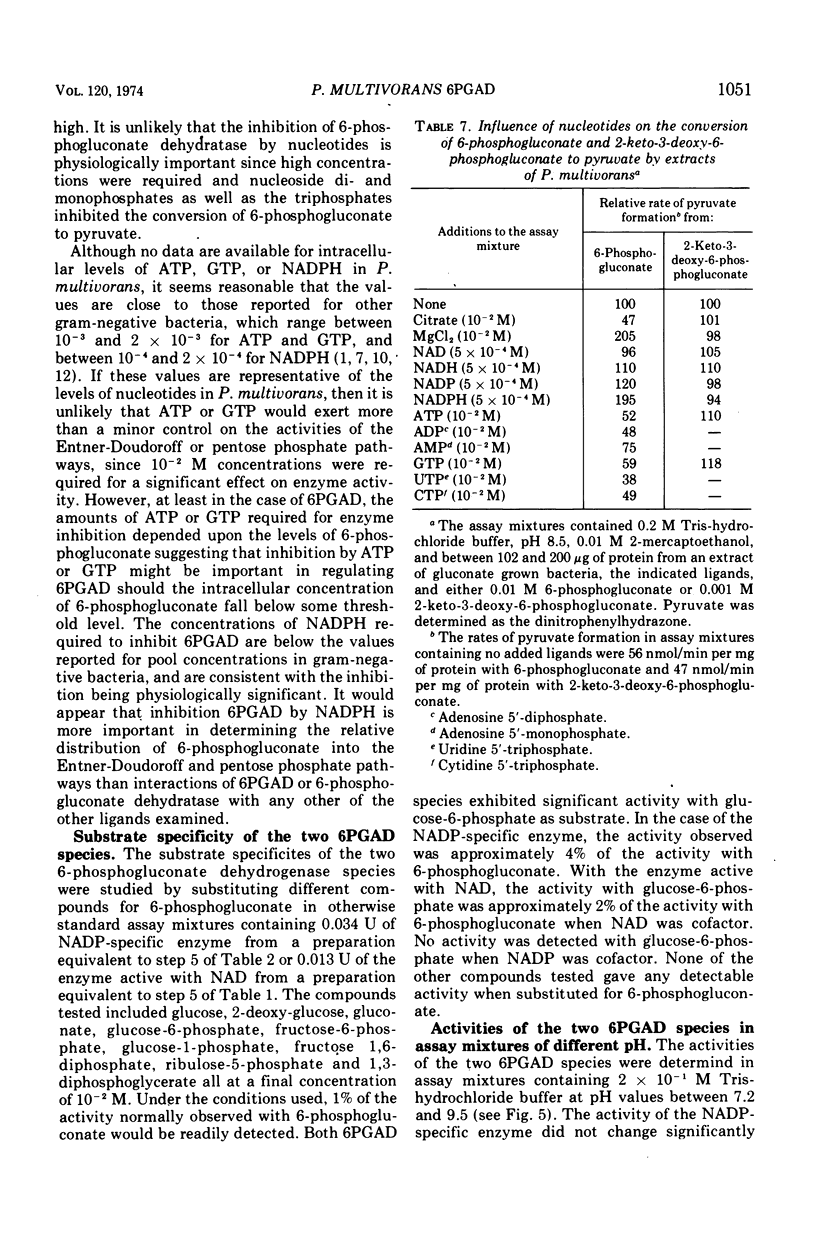
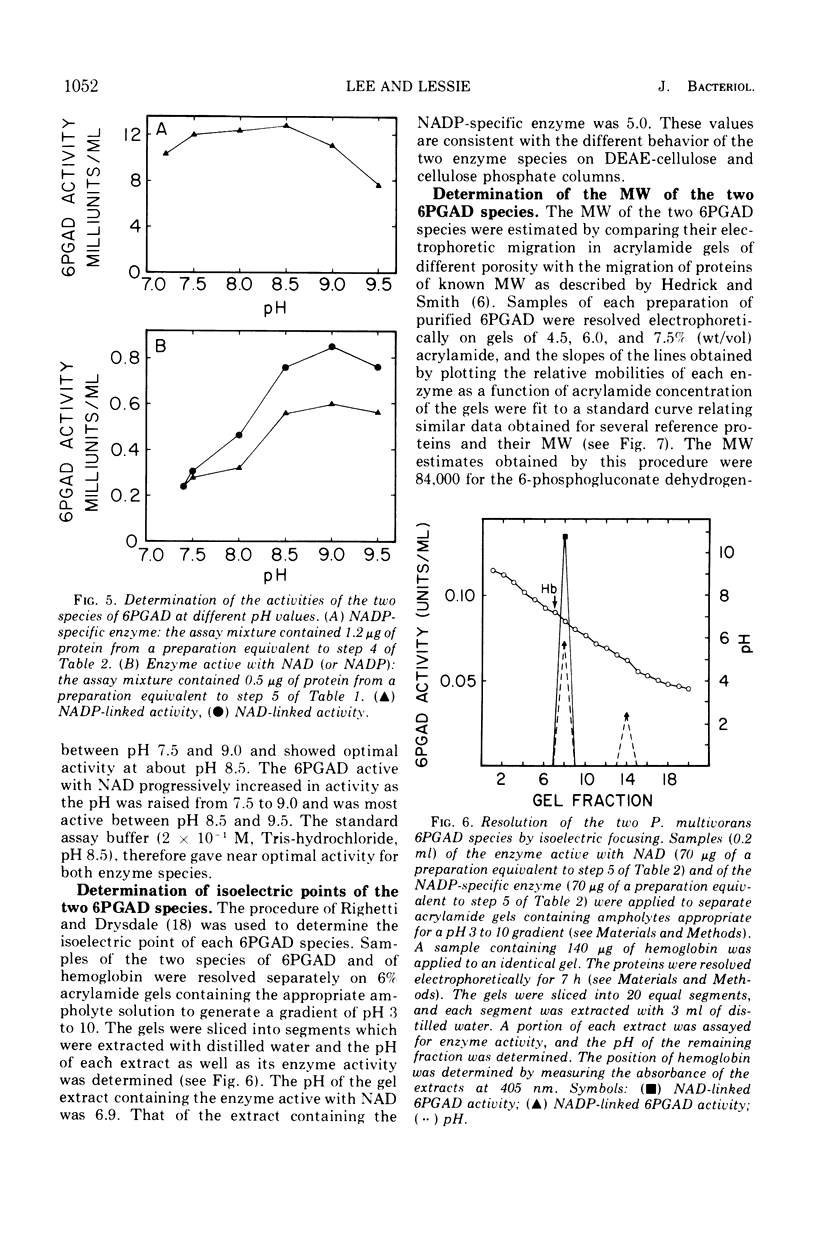
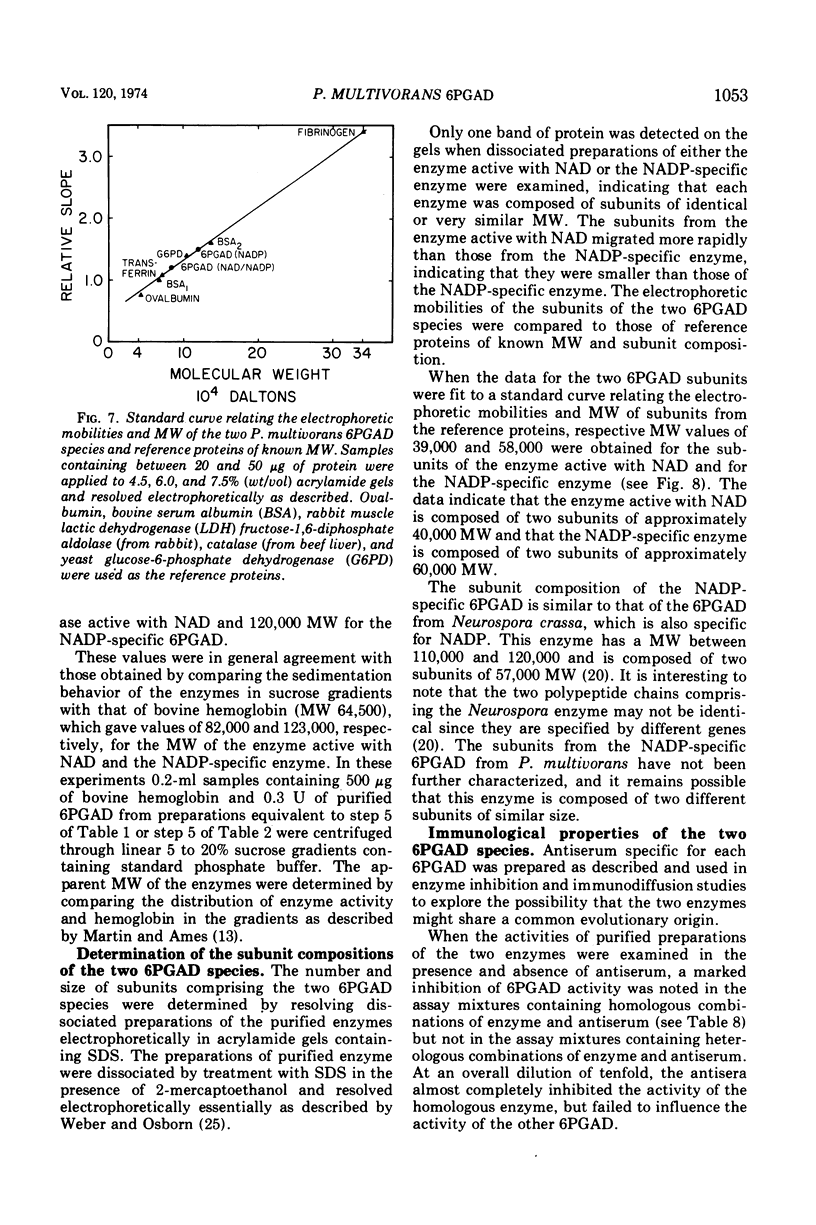
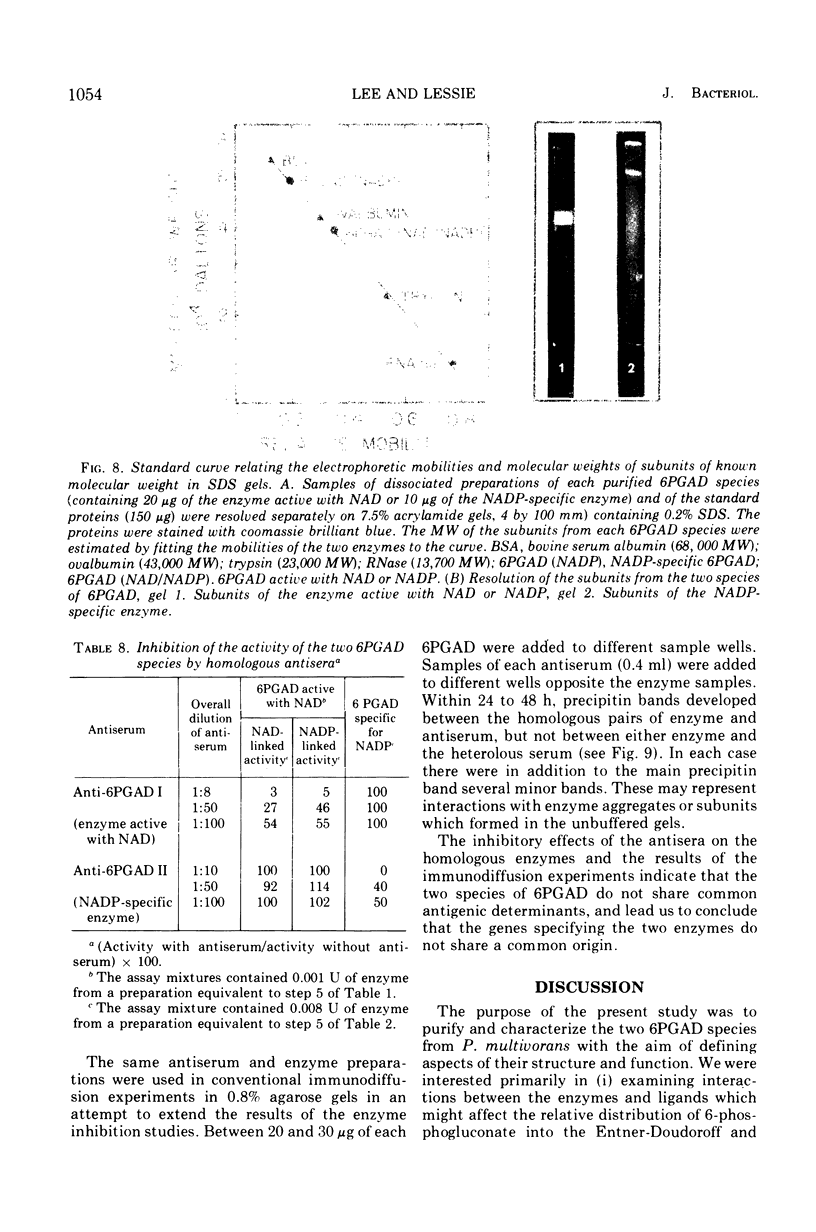
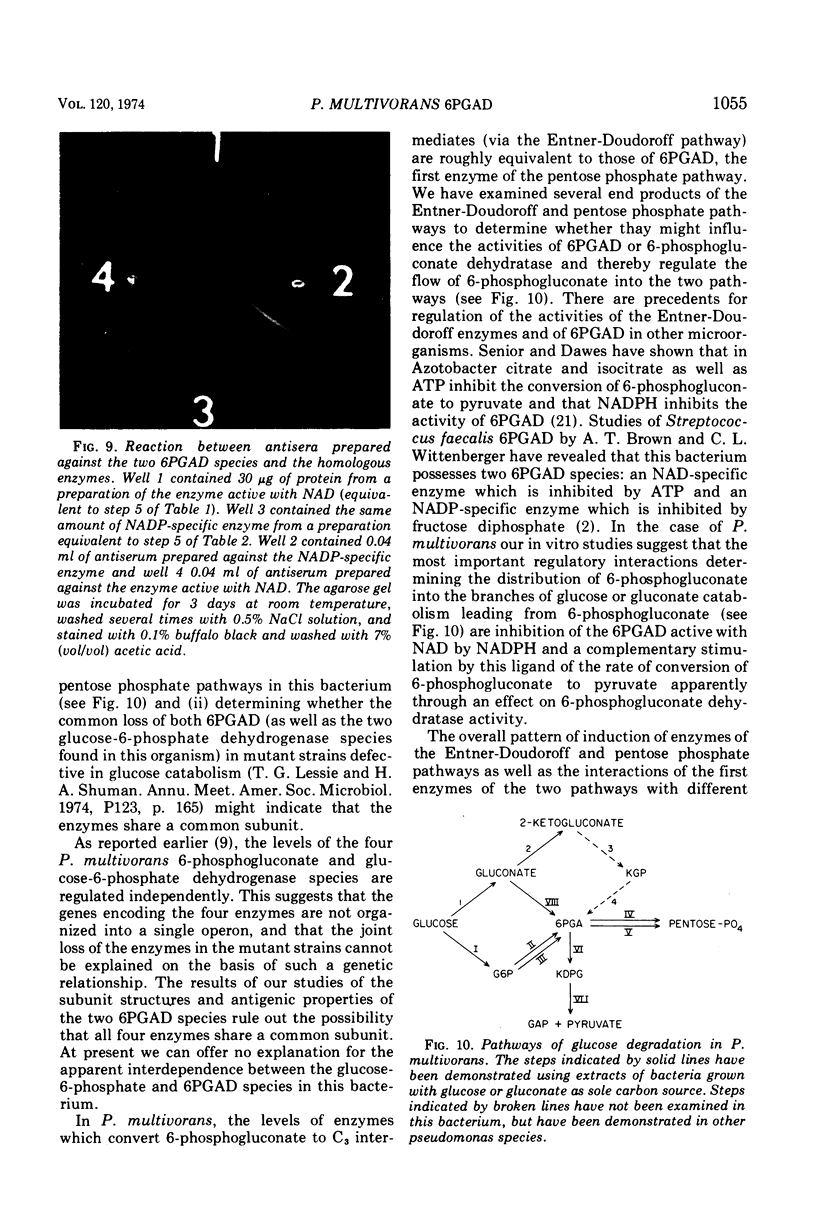
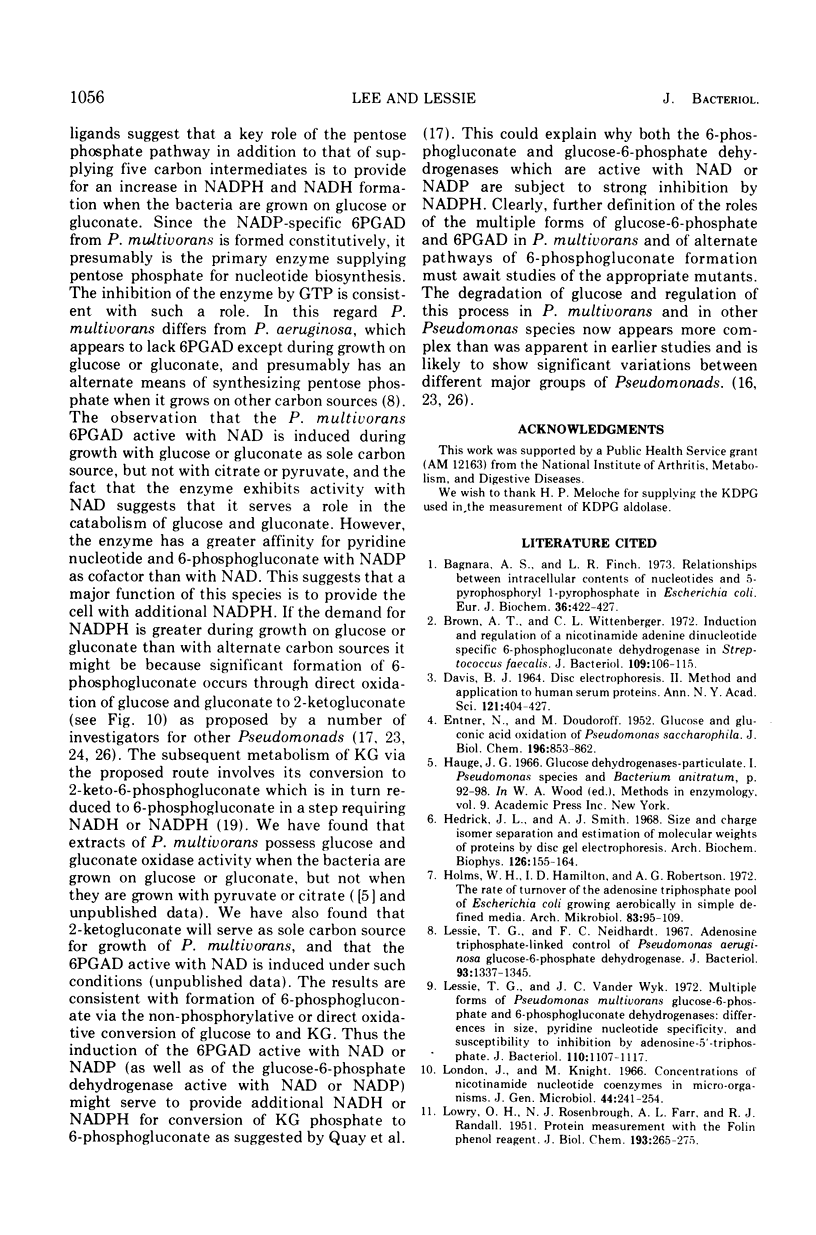
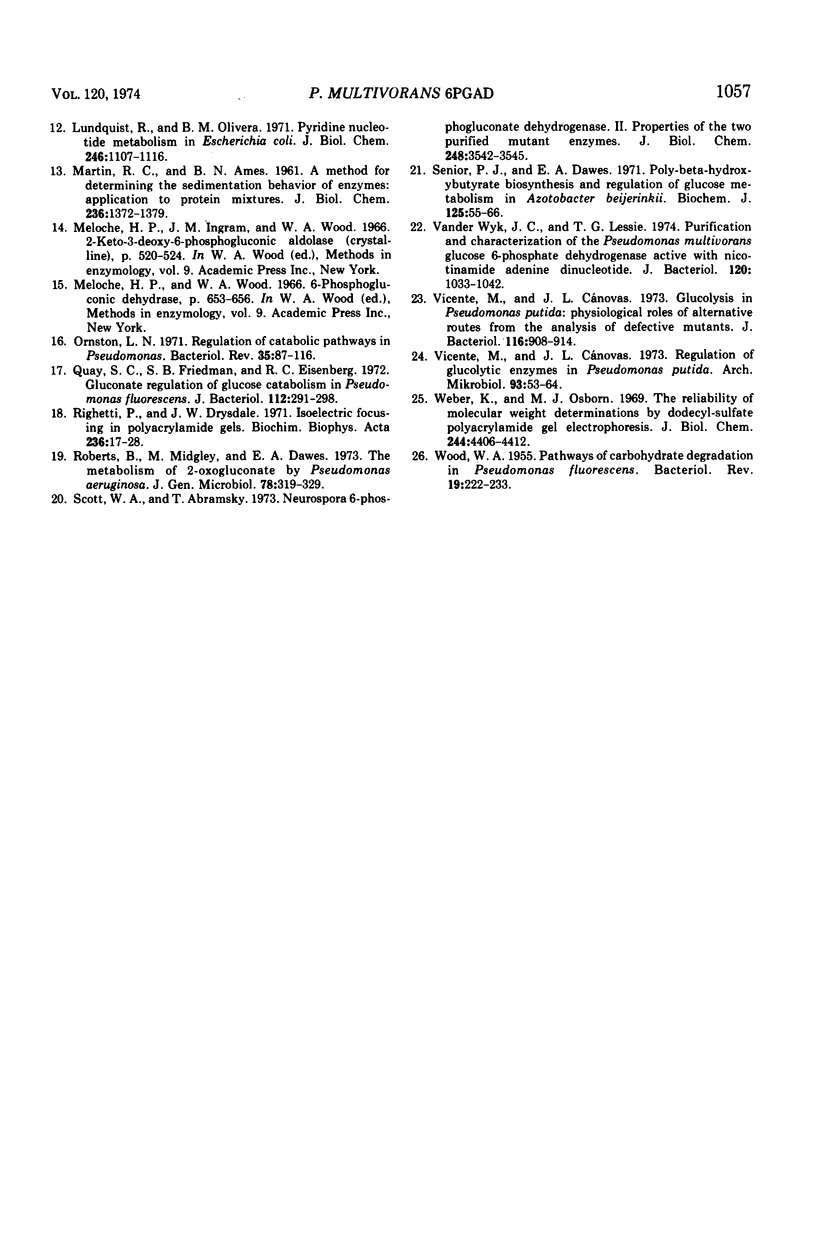
Images in this article
Selected References
These references are in PubMed. This may not be the complete list of references from this article.
- Bagnara A. S., Finch L. R. Relationships between intracellular contents of nucleotides and 5-phosphoribosyl 1-pyrophosphate in Escherichia coli. Eur J Biochem. 1973 Jul 16;36(2):422–427. doi: 10.1111/j.1432-1033.1973.tb02927.x. [DOI] [PubMed] [Google Scholar]
- Brown A. T., Wittenberger C. L. Induction and regulation of a nicotinamide adenine dinucleotide-specific 6-phosphogluconate dehydrogenase in Streptococcus faecalis. J Bacteriol. 1972 Jan;109(1):106–115. doi: 10.1128/jb.109.1.106-115.1972. [DOI] [PMC free article] [PubMed] [Google Scholar]
- DAVIS B. J. DISC ELECTROPHORESIS. II. METHOD AND APPLICATION TO HUMAN SERUM PROTEINS. Ann N Y Acad Sci. 1964 Dec 28;121:404–427. doi: 10.1111/j.1749-6632.1964.tb14213.x. [DOI] [PubMed] [Google Scholar]
- ENTNER N., DOUDOROFF M. Glucose and gluconic acid oxidation of Pseudomonas saccharophila. J Biol Chem. 1952 May;196(2):853–862. [PubMed] [Google Scholar]
- Hedrick J. L., Smith A. J. Size and charge isomer separation and estimation of molecular weights of proteins by disc gel electrophoresis. Arch Biochem Biophys. 1968 Jul;126(1):155–164. doi: 10.1016/0003-9861(68)90569-9. [DOI] [PubMed] [Google Scholar]
- Holms W. H., Hamilton I. D., Robertson A. G. The rate of turnover of the adenosine triphosphate pool of Escherichia coli growing aerobically in simple defined media. Arch Mikrobiol. 1972;83(2):95–109. doi: 10.1007/BF00425016. [DOI] [PubMed] [Google Scholar]
- LOWRY O. H., ROSEBROUGH N. J., FARR A. L., RANDALL R. J. Protein measurement with the Folin phenol reagent. J Biol Chem. 1951 Nov;193(1):265–275. [PubMed] [Google Scholar]
- Lessie T. G., Wyk J. C. Multiple forms of Pseudomonas multivorans glucose-6-phosphate and 6-phosphogluconate dehydrogenases: differences in size, pyridine nucleotide specificity, and susceptibility to inhibition by adenosine 5'-triphosphate. J Bacteriol. 1972 Jun;110(3):1107–1117. doi: 10.1128/jb.110.3.1107-1117.1972. [DOI] [PMC free article] [PubMed] [Google Scholar]
- Lessie T., Neidhardt F. C. Adenosine triphosphate-linked control of Pseudomonas aeruginosa glucose-6-phosphate dehydrogenase. J Bacteriol. 1967 Apr;93(4):1337–1345. doi: 10.1128/jb.93.4.1337-1345.1967. [DOI] [PMC free article] [PubMed] [Google Scholar]
- London J., Knight M. Concentrations of nicotinamide nucleotide coenzymes in micro-organisms. J Gen Microbiol. 1966 Aug;44(2):241–254. doi: 10.1099/00221287-44-2-241. [DOI] [PubMed] [Google Scholar]
- Lundquist R., Olivera B. M. Pyridine nucleotide metabolism in Escherichia coli. I. Exponential growth. J Biol Chem. 1971 Feb 25;246(4):1107–1116. [PubMed] [Google Scholar]
- MARTIN R. G., AMES B. N. A method for determining the sedimentation behavior of enzymes: application to protein mixtures. J Biol Chem. 1961 May;236:1372–1379. [PubMed] [Google Scholar]
- Ornston L. N. Regulation of catabolic pathways in Pseudomonas. Bacteriol Rev. 1971 Jun;35(2):87–116. doi: 10.1128/br.35.2.87-116.1971. [DOI] [PMC free article] [PubMed] [Google Scholar]
- Quay S. C., Friedman S. B., Eisenberg R. C. Gluconate regulation of glucose catabolism in Pseudomonas fluorescens. J Bacteriol. 1972 Oct;112(1):291–298. doi: 10.1128/jb.112.1.291-298.1972. [DOI] [PMC free article] [PubMed] [Google Scholar]
- Righetti P., Drysdale J. W. Isoelectric focusing in polyacrylamide gels. Biochim Biophys Acta. 1971 Apr 27;236(1):17–28. doi: 10.1016/0005-2795(71)90144-9. [DOI] [PubMed] [Google Scholar]
- Roberts B. K., Midgley M., Dawes E. A. The metabolism of 2-oxogluconate by Pseudomonas aeruginosa. J Gen Microbiol. 1973 Oct;78(2):319–329. doi: 10.1099/00221287-78-2-319. [DOI] [PubMed] [Google Scholar]
- Scott W. A., Abramsky T. Neurospora 6-phosphogluconate dehydrogenase. II. Properties of two purified mutant enzymes. J Biol Chem. 1973 May 25;248(10):3542–3545. [PubMed] [Google Scholar]
- Senior P. J., Dawes E. A. Poly- -hydroxybutyrate biosynthesis and the regulation of glucose metabolism in Azotobacter beijerinckii. Biochem J. 1971 Nov;125(1):55–66. doi: 10.1042/bj1250055. [DOI] [PMC free article] [PubMed] [Google Scholar]
- Vander Wyk J. C., Lessie T. G. Purification and characterization of the Pseudomonas multivorans glucose-6-phosphate dehydrogenase active with nicotinamide adenine dinucleotide. J Bacteriol. 1974 Dec;120(3):1033–1042. doi: 10.1128/jb.120.3.1033-1042.1974. [DOI] [PMC free article] [PubMed] [Google Scholar]
- Vicente M., Cánovas J. L. Glucolysis in Pseudomonas putida: physiological role of alternative routes from the analysis of defective mutants. J Bacteriol. 1973 Nov;116(2):908–914. doi: 10.1128/jb.116.2.908-914.1973. [DOI] [PMC free article] [PubMed] [Google Scholar]
- Vicente M., Cánovas J. L. Regulation of the glucolytic enzymes in Pseudomonas putida. Arch Mikrobiol. 1973 Oct 4;93(1):53–64. doi: 10.1007/BF00666080. [DOI] [PubMed] [Google Scholar]
- WOOD W. A. Pathways of carbohydrate degradation in Pseudomonas fluorescens. Bacteriol Rev. 1955 Dec;19(4):222–233. doi: 10.1128/br.19.4.222-233.1955. [DOI] [PMC free article] [PubMed] [Google Scholar]
- Weber K., Osborn M. The reliability of molecular weight determinations by dodecyl sulfate-polyacrylamide gel electrophoresis. J Biol Chem. 1969 Aug 25;244(16):4406–4412. [PubMed] [Google Scholar]