Abstract
Streptococcus faecalis obtains metabolic energy chiefly from the conversion of glucose to lactic acid; the present experiments deal with the mechanism of lactic acid translocation across the cytoplasmic membrane. Efflux of [14C]lactate from preloaded cells was accelerated by raising the external pH, and also by the ionophores nigericin and valinomycin. These results suggest that lactate leaves the cell by an electroneutral process, presumably as lactic acid. Further evidence was obtained by studying the entry of [14C]lactate into nonmetabolizing cells. It appears that the membrane is essentially impermeable to the lactate anion, but allows passage of lactic acid. The most persuasive evidence is that, upon establishment of a pH gradient such that the cytoplasm was alkaline, l-[14C]lactate accumulated in the cells against the concentration gradient. Accumulation was transient, and dissipated in parallel with the collapse of the pH gradient. The concentration gradient attained at the peak was a function of the pH difference. Ionophores which are known to collapse a pH gradient, such as nigericin and valinomycin, abolished accumulation of l-lactate. We infer that lactic acid translocation, whether into the cells or outward, is an electroneutral process and for that reason the distribution of lactic acid across the membrane is a function of the pH of cytoplasm and medium. The specificity of translocation and its kinetic parameters suggest that it is mediated by a carrier of low specificity.
Full text
PDF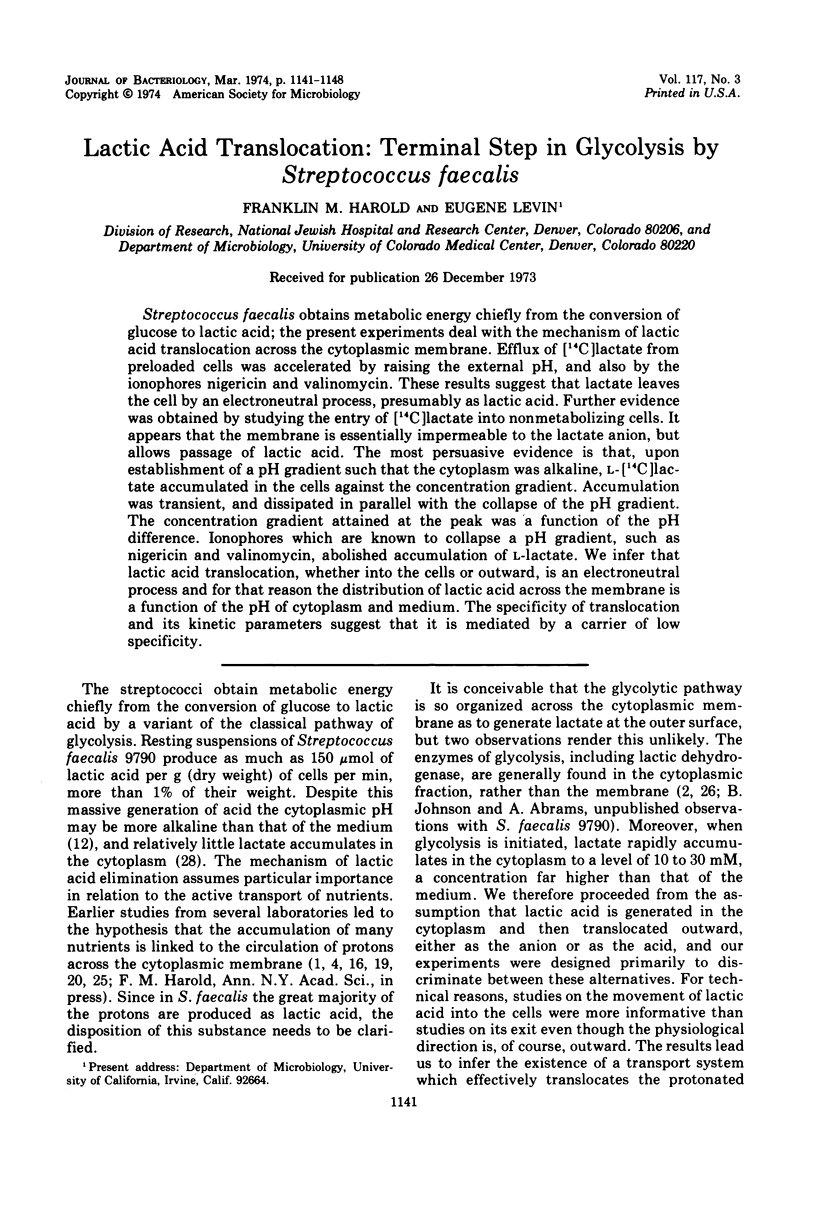
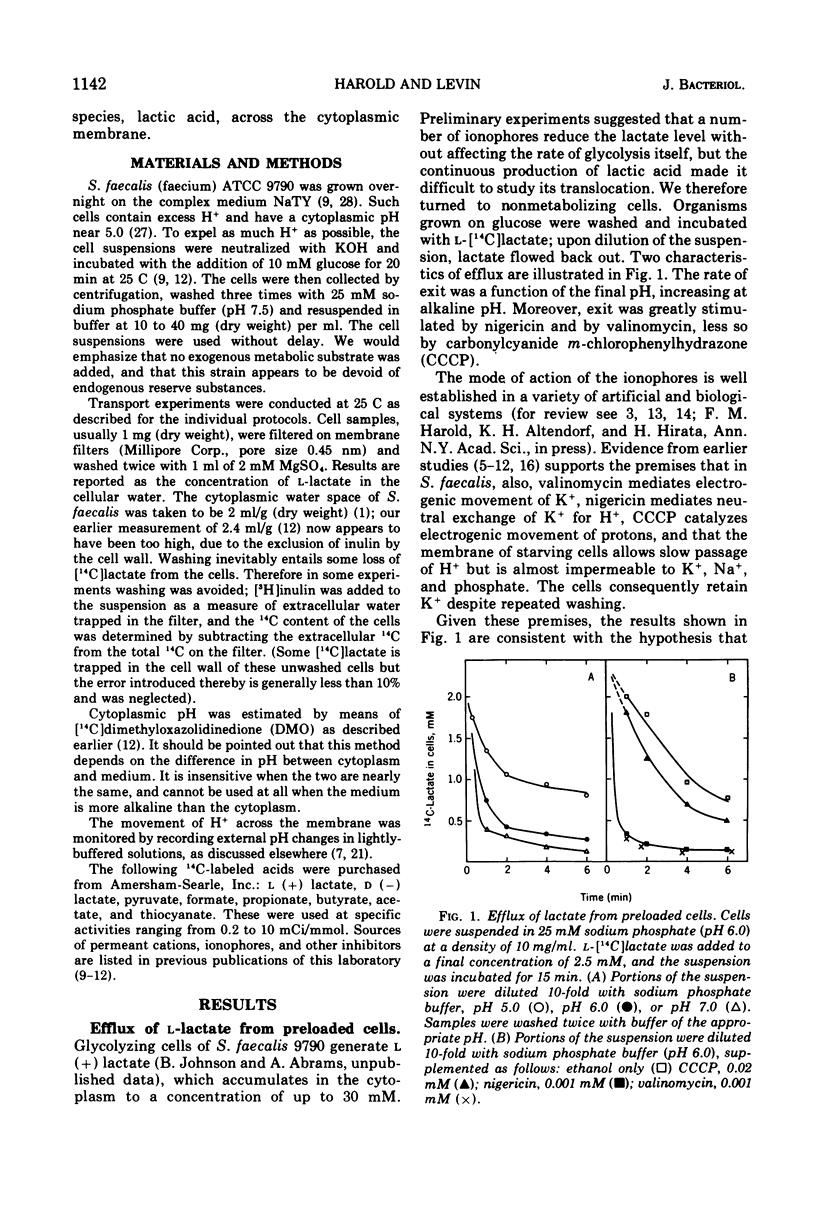
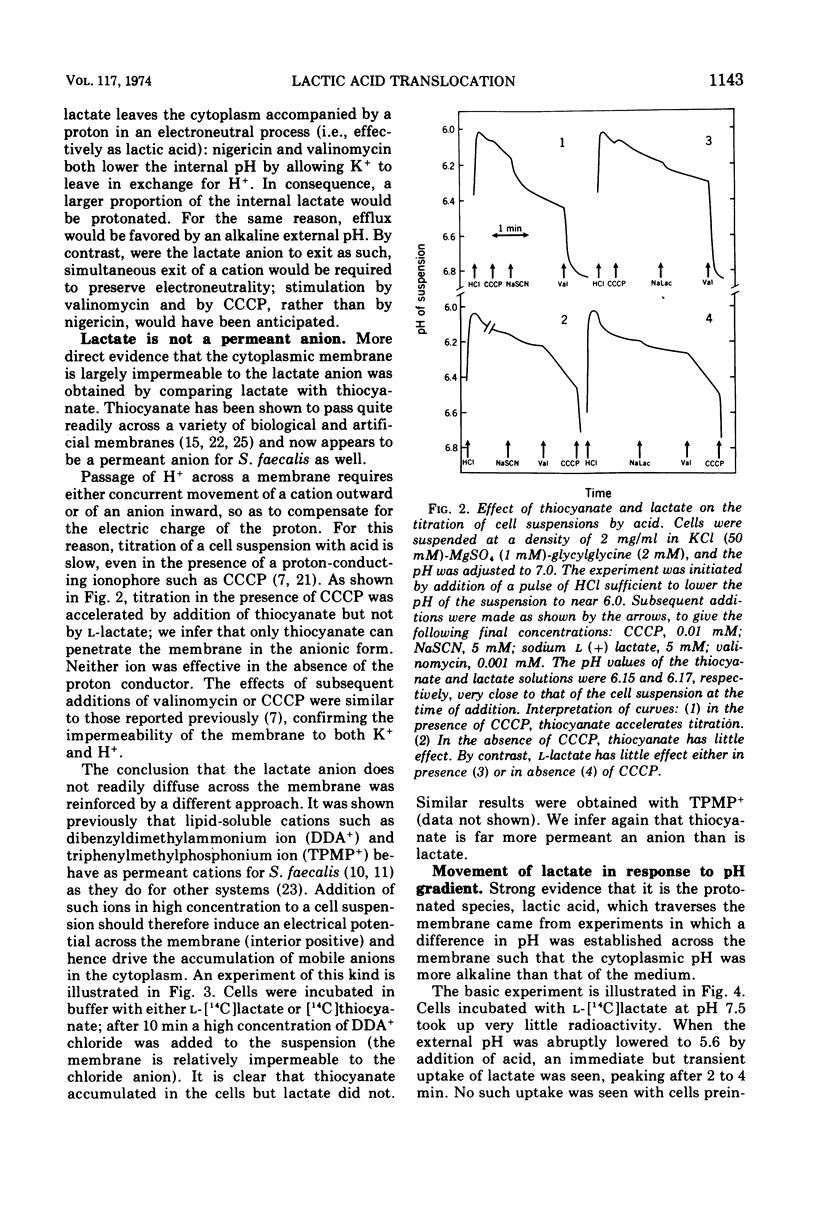
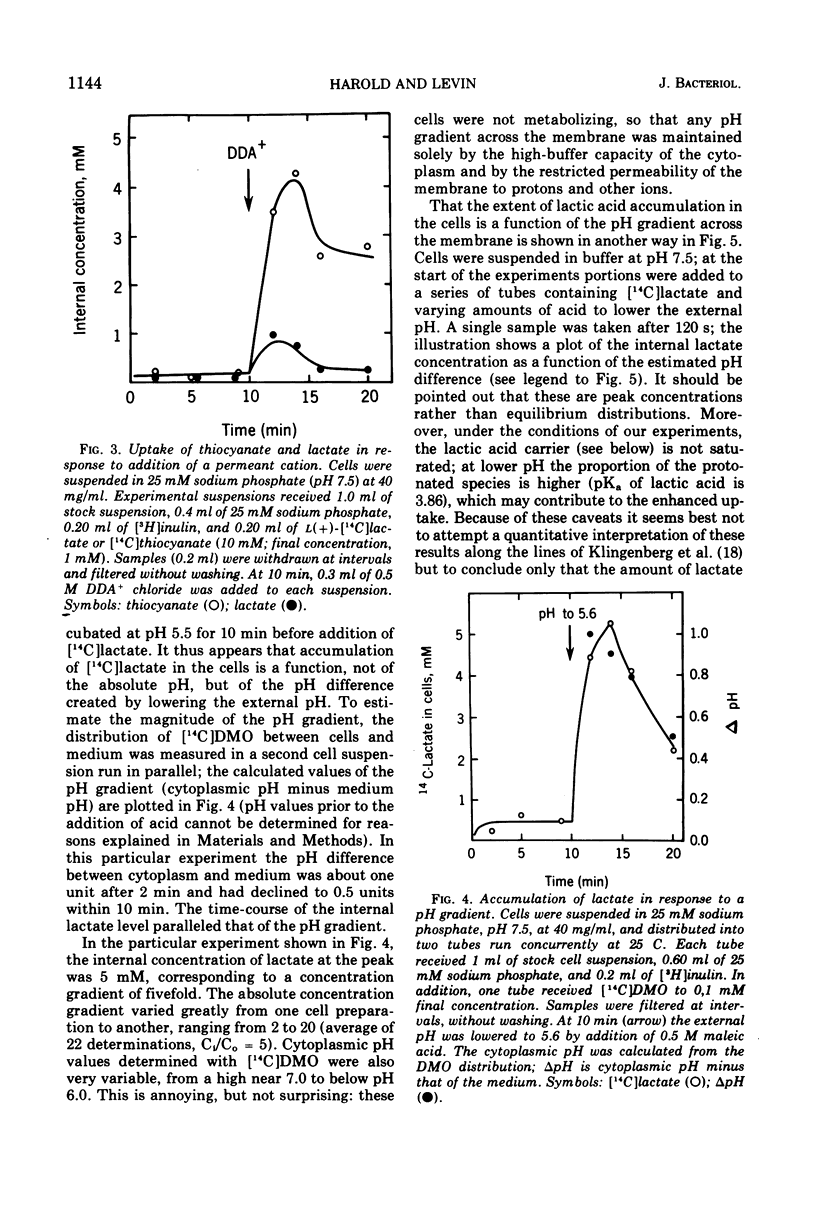
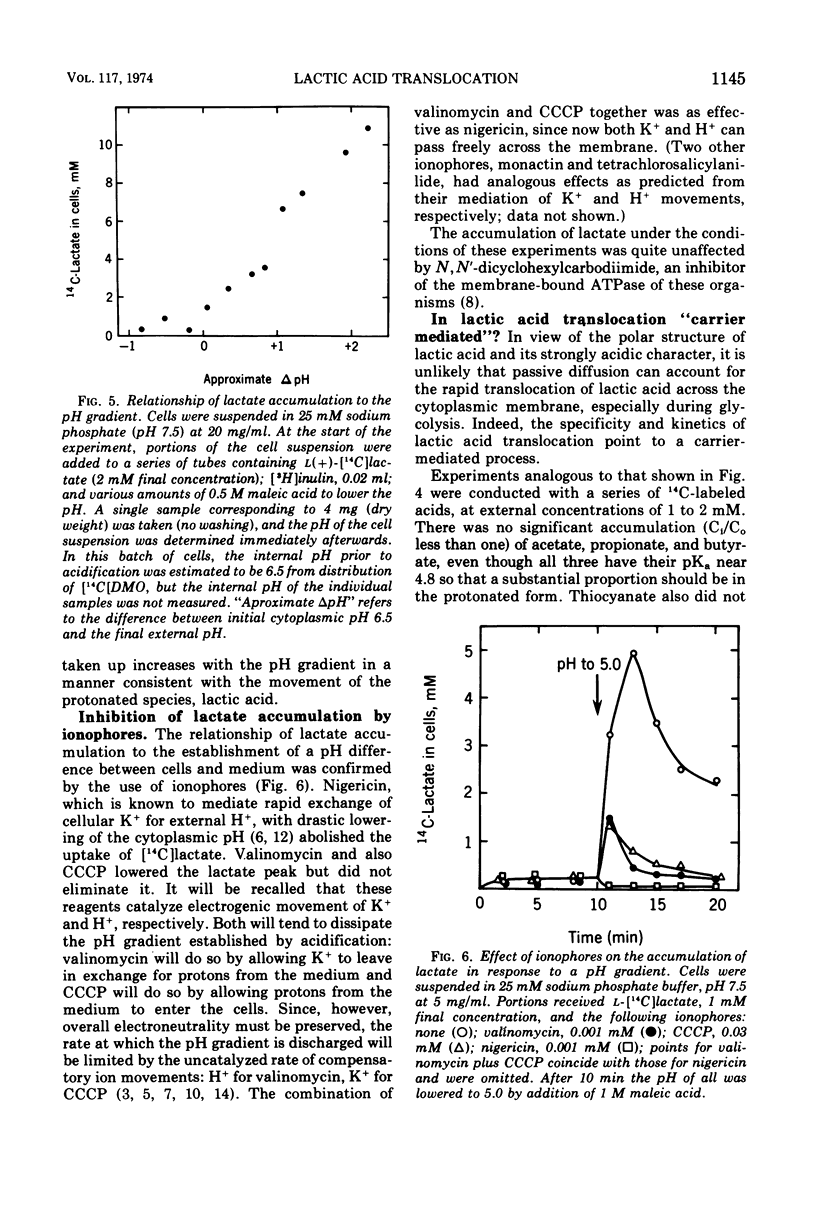
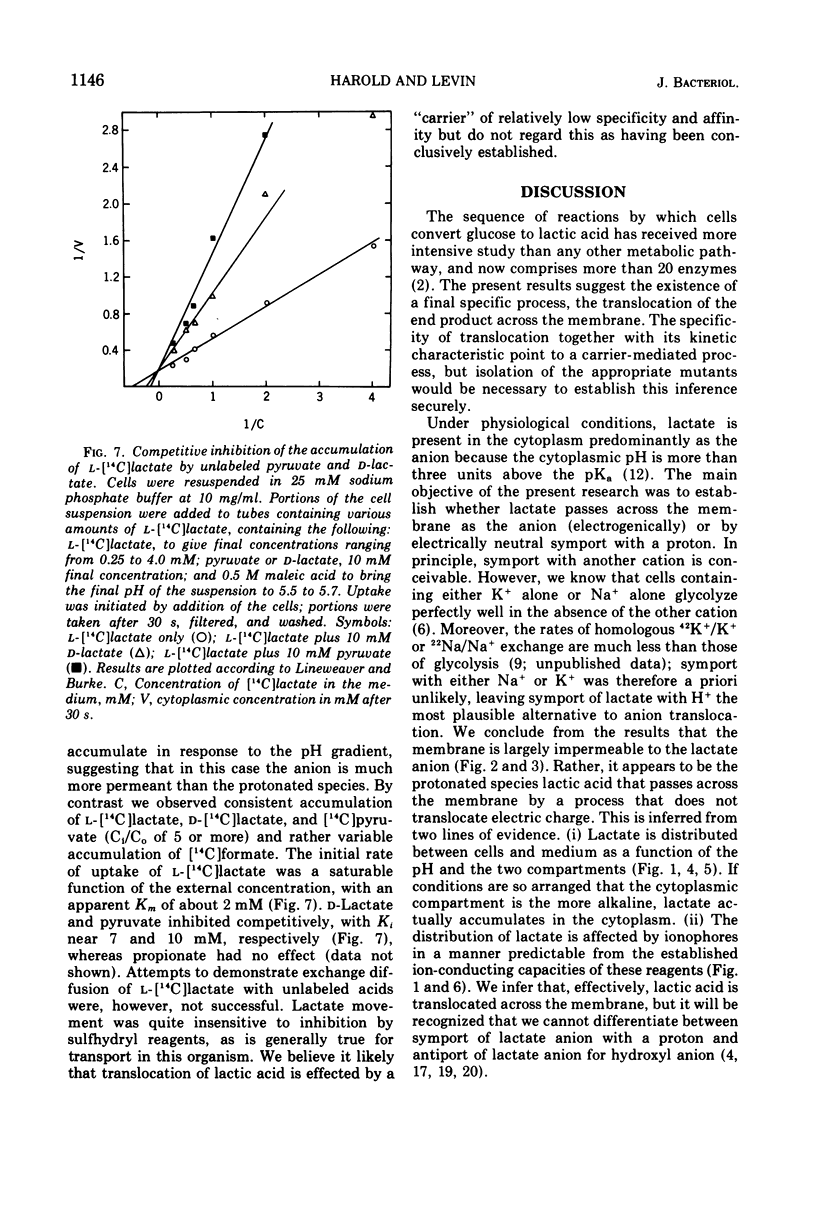
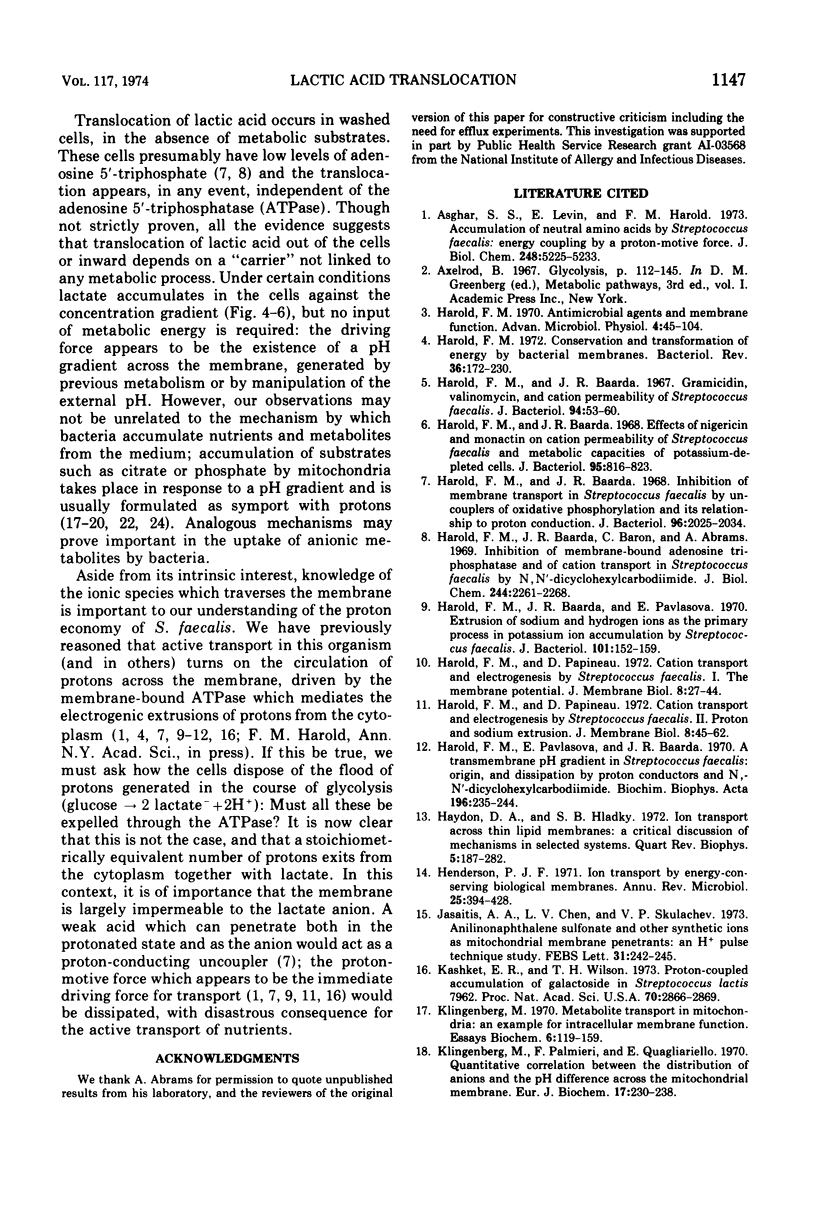
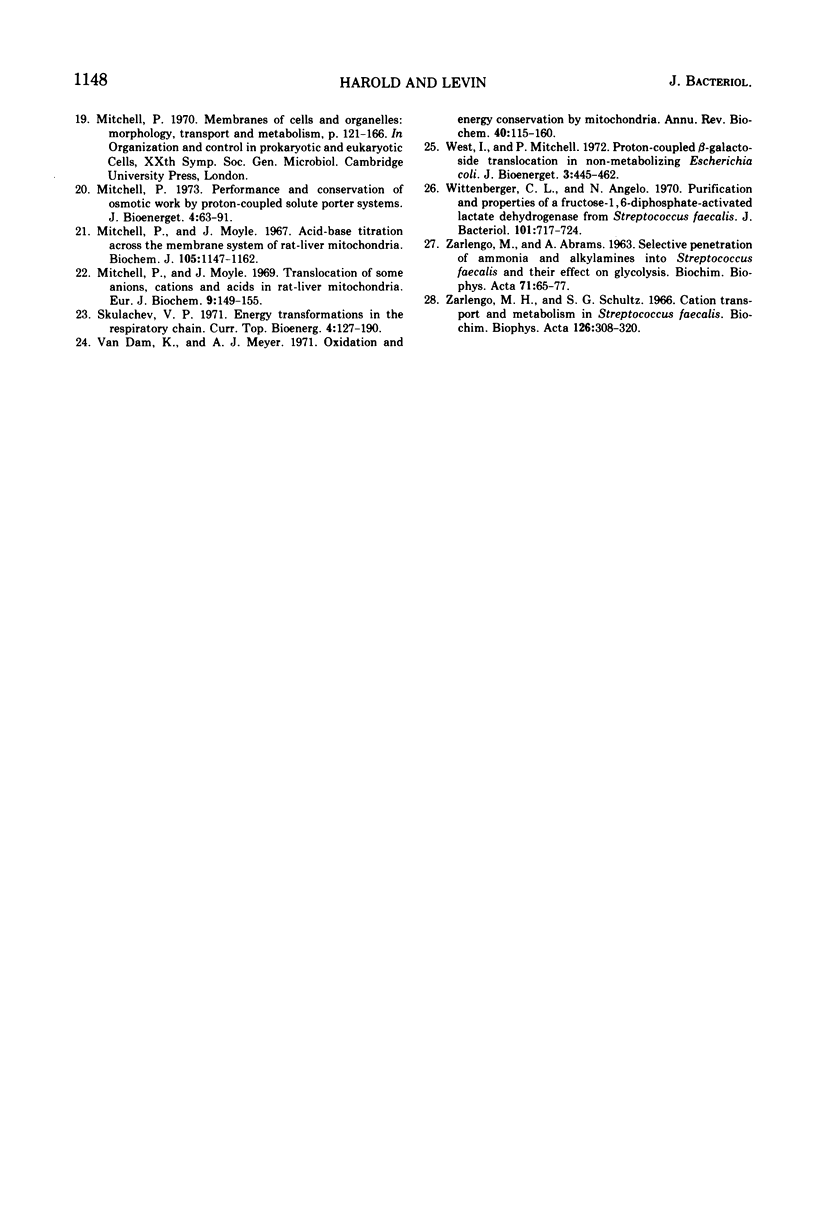
Selected References
These references are in PubMed. This may not be the complete list of references from this article.
- Asghar S. S., Levin E., Harold F. M. Accumulation of neutral amino acids by Streptococcus faecalis. Energy coupling by a proton-motive force. J Biol Chem. 1973 Aug 10;248(15):5225–5233. [PubMed] [Google Scholar]
- Harold F. M., Baarda J. R., Baron C., Abrams A. Inhibition of membrane-bound adenosine triphosphatase and of cation transport in Streptococcus faecalis by N,N'-dicyclohexylcarbodiimide. J Biol Chem. 1969 May 10;244(9):2261–2268. [PubMed] [Google Scholar]
- Harold F. M., Baarda J. R. Effects of nigericin and monactin on cation permeability of Streptococcus faecalis and metabolic capacities of potassium-depleted cells. J Bacteriol. 1968 Mar;95(3):816–823. doi: 10.1128/jb.95.3.816-823.1968. [DOI] [PMC free article] [PubMed] [Google Scholar]
- Harold F. M., Baarda J. R. Gramicidin, valinomycin, and cation permeability of Streptococcus faecalis. J Bacteriol. 1967 Jul;94(1):53–60. doi: 10.1128/jb.94.1.53-60.1967. [DOI] [PMC free article] [PubMed] [Google Scholar]
- Harold F. M., Baarda J. R. Inhibition of membrane transport in Streptococcus faecalis by uncouplers of oxidative phosphorylation and its relationship to proton conduction. J Bacteriol. 1968 Dec;96(6):2025–2034. doi: 10.1128/jb.96.6.2025-2034.1968. [DOI] [PMC free article] [PubMed] [Google Scholar]
- Harold F. M., Baarda J. R., Pavlasova E. Extrusion of sodium and hydrogen ions as the primary process in potassium ion accumulation by Streptococcus faecalis. J Bacteriol. 1970 Jan;101(1):152–159. doi: 10.1128/jb.101.1.152-159.1970. [DOI] [PMC free article] [PubMed] [Google Scholar]
- Harold F. M. Conservation and transformation of energy by bacterial membranes. Bacteriol Rev. 1972 Jun;36(2):172–230. doi: 10.1128/br.36.2.172-230.1972. [DOI] [PMC free article] [PubMed] [Google Scholar]
- Harold F. M., Papineau D. Cation transport and electrogenesis by Streptococcus faecalis. I. The membrane potential. J Membr Biol. 1972;8(1):27–44. doi: 10.1007/BF01868093. [DOI] [PubMed] [Google Scholar]
- Harold F. M., Papineau D. Cation transport and electrogenesis by Streptococcus faecalis. II. Proton and sodium extrusion. J Membr Biol. 1972;8(1):45–62. doi: 10.1007/BF01868094. [DOI] [PubMed] [Google Scholar]
- Harold F. M., Pavlasová E., Baarda J. R. A transmembrane pH gradient in Streptococcus faecalis: origin, and dissipation by proton conductors and N,N'-dicyclohexylcarbodimide. Biochim Biophys Acta. 1970;196(2):235–244. doi: 10.1016/0005-2736(70)90011-8. [DOI] [PubMed] [Google Scholar]
- Haydon D. A., Hladky S. B. Ion transport across thin lipid membranes: a critical discussion of mechanisms in selected systems. Q Rev Biophys. 1972 May;5(2):187–282. doi: 10.1017/s0033583500000883. [DOI] [PubMed] [Google Scholar]
- Henderson P. J. Ion transport by energy-conserving biological membranes. Annu Rev Microbiol. 1971;25:393–428. doi: 10.1146/annurev.mi.25.100171.002141. [DOI] [PubMed] [Google Scholar]
- Jasaitis A. A., Van Chu L., Skulachev V. P. Anilinonaphthalene sulfonate and other synthetic ions as mitochondrial membrane penetrants: An H(+) pulse technique study. FEBS Lett. 1973 Apr 15;31(2):241–245. doi: 10.1016/0014-5793(73)80113-9. [DOI] [PubMed] [Google Scholar]
- Kashket E. R., Wilson T. H. Proton-coupled accumulation of galactoside in Streptococcus lactis 7962. Proc Natl Acad Sci U S A. 1973 Oct;70(10):2866–2869. doi: 10.1073/pnas.70.10.2866. [DOI] [PMC free article] [PubMed] [Google Scholar]
- Klingenberg M. Metabolite transport in mitochondria: an example for intracellular membrane function. Essays Biochem. 1970;6:119–159. [PubMed] [Google Scholar]
- Klingenberg M., Palmieri F., Quagliariello E. Quantitative correlation between the distribution of anions and the pH difference across the mitochondrial membrane. Eur J Biochem. 1970 Dec;17(2):230–238. doi: 10.1111/j.1432-1033.1970.tb01158.x. [DOI] [PubMed] [Google Scholar]
- Mitchell P., Moyle J. Respiration-driven proton translocation in rat liver mitochondria. Biochem J. 1967 Dec;105(3):1147–1162. doi: 10.1042/bj1051147. [DOI] [PMC free article] [PubMed] [Google Scholar]
- Mitchell P., Moyle J. Translocation of some anions cations and acids in rat liver mitochondria. Eur J Biochem. 1969 Jun;9(2):149–155. doi: 10.1111/j.1432-1033.1969.tb00588.x. [DOI] [PubMed] [Google Scholar]
- Mitchell P. Performance and conservation of osmotic work by proton-coupled solute porter systems. J Bioenerg. 1973 Jan;4(1):63–91. doi: 10.1007/BF01516051. [DOI] [PubMed] [Google Scholar]
- West I., Mitchell P. Proton-coupled beta-galactoside translocation in non-metabolizing Escherichia coli. J Bioenerg. 1972 Aug;3(5):445–462. doi: 10.1007/BF01516082. [DOI] [PubMed] [Google Scholar]
- Wittenberger C. L., Angelo N. Purificationa and properties of a fructose-1,6-diphosphate-activated lactate dehydrogenase from Streptococcus faecalis. J Bacteriol. 1970 Mar;101(3):717–724. doi: 10.1128/jb.101.3.717-724.1970. [DOI] [PMC free article] [PubMed] [Google Scholar]
- ZARLENGO M., ABRAMS A. Selective penetration of ammonia and alkylamines into Streptococcus fecalis and their effect on glycolysis. Biochim Biophys Acta. 1963 Apr 2;71:65–77. doi: 10.1016/0006-3002(63)90986-7. [DOI] [PubMed] [Google Scholar]
- Zarlengo M. H., Schultz S. G. Cation transport and metabolism in Streptococcus fecalis. Biochim Biophys Acta. 1966 Oct 10;126(2):308–320. doi: 10.1016/0926-6585(66)90068-9. [DOI] [PubMed] [Google Scholar]