Abstract
Purine-requiring mutants of Salmonella typhimurium LT2 containing additional mutations in either adenosine deaminase or purine nucleoside phosphorylase have been constructed. From studies of the ability of these mutants to utilize different purine compounds as the sole source of purines, the following conclusions may be drawn. (i) S. typhimurium does not contain physiologically significant amounts of adenine deaminase and adenosine kinase activities. (ii) The presence of inosine and guanosine kinase activities in vivo was established, although the former activity appears to be of minor significance for inosine metabolism. (iii) The utilization of exogenous purine deoxyribonucleosides is entirely dependent on a functional purine nucleoside phosphorylase. (iv) The pathway by which exogenous adenine is converted to guanine nucleotides in the presence of histidine requires a functional purine nucleoside phosphorylase. Evidence is presented that this pathway involves the conversion of adenine to adenosine, followed by deamination to inosine and subsequent phosphorolysis to hypoxanthine. Hypoxanthine is then converted to inosine monophosphate by inosine monophosphate pyrophosphorylase. The rate-limiting step in this pathway is the synthesis of adenosine from adenine due to lack of endogenous ribose-l-phosphate.
Full text
PDF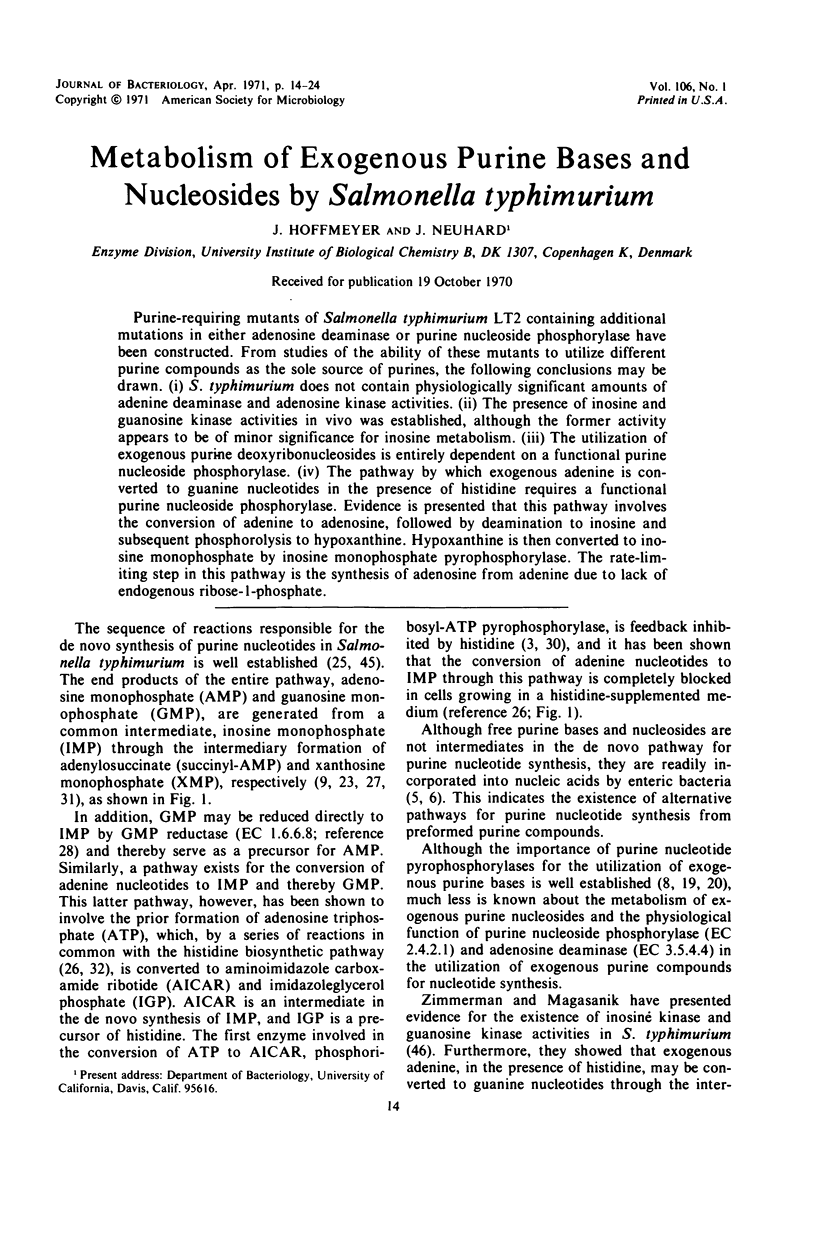
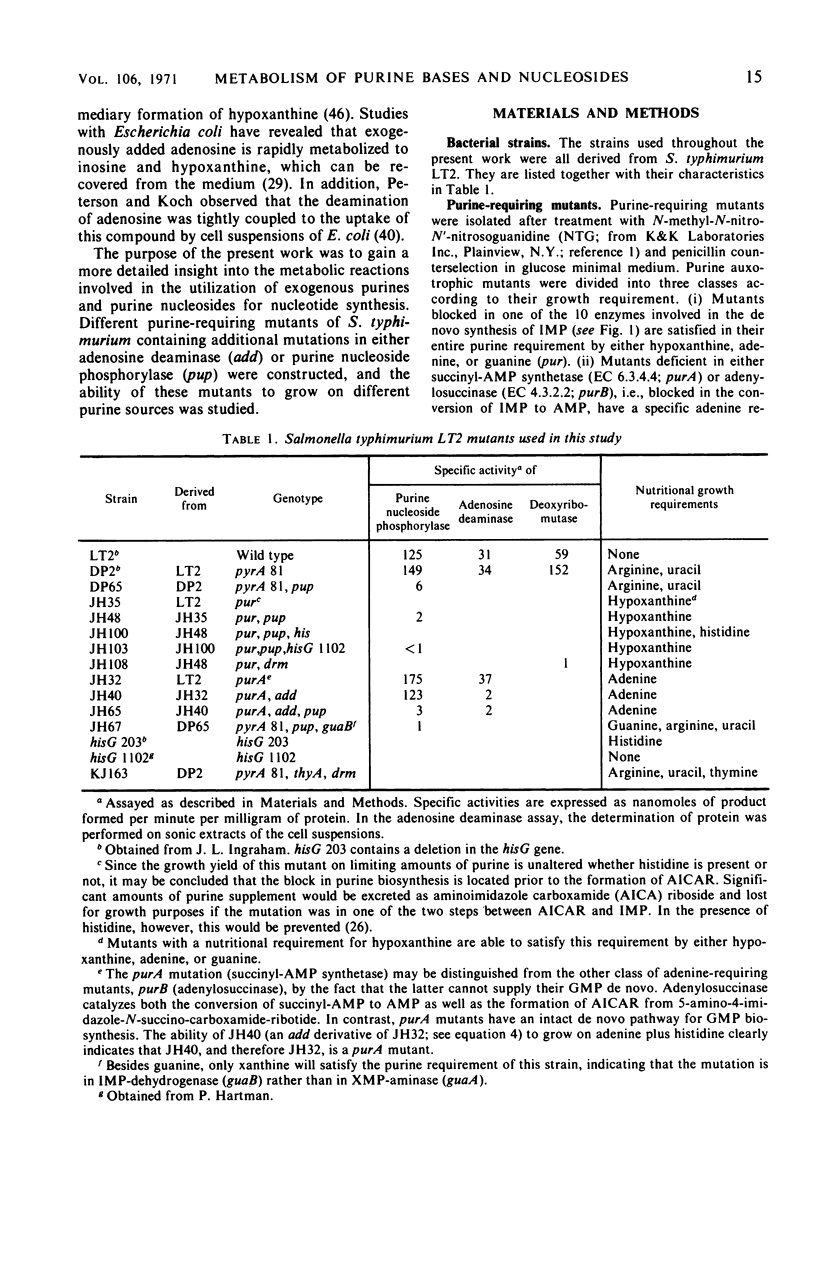
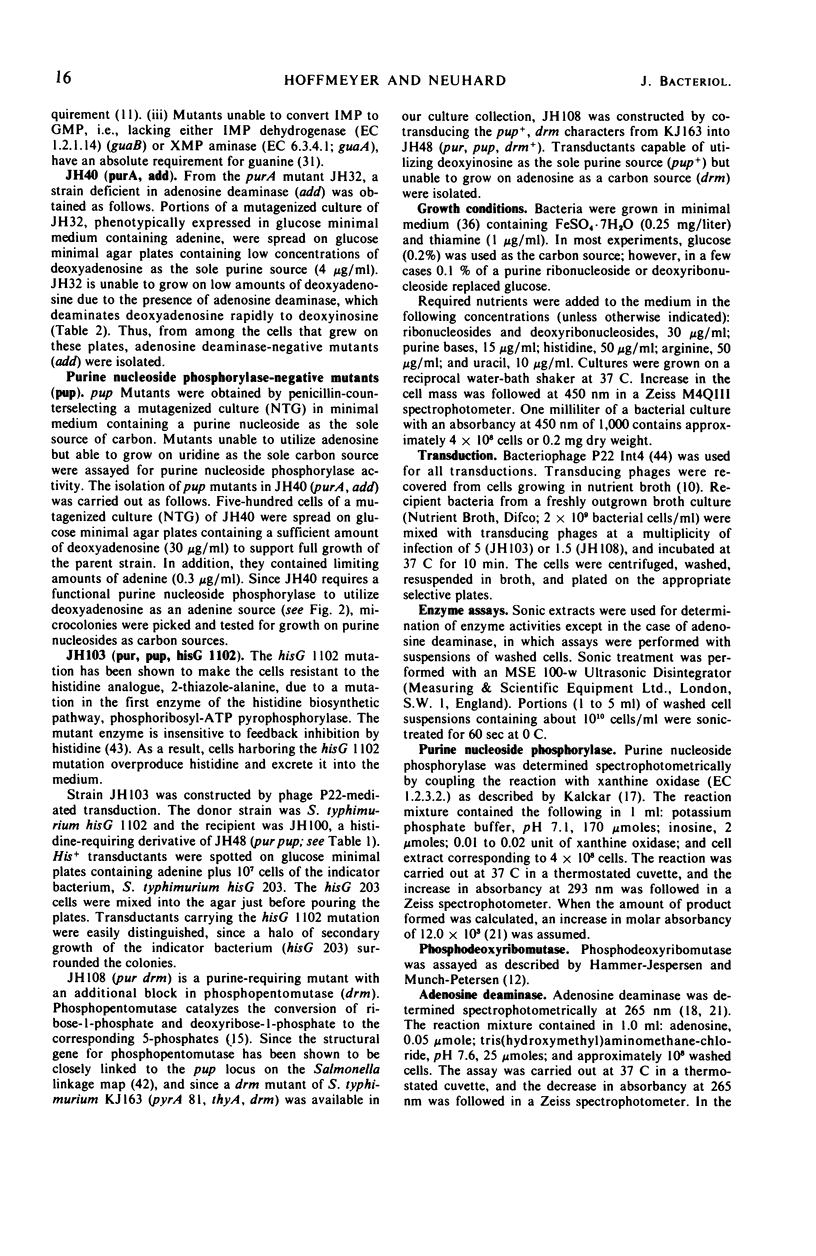
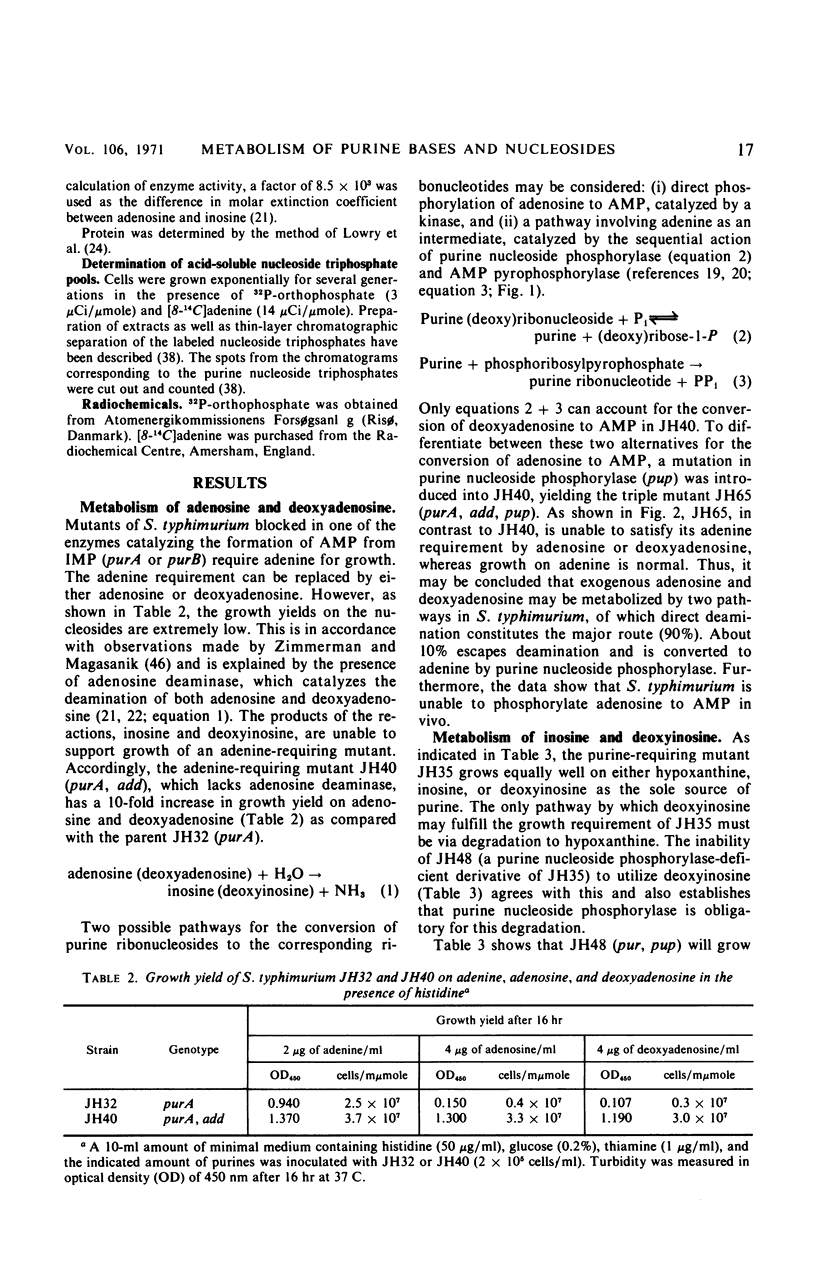
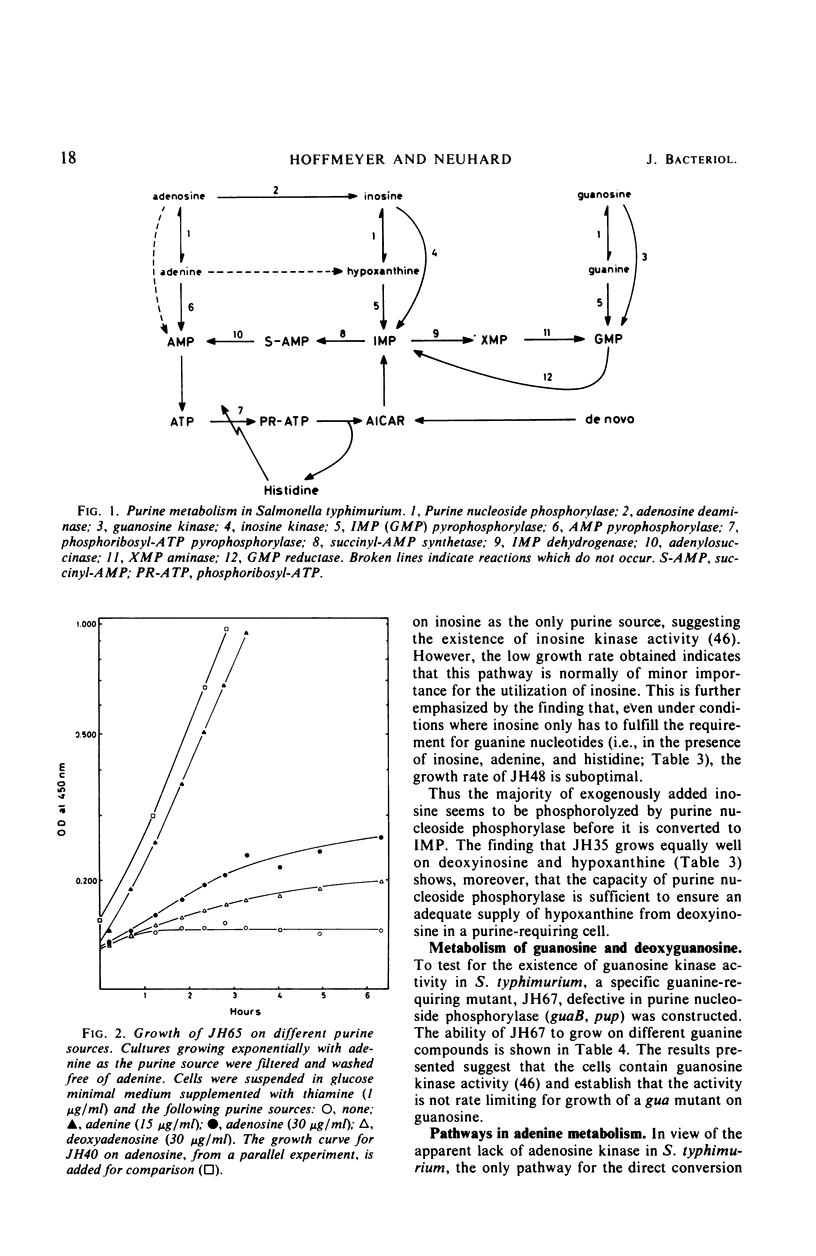
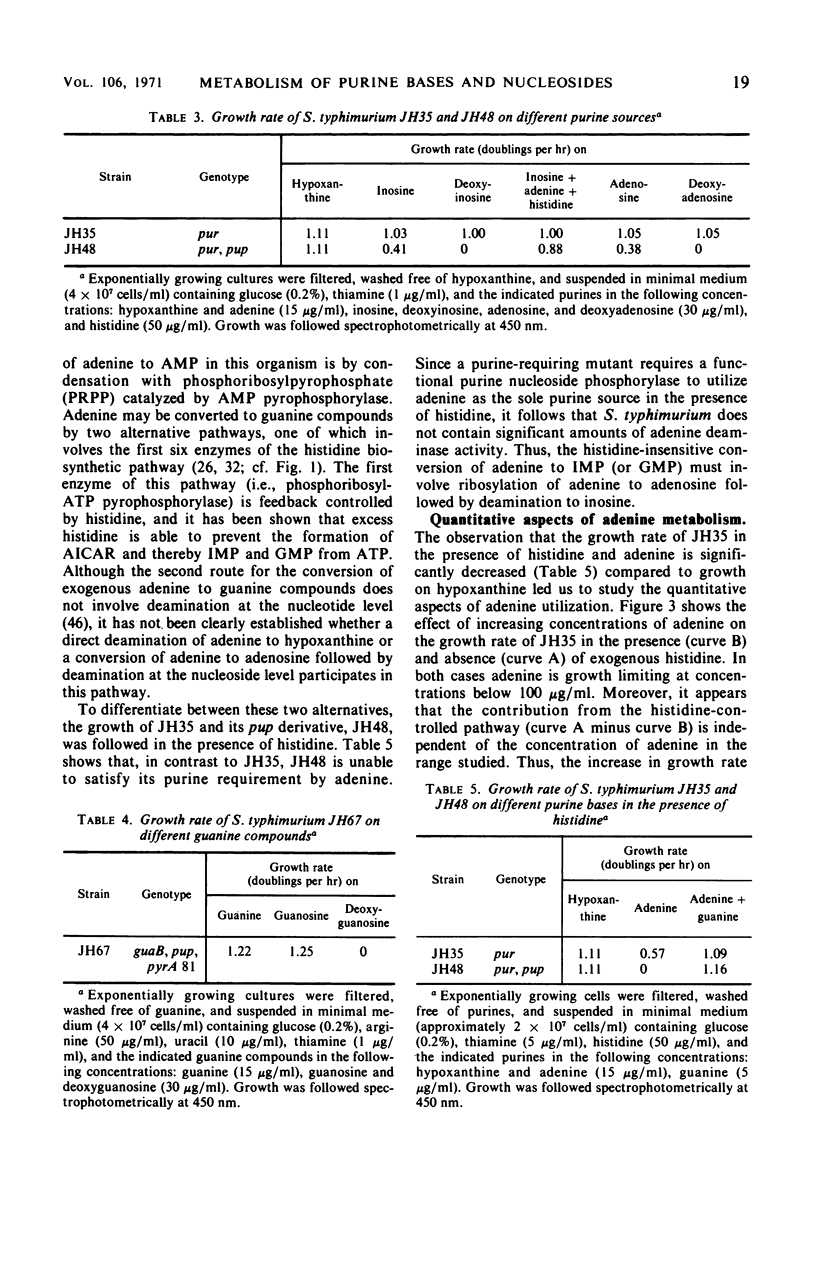
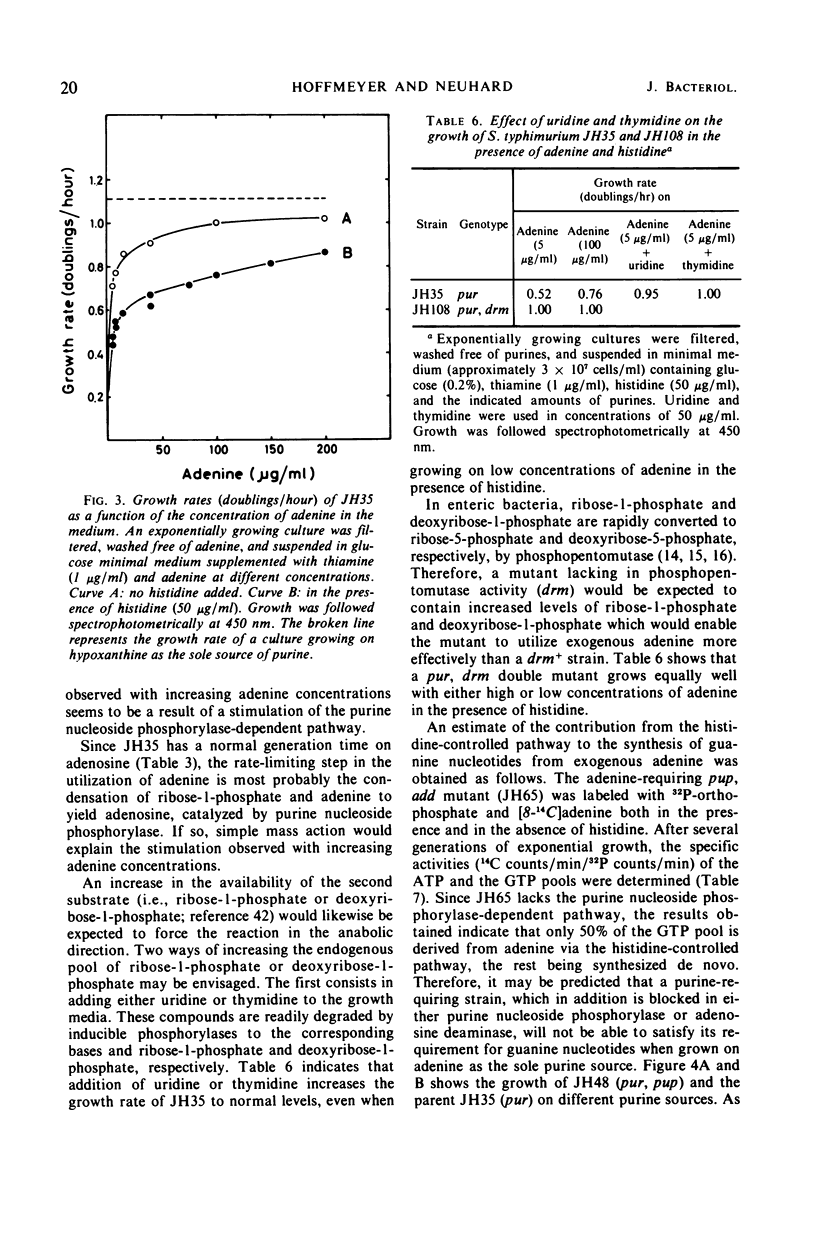
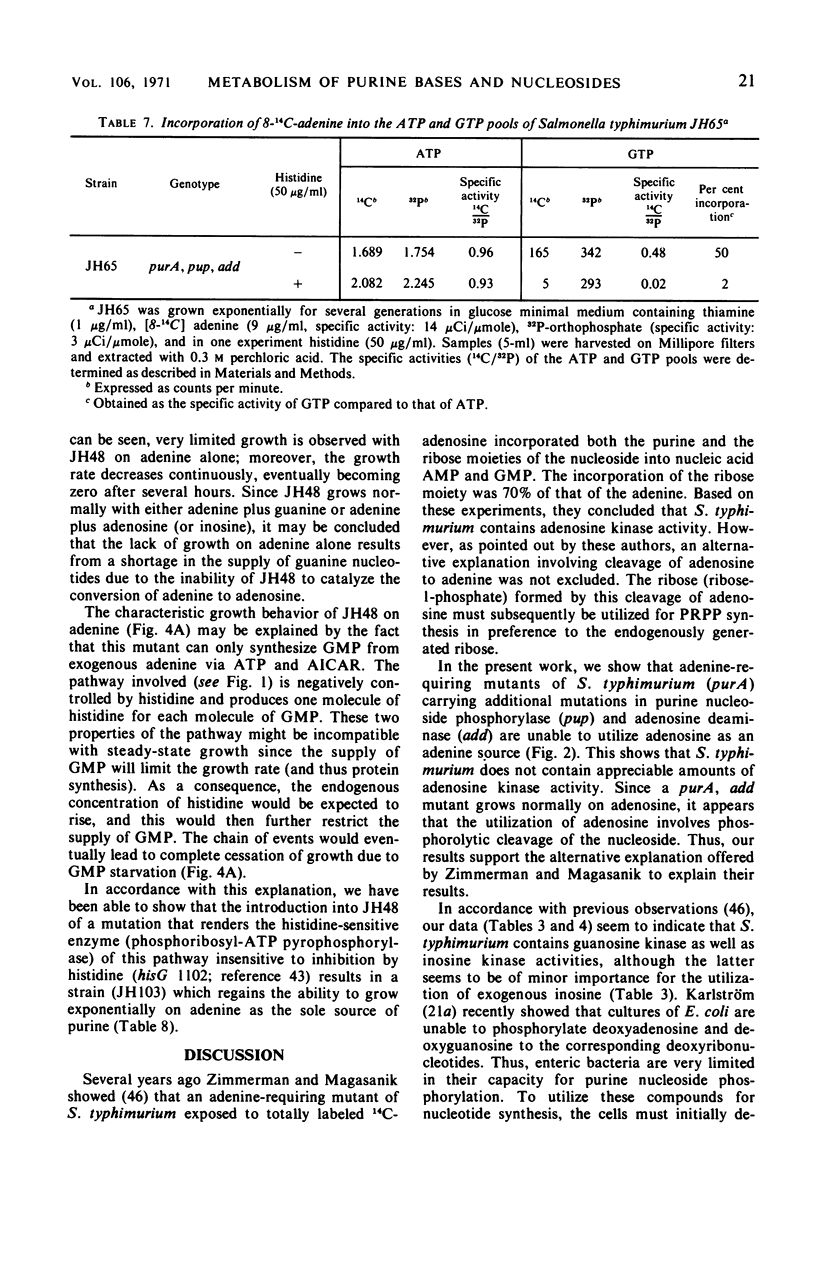
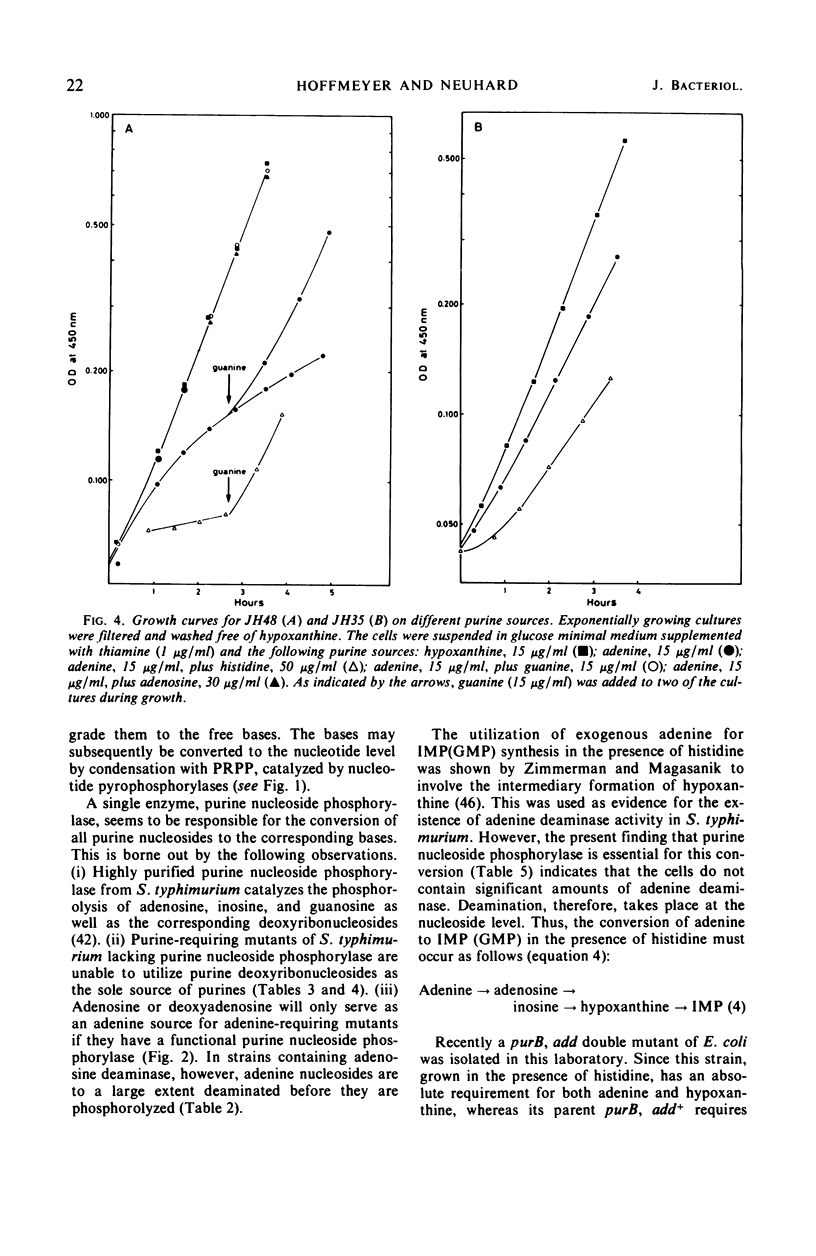
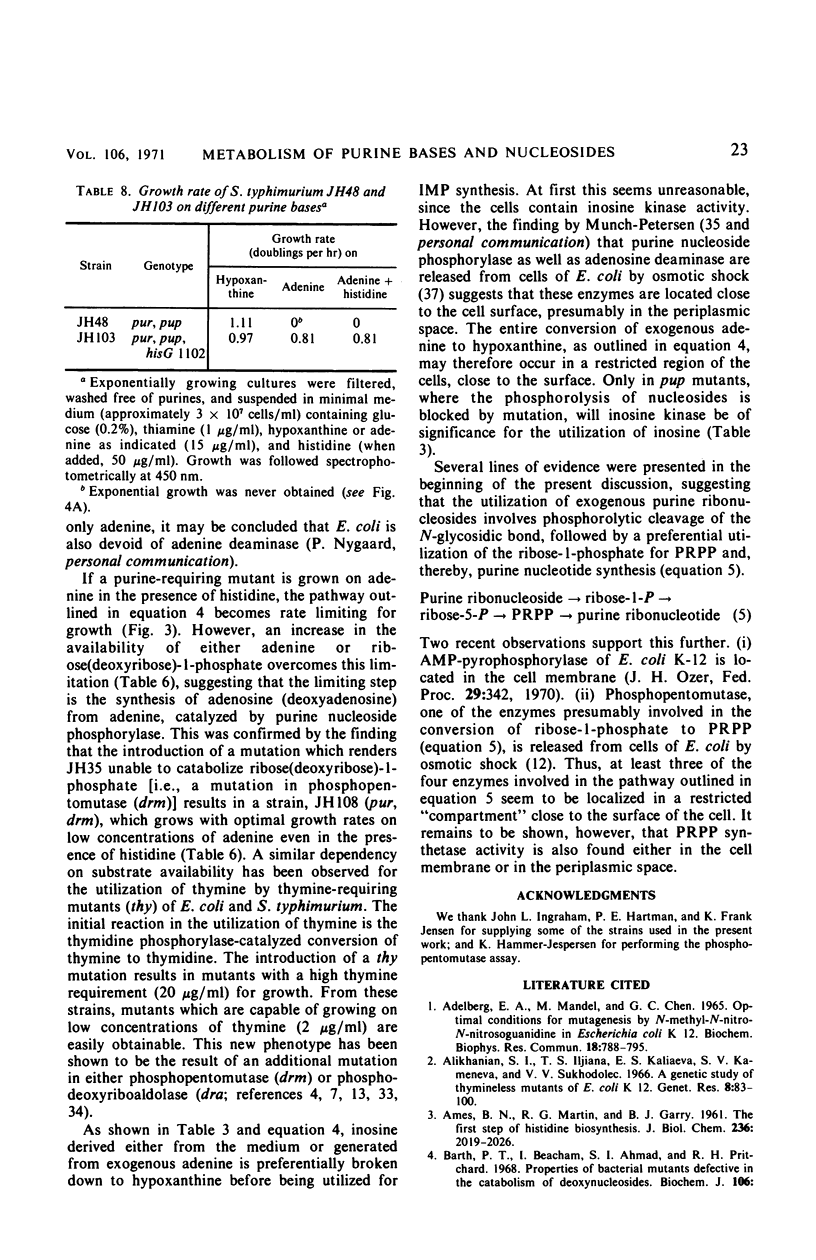
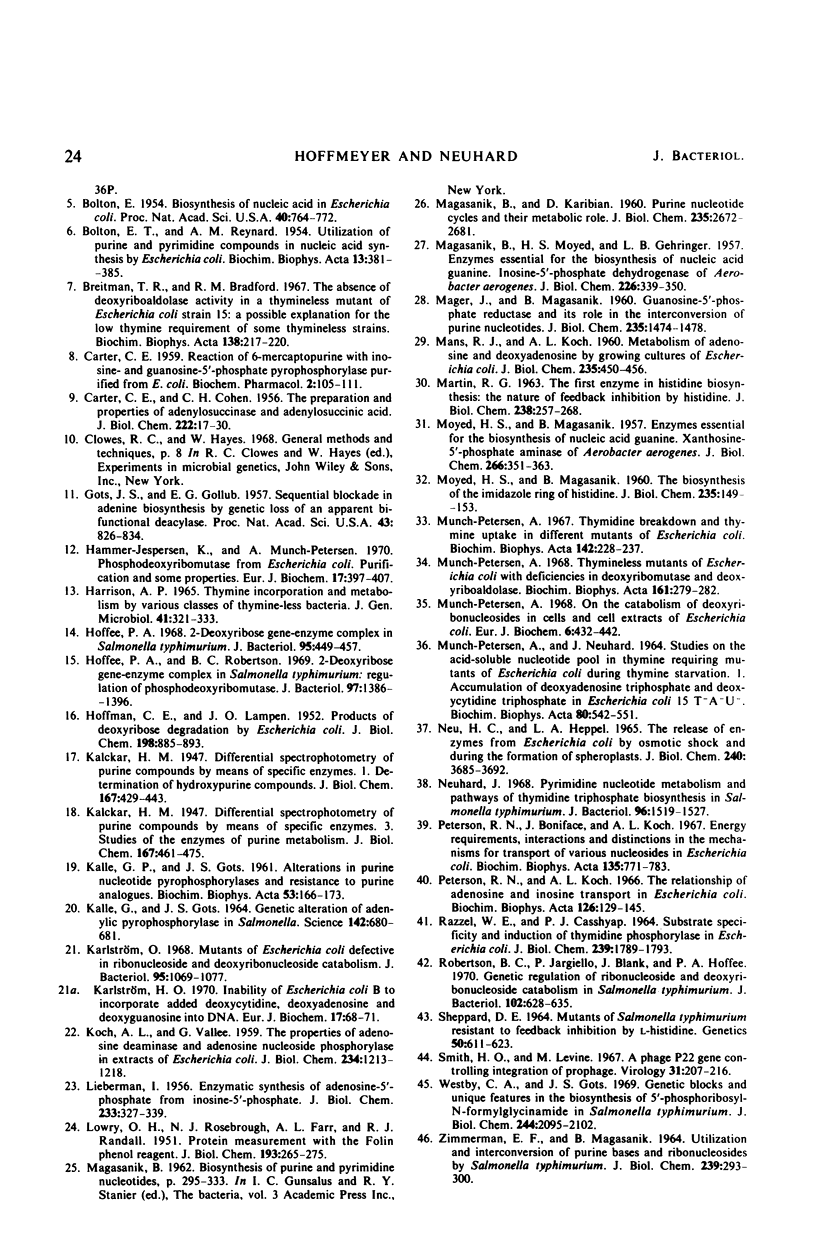
Selected References
These references are in PubMed. This may not be the complete list of references from this article.
- AMES B. N., MARTIN R. G., GARRY B. J. The first step of histidine biosynthesis. J Biol Chem. 1961 Jul;236:2019–2026. [PubMed] [Google Scholar]
- Alikhanian S. I., Iljina T. S., Kaliaeva E. S., Kameneva S. V., Sukhodolec V. V. A genetical study of thymineless mutants of E. coli K12. Genet Res. 1966 Aug;8(1):83–100. doi: 10.1017/s0016672300009939. [DOI] [PubMed] [Google Scholar]
- BOLTON E. T., REYNARD A. M. Utilization of purine and pyrimidine compounds in nucleic acid synthesis by Escherichia coli. Biochim Biophys Acta. 1954 Mar;13(3):381–385. doi: 10.1016/0006-3002(54)90345-5. [DOI] [PubMed] [Google Scholar]
- Bolton E. BIOSYNTHESIS OF NUCLEIC ACID IN ESCHERICHIA COLI. Proc Natl Acad Sci U S A. 1954 Aug;40(8):764–772. doi: 10.1073/pnas.40.8.764. [DOI] [PMC free article] [PubMed] [Google Scholar]
- Breitman T. R., Bradford R. M. The absence of deoxyriboaldolase activity in a thymineless mutant of Escherichia coli strain 15: a possible explanation for the low thymine requirement of some thymineless strains. Biochim Biophys Acta. 1967 Mar 29;138(1):217–220. doi: 10.1016/0005-2787(67)90610-7. [DOI] [PubMed] [Google Scholar]
- CARTER C. E., COHEN L. H. The preparation and properties of adenylosuccinase and adenylosuccinic acid. J Biol Chem. 1956 Sep;222(1):17–30. [PubMed] [Google Scholar]
- CARTER C. E. Reaction of 6-mercaptopurine with inosine- and guanosine-5'-phosphate pyrophosphorylase purified from E. coli. Biochem Pharmacol. 1959 Aug;2:105–111. doi: 10.1016/0006-2952(59)90077-2. [DOI] [PubMed] [Google Scholar]
- Gots J. S., Gollub E. G. SEQUENTIAL BLOCKADE IN ADENINE BIOSYNTHESIS BY GENETIC LOSS OF AN APPARENT BIFUNCTIONAL DEACYLASE. Proc Natl Acad Sci U S A. 1957 Sep 15;43(9):826–834. doi: 10.1073/pnas.43.9.826. [DOI] [PMC free article] [PubMed] [Google Scholar]
- HOFFMANN C. E., LAMPEN J. O. Products of desoxyribose degradation by Escherichia coli. J Biol Chem. 1952 Oct;198(2):885–893. [PubMed] [Google Scholar]
- Hammer-Jespersen K., Munch-Petersen A. Phosphodeoxyribomutase from Escherichia coli. Purification and some properties. Eur J Biochem. 1970 Dec;17(3):397–407. doi: 10.1111/j.1432-1033.1970.tb01179.x. [DOI] [PubMed] [Google Scholar]
- Harrison A. P., Jr Thymine incorporation and metabolism by various classes of thymine-less bacteria. J Gen Microbiol. 1965 Dec;41(3):321–333. doi: 10.1099/00221287-41-3-321. [DOI] [PubMed] [Google Scholar]
- Hoffee P. A. 2-deoxyribose gene-enzyme complex in Salmonella typhimurium. I. Isolation and enzymatic characterization of 2-deoxyribose-negative mutants. J Bacteriol. 1968 Feb;95(2):449–457. doi: 10.1128/jb.95.2.449-457.1968. [DOI] [PMC free article] [PubMed] [Google Scholar]
- Hoffee P. A., Robertson B. C. 2-Deoxyribose gene-enzyme complex in Salmonella typhimurium: regulation of phosphodeoxyribomutase. J Bacteriol. 1969 Mar;97(3):1386–1396. doi: 10.1128/jb.97.3.1386-1396.1969. [DOI] [PMC free article] [PubMed] [Google Scholar]
- KALLE G. P., GOTS J. S. Alterations in purine nucleotide pyrophosphorylases and resistance to purine analogues. Biochim Biophys Acta. 1961 Oct 14;53:166–173. doi: 10.1016/0006-3002(61)90803-4. [DOI] [PubMed] [Google Scholar]
- KALLE G. P., GOTS J. S. GENETIC ALTERATION OF ADENYLIC PYROPHOSPHORYLASE IN SALMONELLA. Science. 1963 Nov 8;142(3593):680–681. doi: 10.1126/science.142.3593.680. [DOI] [PubMed] [Google Scholar]
- KOCH A. L., VALLEE G. The properties of adenosine deaminase and adenosine nucleoside phosphorylase in extracts of Escherichia coli. J Biol Chem. 1959 May;234(5):1213–1218. [PubMed] [Google Scholar]
- Karlström O. Mutants of Escherichia coli defective in ribonucleoside and deoxyribonucleoside catabolism. J Bacteriol. 1968 Mar;95(3):1069–1077. doi: 10.1128/jb.95.3.1069-1077.1968. [DOI] [PMC free article] [PubMed] [Google Scholar]
- LIEBERMAN I. Enzymatic synthesis of adenosine-5'-phosphate from inosine-5'-phosphate. J Biol Chem. 1956 Nov;223(1):327–339. [PubMed] [Google Scholar]
- LOWRY O. H., ROSEBROUGH N. J., FARR A. L., RANDALL R. J. Protein measurement with the Folin phenol reagent. J Biol Chem. 1951 Nov;193(1):265–275. [PubMed] [Google Scholar]
- MAGASANIK B., KARIBIAN D. Purine nucleotide cycles and their metabolic role. J Biol Chem. 1960 Sep;235:2672–2681. [PubMed] [Google Scholar]
- MAGASANIK B., MOYED H. S., GEHRING L. B. Enzymes essential for the biosynthesis of nucleic acid guanine; inosine 5'-phosphate dehydrogenase of Aerobacter aerogenes. J Biol Chem. 1957 May;226(1):339–350. [PubMed] [Google Scholar]
- MAGER J., MAGASANIK B. Guanosine 5'-phosphate reductase and its role in the interconversion of purine nucleotides. J Biol Chem. 1960 May;235:1474–1478. [PubMed] [Google Scholar]
- MANS R. J., KOCH A. L. Metabolism of adenosine and deoxyadenosine by growing cultures of Escherichia coli. J Biol Chem. 1960 Feb;235:450–456. [PubMed] [Google Scholar]
- MOYED H. S., MAGASANIK B. Enzymes essential for the biosynthesis of nucleic acid guanine; xanthosine 5'-phosphate aminase of Aerobacter aerogenes. J Biol Chem. 1957 May;226(1):351–363. [PubMed] [Google Scholar]
- MOYED H. S., MAGASANIK B. The biosynthesis of the imidazole ring of histidine. J Biol Chem. 1960 Jan;235:149–153. [PubMed] [Google Scholar]
- MUNCH-PETERSEN A., NEUHARD J. STUDIES ON THE ACID-SOLUBLE NUCLEOTIDE POOL IN THYMINE-REQUIRING MUTANTS OF ESCHERICHIA COLI DURING THYMINE STARVATION. I. ACCUMULATION OF DEOXYADENOSINE TRIPHOSPHATE IN ESCHERICHIA COLI 15 T-A-U-. Biochim Biophys Acta. 1964 Apr 27;80:542–551. doi: 10.1016/0926-6550(64)90298-1. [DOI] [PubMed] [Google Scholar]
- Munch-Petersen A. On the catabolism of deoxyribonucleosides in cells and cell extracts of Escherichia coli. Eur J Biochem. 1968 Nov;6(3):432–442. doi: 10.1111/j.1432-1033.1968.tb00465.x. [DOI] [PubMed] [Google Scholar]
- Munch-Petersen A. Thymidine breakdown and thymine uptake in different mutants of Escherichia coli. Biochim Biophys Acta. 1967 Jun 20;142(1):228–237. doi: 10.1016/0005-2787(67)90530-8. [DOI] [PubMed] [Google Scholar]
- Munch-Petersen A. Thymineless mutants of Escherichia coli with deficiencies in deoxyribomutase and deoxyriboaldolase. Biochim Biophys Acta. 1968 Jun 18;161(1):279–282. doi: 10.1016/0005-2787(68)90325-0. [DOI] [PubMed] [Google Scholar]
- Neu H. C., Heppel L. A. The release of enzymes from Escherichia coli by osmotic shock and during the formation of spheroplasts. J Biol Chem. 1965 Sep;240(9):3685–3692. [PubMed] [Google Scholar]
- Neuhard J. Pyrimidine nucleotide metabolism and pathways of thymidine triphosphate biosynthesis in Salmonella typhimurium. J Bacteriol. 1968 Nov;96(5):1519–1527. doi: 10.1128/jb.96.5.1519-1527.1968. [DOI] [PMC free article] [PubMed] [Google Scholar]
- Peterson R. N., Boniface J., Koch A. L. Energy requirements, interactions and distinctions in the mechanisms for transport of various nucleosides in Escherichia coli. Biochim Biophys Acta. 1967 Sep 9;135(4):771–783. doi: 10.1016/0005-2736(67)90108-3. [DOI] [PubMed] [Google Scholar]
- Peterson R. N., Koch A. L. The relationship of adenosine and inosine transport in Escherichia coli. Biochim Biophys Acta. 1966 Sep 5;126(1):129–145. doi: 10.1016/0926-6585(66)90043-4. [DOI] [PubMed] [Google Scholar]
- RAZZELL W. E., CASSHYAP P. SUBSTRATE SPECIFICITY AND INDUCTION OF THYMIDINE PHOSPHORYLASE IN ESCHERICHIA COLI. J Biol Chem. 1964 Jun;239:1789–1793. [PubMed] [Google Scholar]
- Robertson B. C., Jargiello P., Blank J., Hoffee P. A. Genetic regulation of ribonucleoside and deoxyribonucleoside catabolism in Salmonella typhimurium. J Bacteriol. 1970 Jun;102(3):628–635. doi: 10.1128/jb.102.3.628-635.1970. [DOI] [PMC free article] [PubMed] [Google Scholar]
- SHEPPARD D. E. MUTANTS OF SALMONELLA TYPHIMURIUM RESISTANT TO FEEDBACK INHIBITION BY L-HISTIDINE. Genetics. 1964 Oct;50:611–623. doi: 10.1093/genetics/50.4.611. [DOI] [PMC free article] [PubMed] [Google Scholar]
- Simkin J. L., Jamieson J. C. Studies on the nature of microsome-bound substances involved in the biosynthesis of acidic glycoproteins of guinea-pig serum. Biochem J. 1968 Jan;106(1):23–28. doi: 10.1042/bj1060023. [DOI] [PMC free article] [PubMed] [Google Scholar]
- Smith H. O., Levine M. A phage P22 gene controlling integration of prophage. Virology. 1967 Feb;31(2):207–216. doi: 10.1016/0042-6822(67)90164-x. [DOI] [PubMed] [Google Scholar]
- Westby C. A., Gots J. S. Genetic blocks and unique features in the biosynthesis of 5'-phosphoribosyl-N-formylglycinamide in Salmonella typhimurium. J Biol Chem. 1969 Apr 25;244(8):2095–2102. [PubMed] [Google Scholar]
- ZIMMERMAN E. F., MAGASANIK B. UTILIZATION AND INTERCONVERSION OF PURINE BASES AND RIBONUCLEOSIDES BY SALMONELLA TYPHIMURIUM. J Biol Chem. 1964 Jan;239:293–300. [PubMed] [Google Scholar]