Abstract
The nicotinamide adenine dinucleotide phosphate-specific isocitrate dehydrogenase of Halobacterium cutirubrum is rapidly inactivated at low NaCl levels. As much as 75% of the initial activity can be restored by dialyzing the inactive enzyme against 4 m NaCl. A mixture of 4 mm isocitrate and 10 mm MnCl2 gives the same protection as 4 m NaCl but does not replace the NaCl requirement for reactivation. The reactivated and native enzymes have identical sedimentation rates on sucrose gradients, electrophoretic mobilities on polyacrylamide gels, and elution rates from Sephadex G-200. However, there are distinct differences between the active and inactive forms of the enzyme. Compared with the active enzyme, the inactive protein has a lower sedimentation rate, a lower electrophoretic mobility, and a faster elution rate from Sephadex. These differences indicate that inactivation causes a major conformational change in the protein. Presumably, the removal of NaCl permits the enzyme to expand into a less dense, inactive form. The isocitrate dehydrogenase was purified 69-fold by a procedure involving the following steps. When the enzyme is selectively protected with isocitrate and MnCl2 at low ionic strength, most of the contaminating proteins are precipitated with (NH4)2SO4 at 0.9 saturation. The enzyme in the supernatant fluid is then inactivated at low NaCl levels, precipitated with 0.5 saturated (NH4)2SO4, and reactivated with 4 m NaCl. Minor impurities are removed by gel filtration on Sephadex G-200. The resulting preparation is more than 95% pure as judged by disc electrophoresis.
Full text
PDF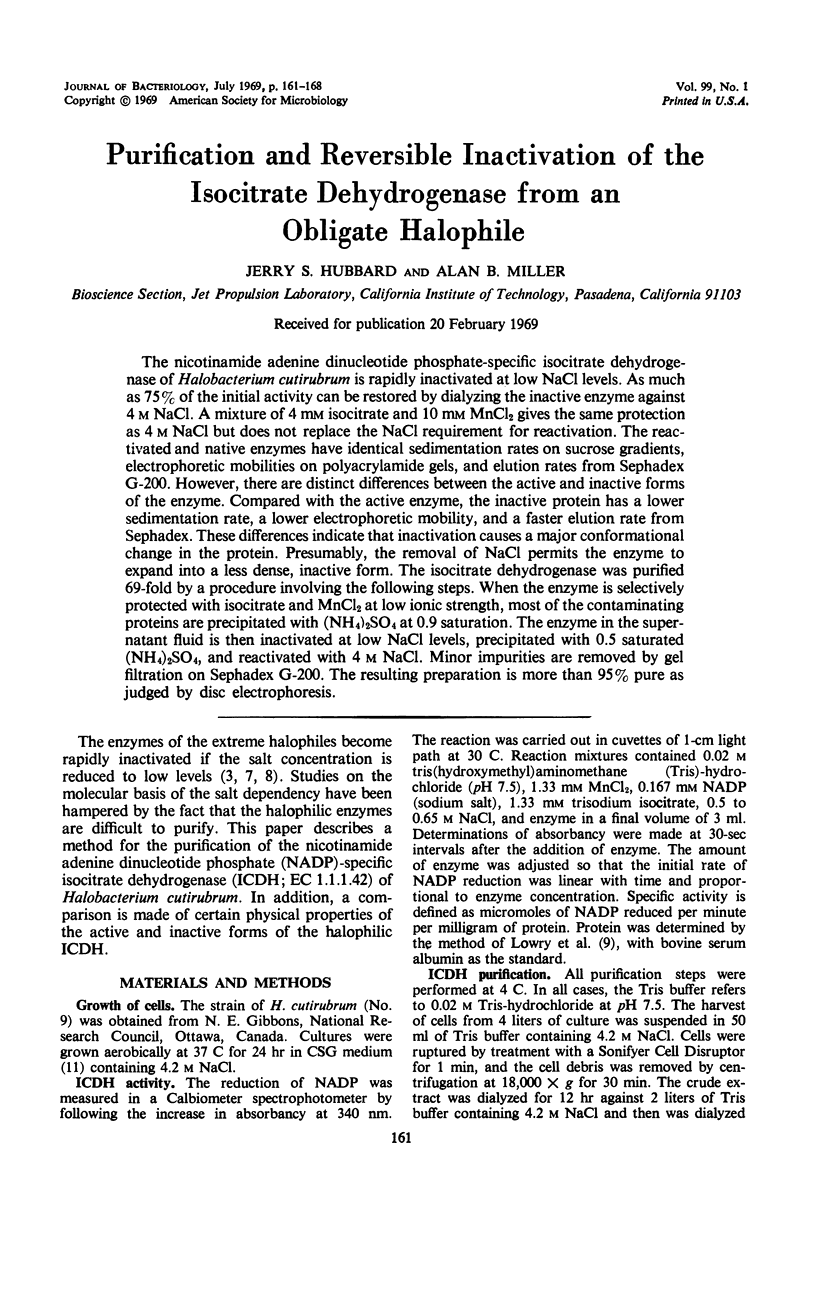
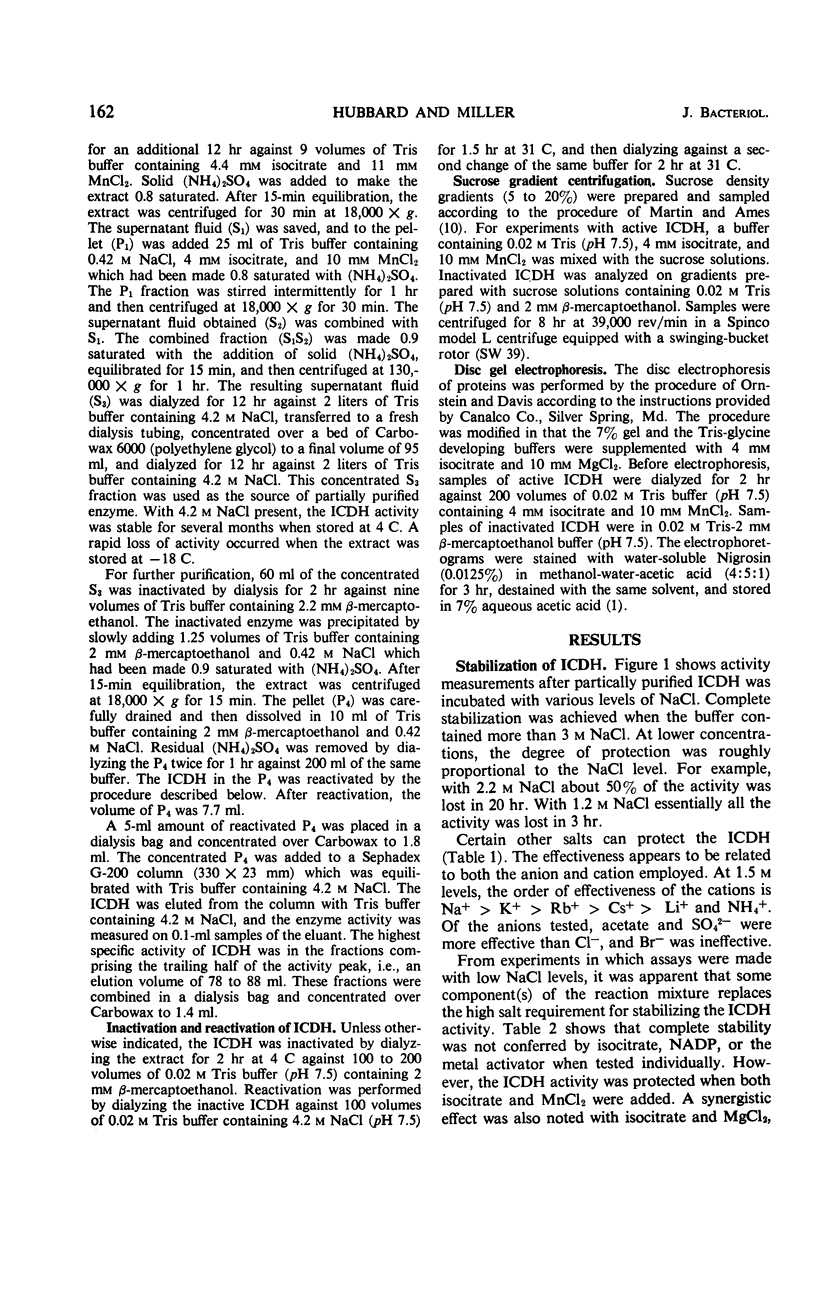
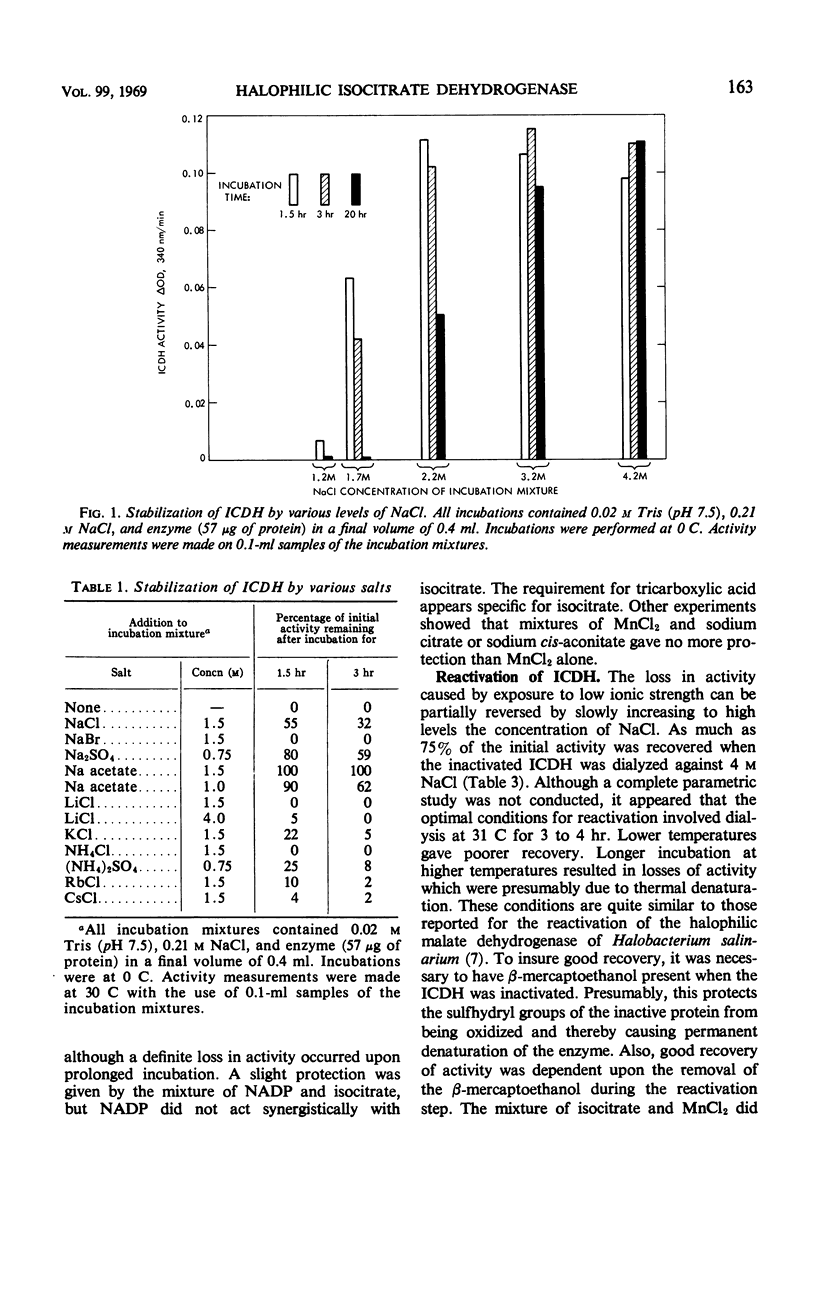
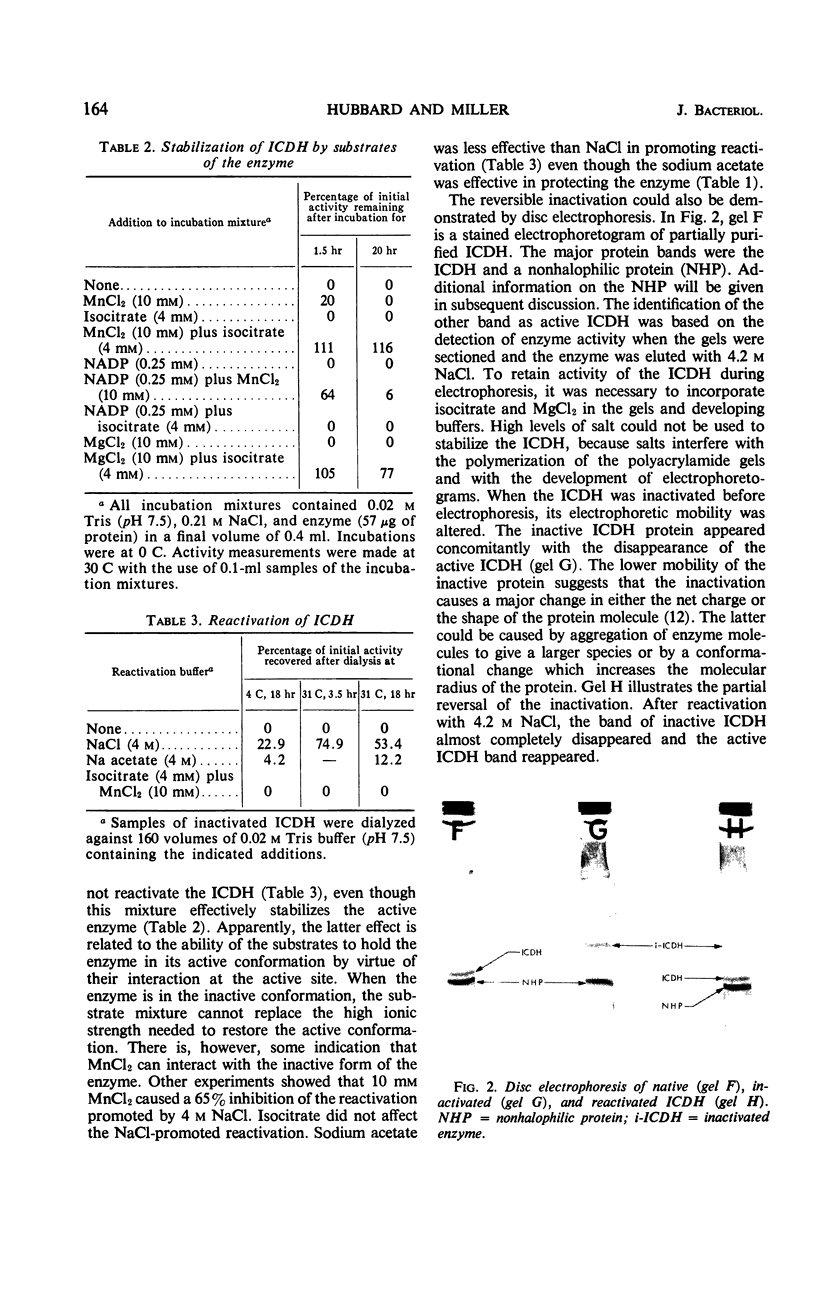
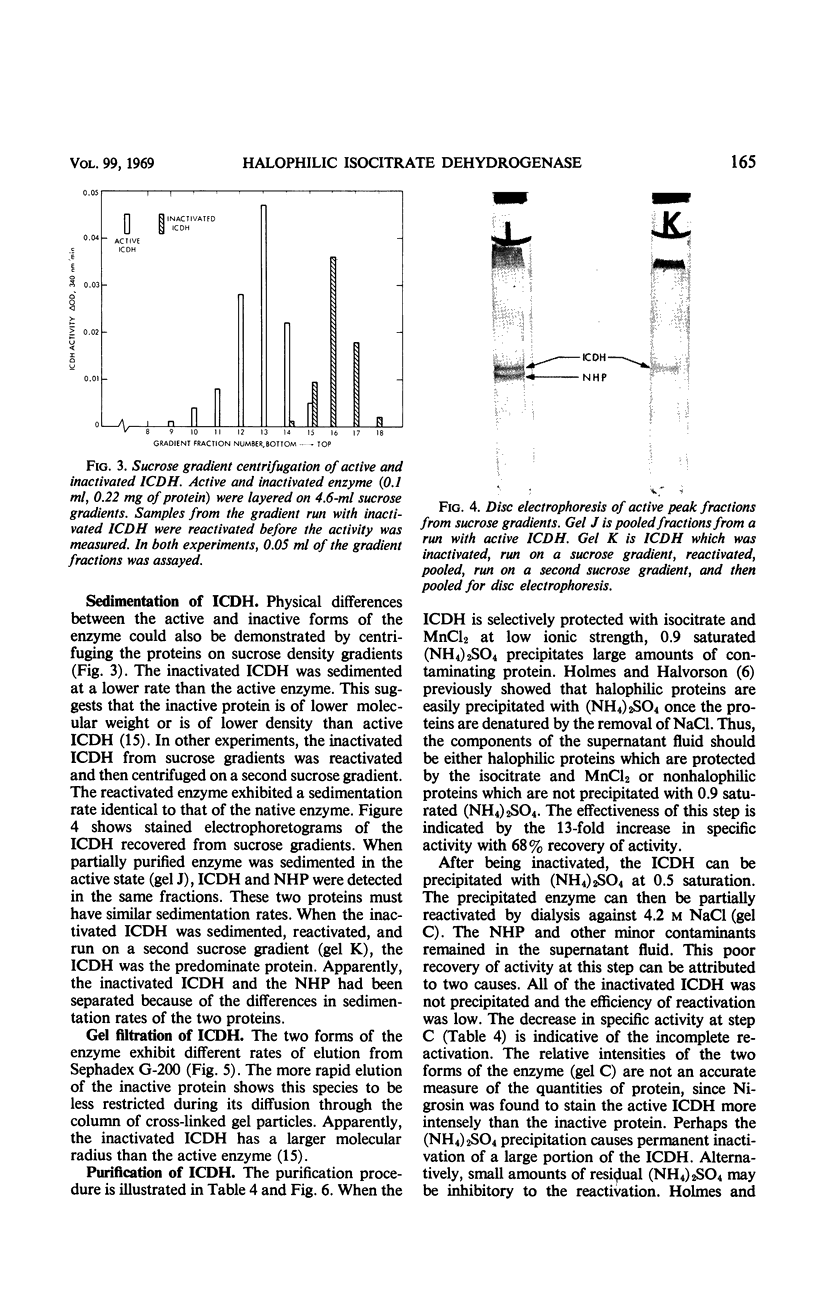
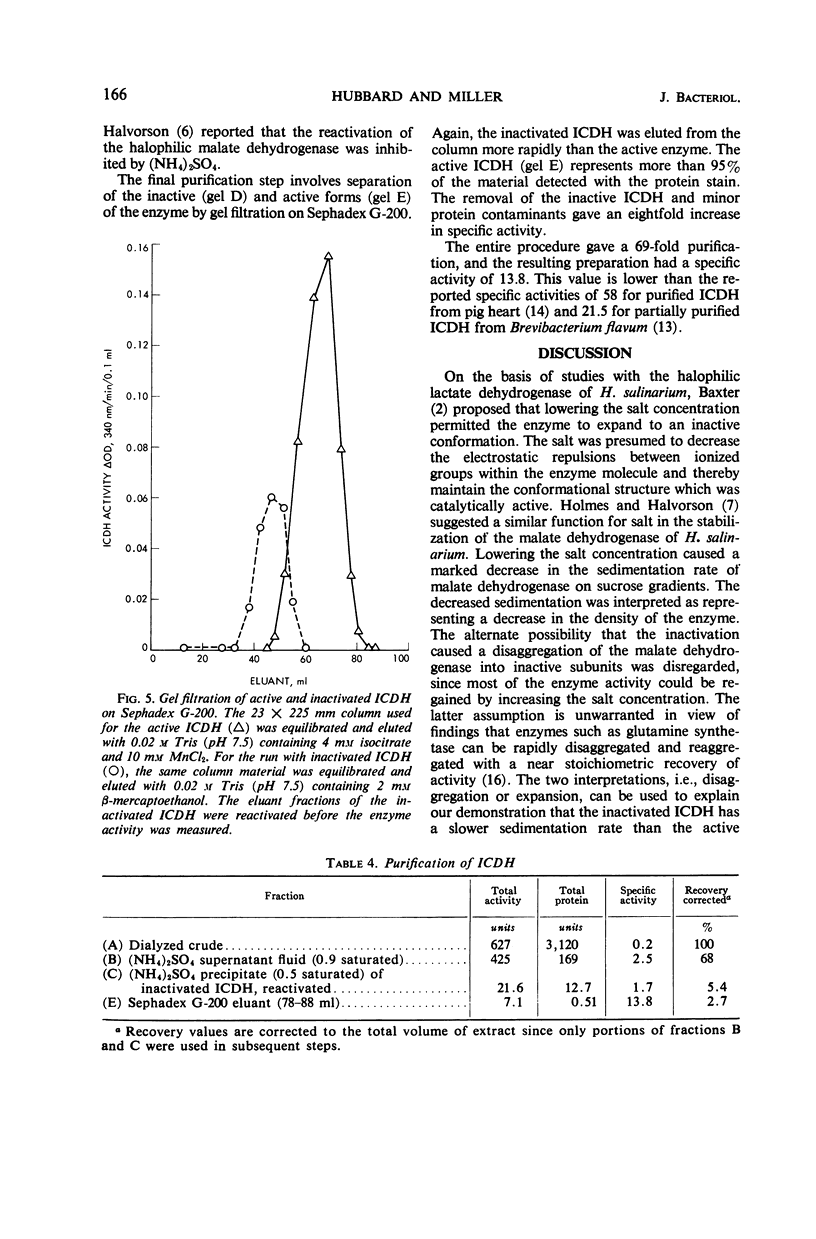
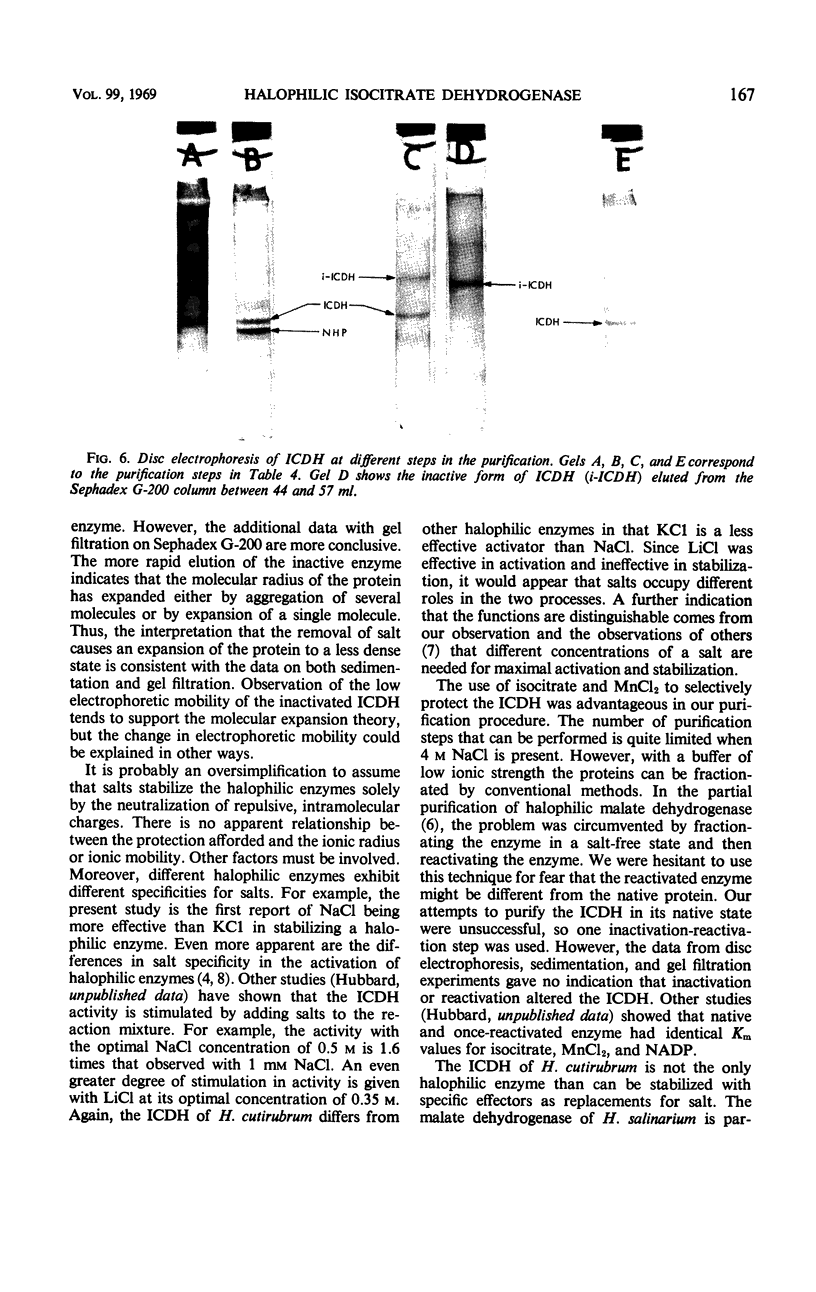
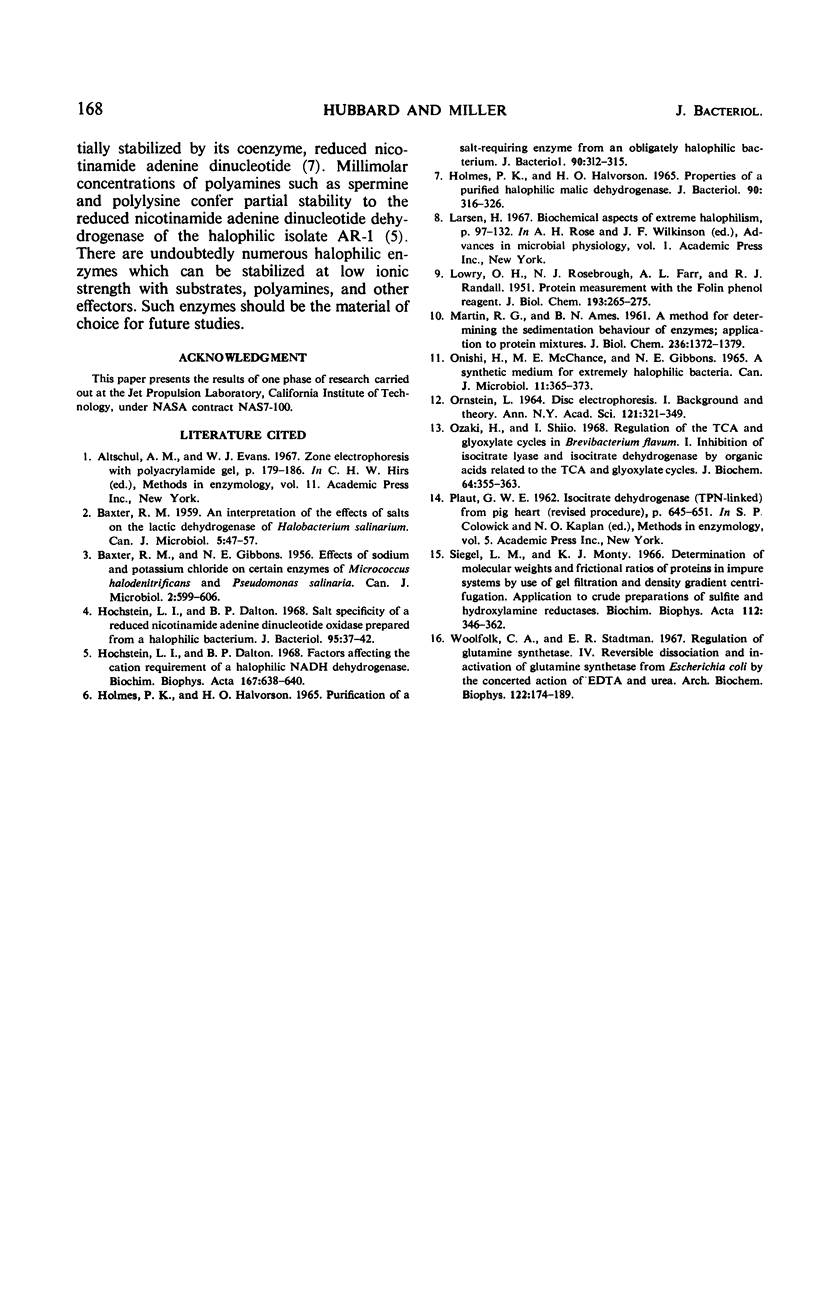
Images in this article
Selected References
These references are in PubMed. This may not be the complete list of references from this article.
- BAXTER R. M. An interpretation of the effects of salts on the lactic dehydrogenase of Halobacterium salinarium. Can J Microbiol. 1959 Feb;5(1):47–57. doi: 10.1139/m59-006. [DOI] [PubMed] [Google Scholar]
- BAXTER R. M., GIBBONS N. E. Effects of sodium and potassium chloride on certain enzymes of Micrococcus halodenitrificans and Pseudomonas salinaria. Can J Microbiol. 1956 Oct;2(6):599–606. doi: 10.1139/m56-072. [DOI] [PubMed] [Google Scholar]
- HOLMES P. K., HALVORSON H. O. PROPERTIES OF A PURIFIED HALOPHILIC MALIC DEHYDROGENASE. J Bacteriol. 1965 Aug;90:316–326. doi: 10.1128/jb.90.2.316-326.1965. [DOI] [PMC free article] [PubMed] [Google Scholar]
- HOLMES P. K., HALVORSON H. O. PURIFICATION OF A SALT-REQUIRING ENZYME FROM AN OBLIGATELY HALOPHILIC BACTERIUM. J Bacteriol. 1965 Aug;90:312–315. doi: 10.1128/jb.90.2.312-315.1965. [DOI] [PMC free article] [PubMed] [Google Scholar]
- Hochstein L. I., Dalton B. P. Factors affecting the cation requirement of a halophilic NADH dehydrogenase. Biochim Biophys Acta. 1968 Nov 19;167(3):638–640. doi: 10.1016/0005-2744(68)90062-4. [DOI] [PubMed] [Google Scholar]
- Hochstein L. I., Dalton B. P. Salt specificity of a reduced nicotinamide adenine dinucleotide oxidase prepared from a halophilic bacterium. J Bacteriol. 1968 Jan;95(1):37–42. doi: 10.1128/jb.95.1.37-42.1968. [DOI] [PMC free article] [PubMed] [Google Scholar]
- LOWRY O. H., ROSEBROUGH N. J., FARR A. L., RANDALL R. J. Protein measurement with the Folin phenol reagent. J Biol Chem. 1951 Nov;193(1):265–275. [PubMed] [Google Scholar]
- MARTIN R. G., AMES B. N. A method for determining the sedimentation behavior of enzymes: application to protein mixtures. J Biol Chem. 1961 May;236:1372–1379. [PubMed] [Google Scholar]
- ONISHI H., MCCANCE E., GIBBONS N. E. A SYNTHETIC MEDIUM FOR EXTREMELY HALOPHILIC BACTERIA. Can J Microbiol. 1965 Apr;11:365–373. doi: 10.1139/m65-044. [DOI] [PubMed] [Google Scholar]
- ORNSTEIN L. DISC ELECTROPHORESIS. I. BACKGROUND AND THEORY. Ann N Y Acad Sci. 1964 Dec 28;121:321–349. doi: 10.1111/j.1749-6632.1964.tb14207.x. [DOI] [PubMed] [Google Scholar]
- Ozaki H., Shiio I. Regulation of the TCA and glyoxylate cycles in Brevibacterium flavum. I. Ingibition of isocitrate lyase and isocitrate dehydrogenase by organic acids related to the TCA and glyoxylate cycles. J Biochem. 1968 Sep;64(3):355–363. doi: 10.1093/oxfordjournals.jbchem.a128902. [DOI] [PubMed] [Google Scholar]
- Siegel L. M., Monty K. J. Determination of molecular weights and frictional ratios of proteins in impure systems by use of gel filtration and density gradient centrifugation. Application to crude preparations of sulfite and hydroxylamine reductases. Biochim Biophys Acta. 1966 Feb 7;112(2):346–362. doi: 10.1016/0926-6585(66)90333-5. [DOI] [PubMed] [Google Scholar]
- Woolfolk C. A., Stadtman E. R. Regulation of glutamine synthetase. IV. Reversible dissociation and inactivation of glutamine synthetase from Escherichia coli by the concerted action of EDTA and urea. Arch Biochem Biophys. 1967 Oct;122(1):174–189. doi: 10.1016/0003-9861(67)90137-3. [DOI] [PubMed] [Google Scholar]