Abstract
Cell-free extracts from aerobically grown Streptococcus agalactiae cells possess a reduced nicotinamide adenine dinucleotide (NADH) oxidase which is linked to oxygen. It is inhibited by cyanide, although cytochromes evidently are not involved. Adenosine triphosphate (ATP) formation occurs during the reaction, but 66 to 75% of the total ATP is formed nonoxidatively. The remaining 25 to 35% of the ATP formation is related to the oxidation of NADH. The formation of ATP in the oxidative reaction can be prevented by excluding oxygen or adding cyanide to prevent NADH oxidation. It can also be prevented by adding methylene blue or pyruvate, which bypasses electron transport to oxygen, but does not interfere with NADH oxidation. Potential sources of ATP, such as glycolysis, the pyruvate oxidase reaction, or the oxidative pentose cycle, are not present, and the high nonoxidative ATP formation is ascribed to the adenylate kinase reaction. The reaction requires adenosine diphosphate (ADP) as a phosphate acceptor. NADH oxidation is independent of ADP. Antimycin A, amytal, and 2,4-dinitrophenol decreased, but did not prevent, oxidative formation of ATP. P:O ratios ranged from 0.15 to 0.25. All of the oxidative activity was in the soluble portion of the cell-free extracts.
Full text
PDF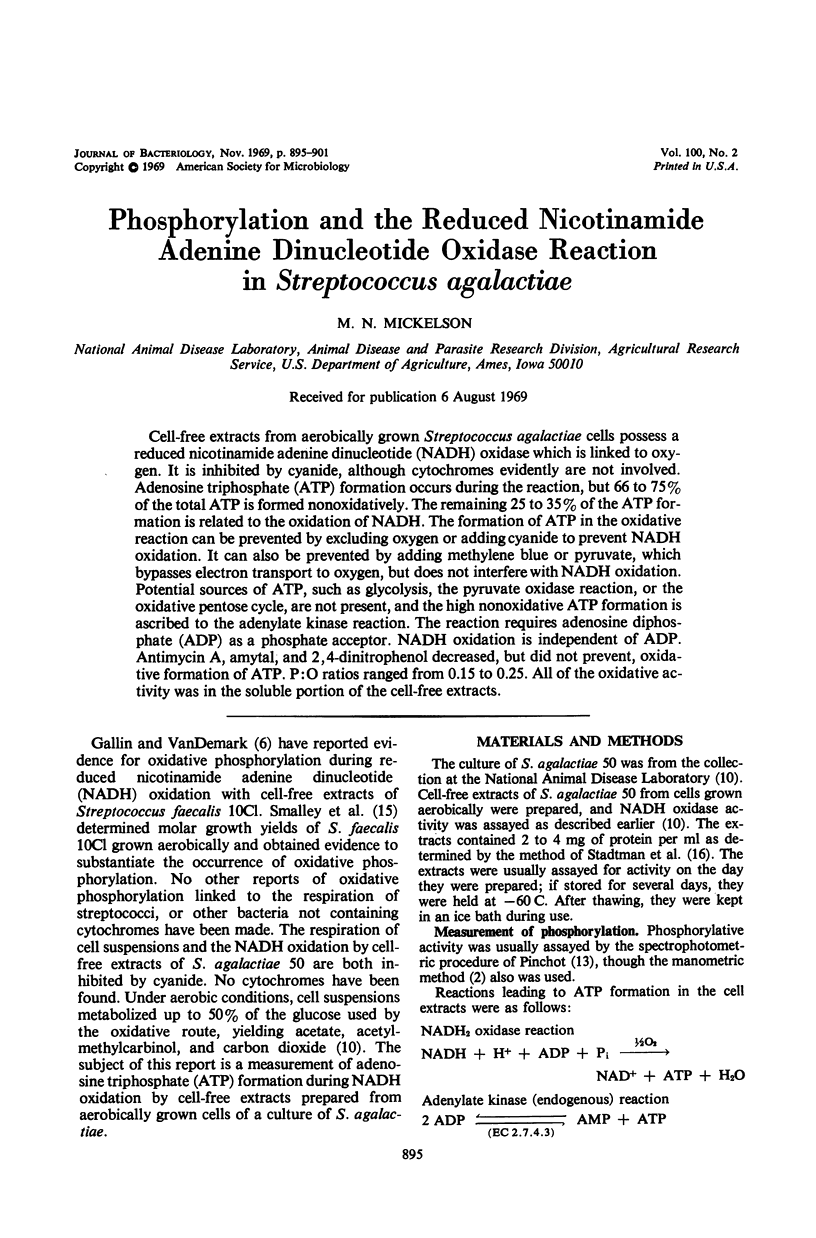
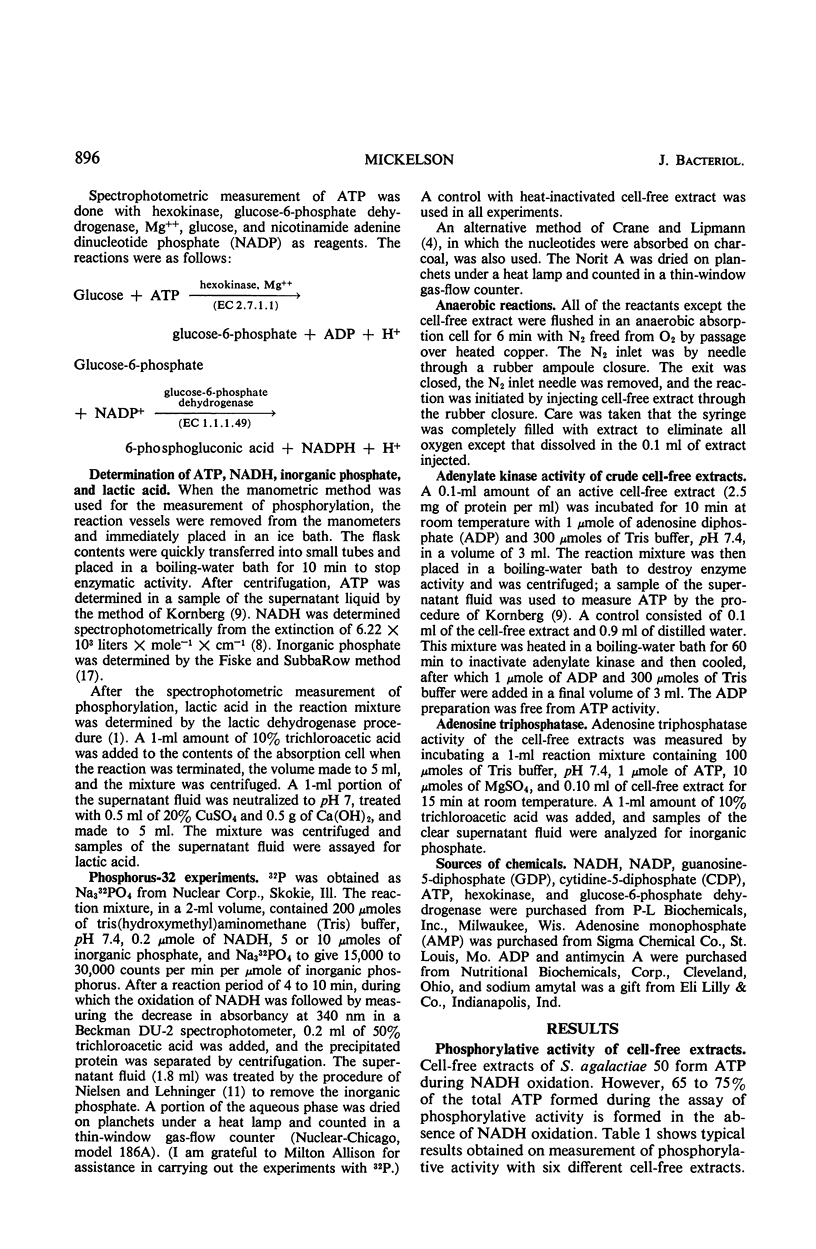
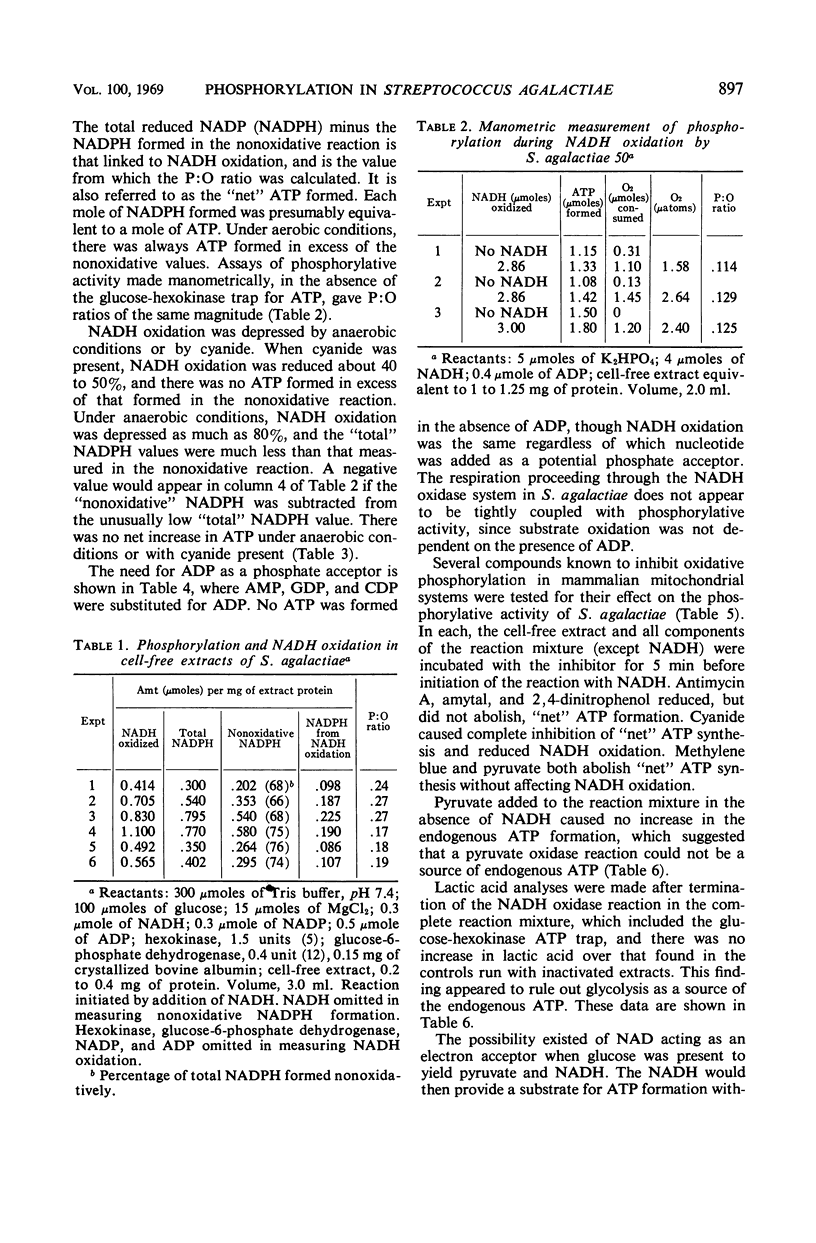
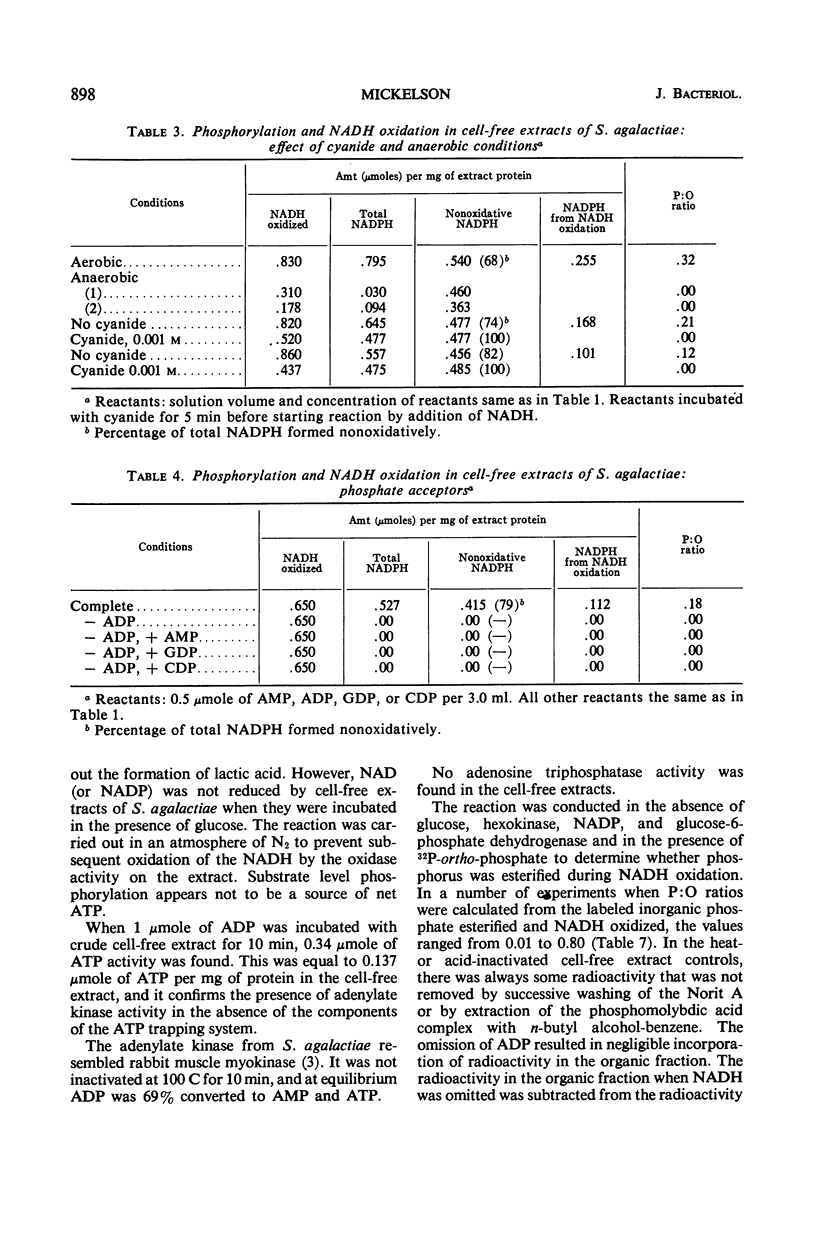
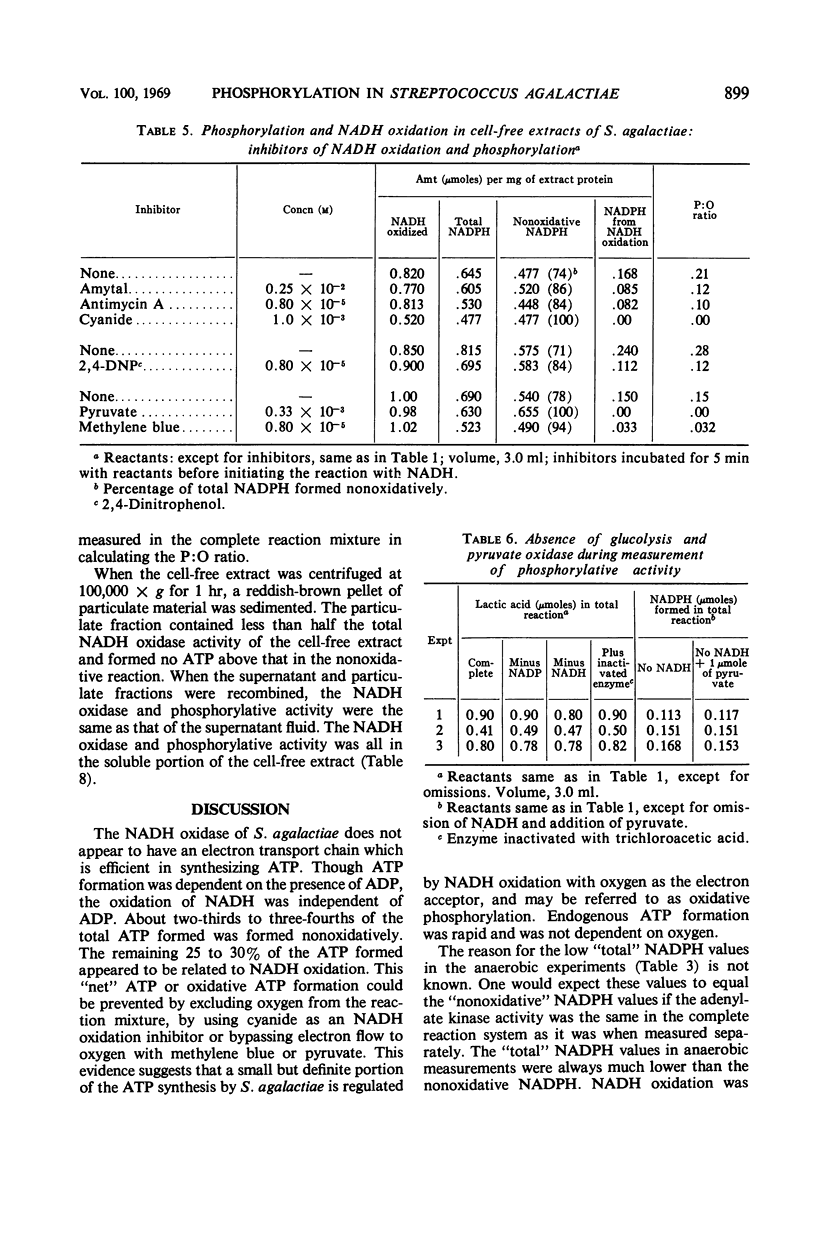
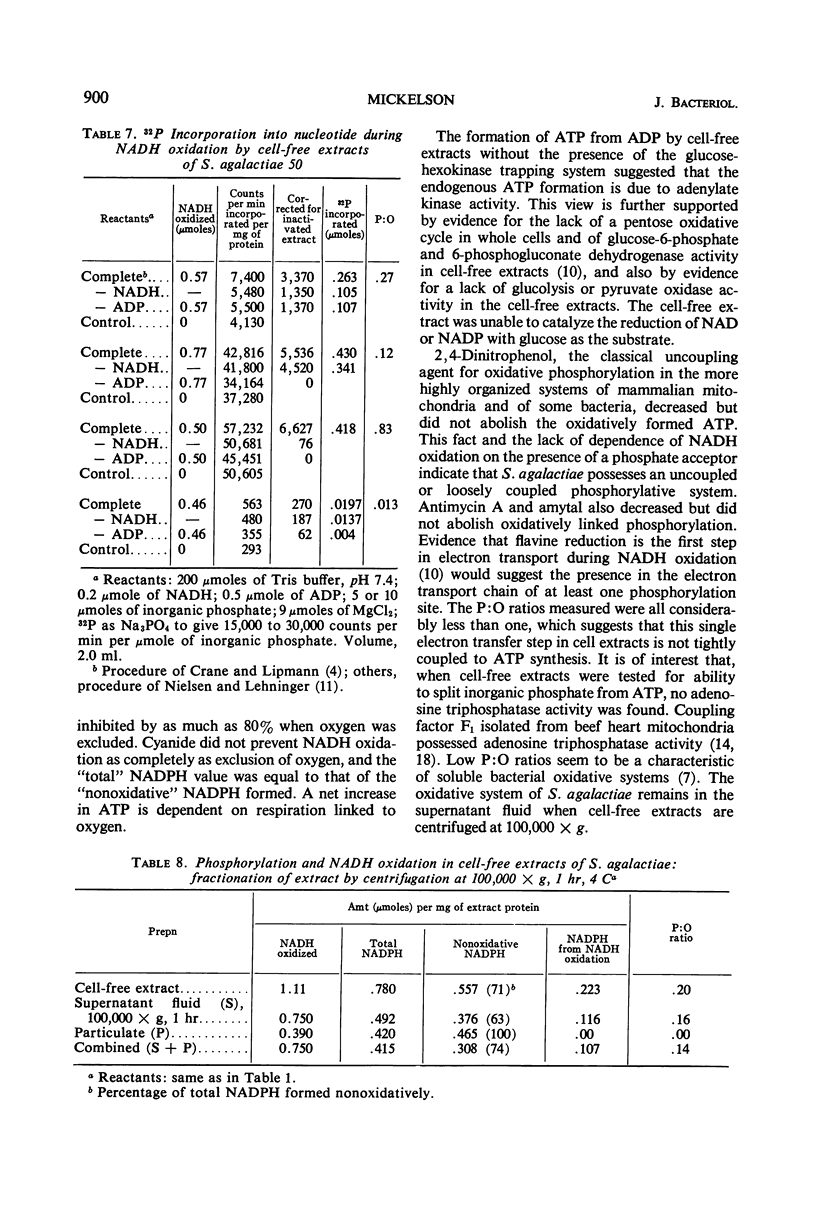
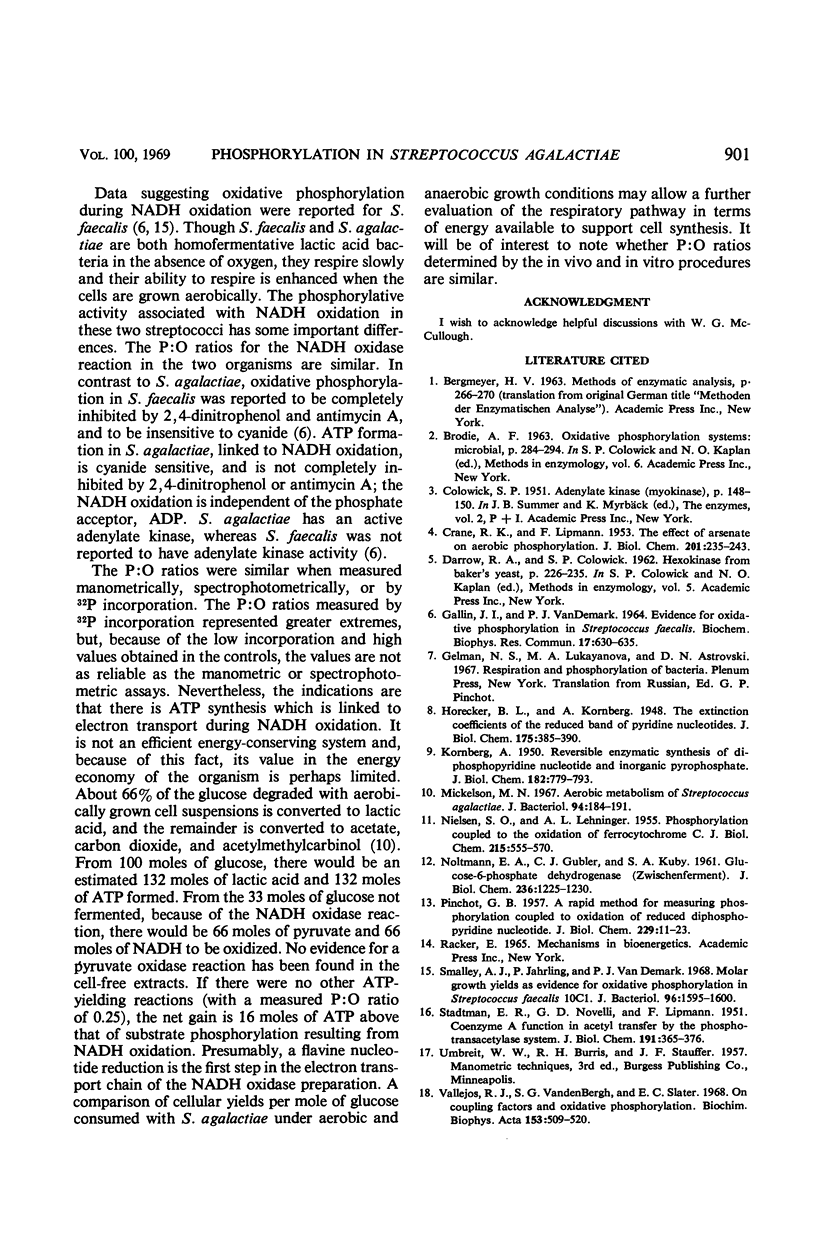
Selected References
These references are in PubMed. This may not be the complete list of references from this article.
- CRANE R. K., LIPMANN F. The effect of arsenate on aerobic phosphorylation. J Biol Chem. 1953 Mar;201(1):235–243. [PubMed] [Google Scholar]
- Mickelson M. N. Aerobic metabolism of Streptococcus agalactiae. J Bacteriol. 1967 Jul;94(1):184–191. doi: 10.1128/jb.94.1.184-191.1967. [DOI] [PMC free article] [PubMed] [Google Scholar]
- NIELSEN S. O., LEHNINGER A. L. Phosphorylation coupled to the oxidation of ferrocytochrome c. J Biol Chem. 1955 Aug;215(2):555–570. [PubMed] [Google Scholar]
- NOLTMANN E. A., GUBLER C. J., KUBY S. A. Glucose 6-phosphate dehydrogenase (Zwischenferment). I. Isolation of the crystalline enzyme from yeast. J Biol Chem. 1961 May;236:1225–1230. [PubMed] [Google Scholar]
- PINCHOT G. B. A rapid method for measuring phosphorylation coupled to the oxidation of reduced diphosphopyridine nucleotide. J Biol Chem. 1957 Nov;229(1):11–23. [PubMed] [Google Scholar]
- STADTMAN E. R., NOVELLI G. D., LIPMANN F. Coenzyme A function in and acetyl transfer by the phosphotransacetylase system. J Biol Chem. 1951 Jul;191(1):365–376. [PubMed] [Google Scholar]
- Smalley A. J., Jahrling P., Van Demark P. J. Molar growth yields as evidence for oxidative phosphorylation in Streptococcus faecalis strain 10Cl. J Bacteriol. 1968 Nov;96(5):1595–1600. doi: 10.1128/jb.96.5.1595-1600.1968. [DOI] [PMC free article] [PubMed] [Google Scholar]
- Vallejos R. H., van den Bergh S. G., Slater E. C. On coupling factors of oxidative phosphorylation. Biochim Biophys Acta. 1968 Apr 2;153(3):509–520. doi: 10.1016/0005-2728(68)90181-3. [DOI] [PubMed] [Google Scholar]