Abstract
Acid titrations of intact and butanol-treated cells of Streptococcus faecalis revealed that nearly all of the intracellular K+ ions could diffuse into the suspending medium in association with small anions, including ribonucleic acid breakdown products, when the cell membrane was damaged. In contrast, nearly all of the intracellular Mg2+ ions appeared to be firmly bound to stable internal cell components but could be displaced reversibly by hydronium ions. The cell membrane acted as a barrier to ion movements, and Mg2+ displacement from intact cells required more acid conditions, by as much as 2.5 pH units, than did displacement from butanol-damaged cells. Some 15 to 20% of the cell magnesium appeared to be associated with surface structures in that it could be removed at pH 7 with ethylenediaminetetraacetic acid or displaced by Co2+, Ni2+, Sr2+, or La3+. Magnesium could be displaced from isolated cell walls and membranes by hydronium ions in the pH range from 5 to 3, over which carboxyl groups were titrated. Displacement of magnesium from ribosomes also took place between pH 5 and 3, but it was more difficult to identify the magnesium-releasing groups because both protein carboxyl groups and purine and pyrimidine ring nitrogens can become protonated in this pH range. Isolated protoplast membranes remained structurally intact when completely depleted of magnesium. Furthermore, protoplasts isolated from intact cells were found to have greatly enhanced resistance to osmotic shock in acid media, even when solute loss was not extensive. Osmotic resistance was lost when the protoplasts were again placed in neutral media, and this reversibility suggested that acidification caused changes in the physical properties of membranes as well as solute leakage from cells.
Full text
PDF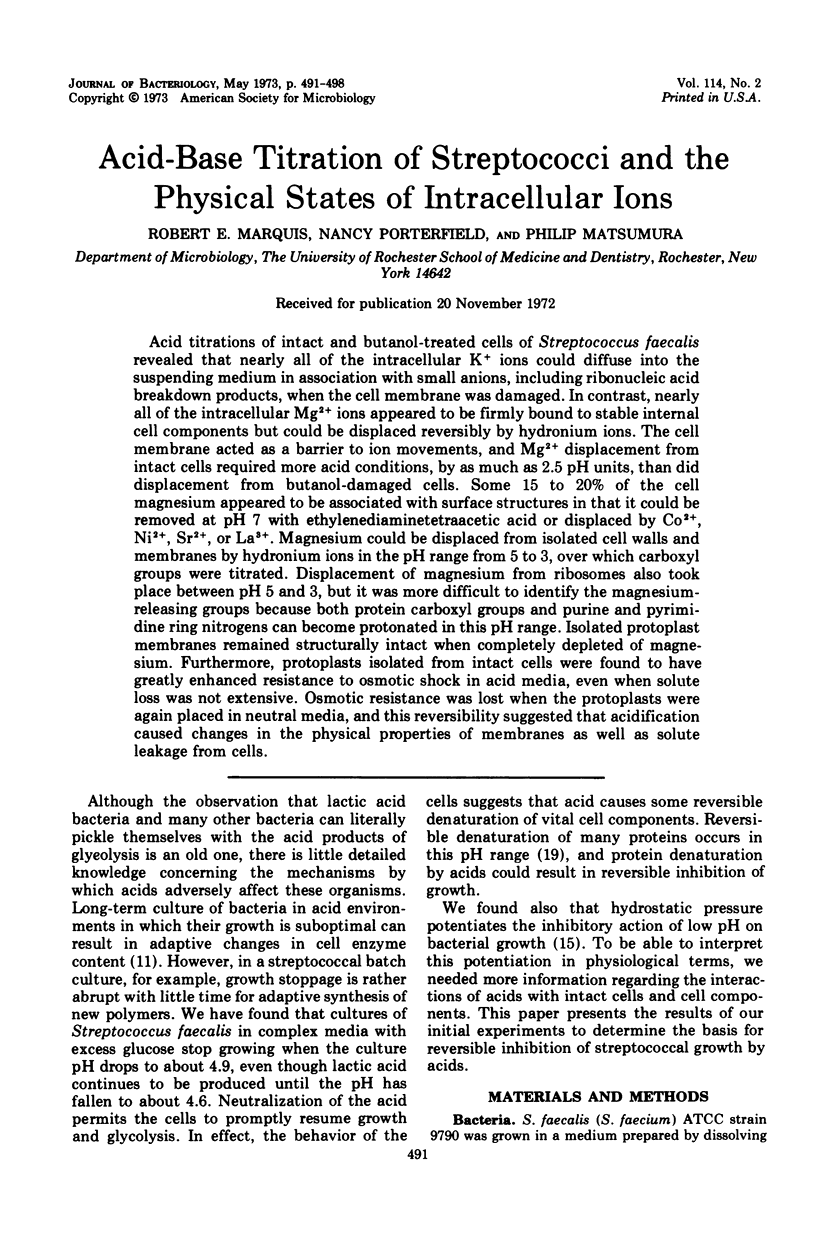
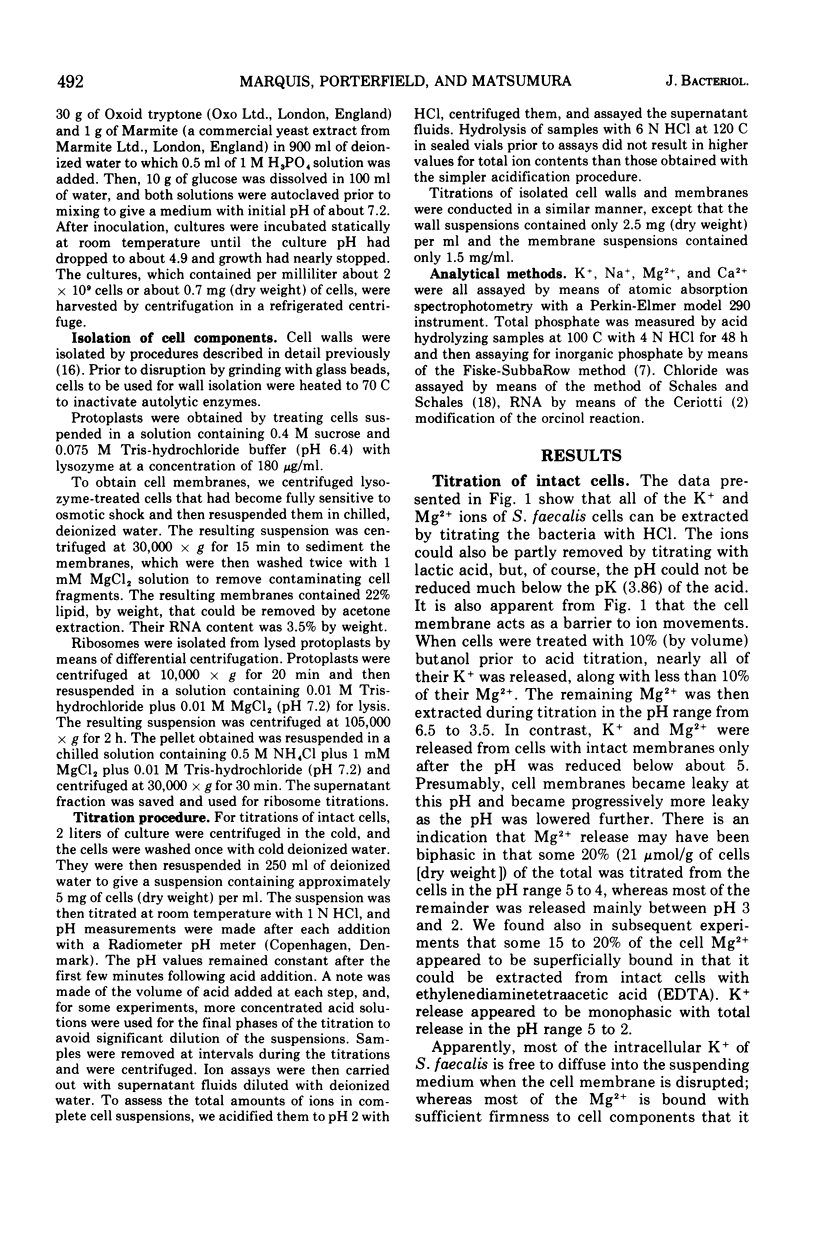
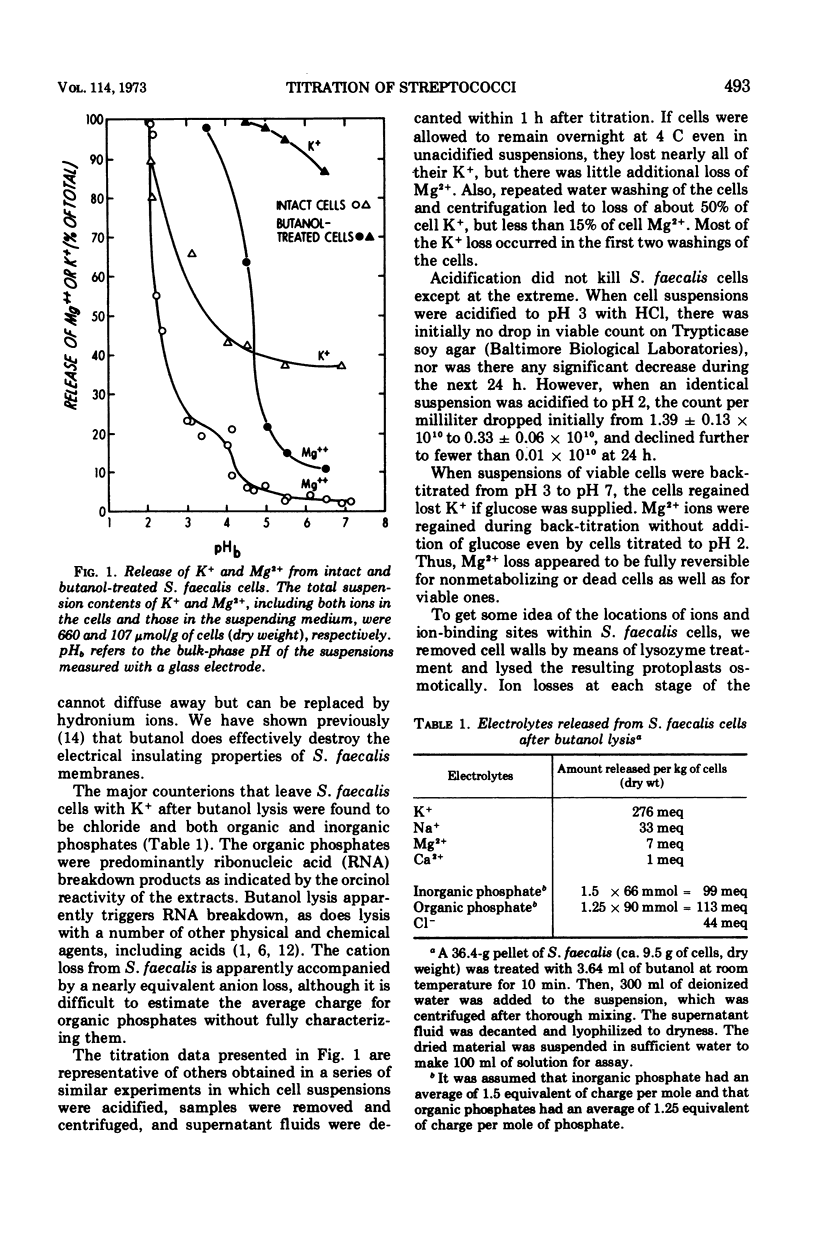
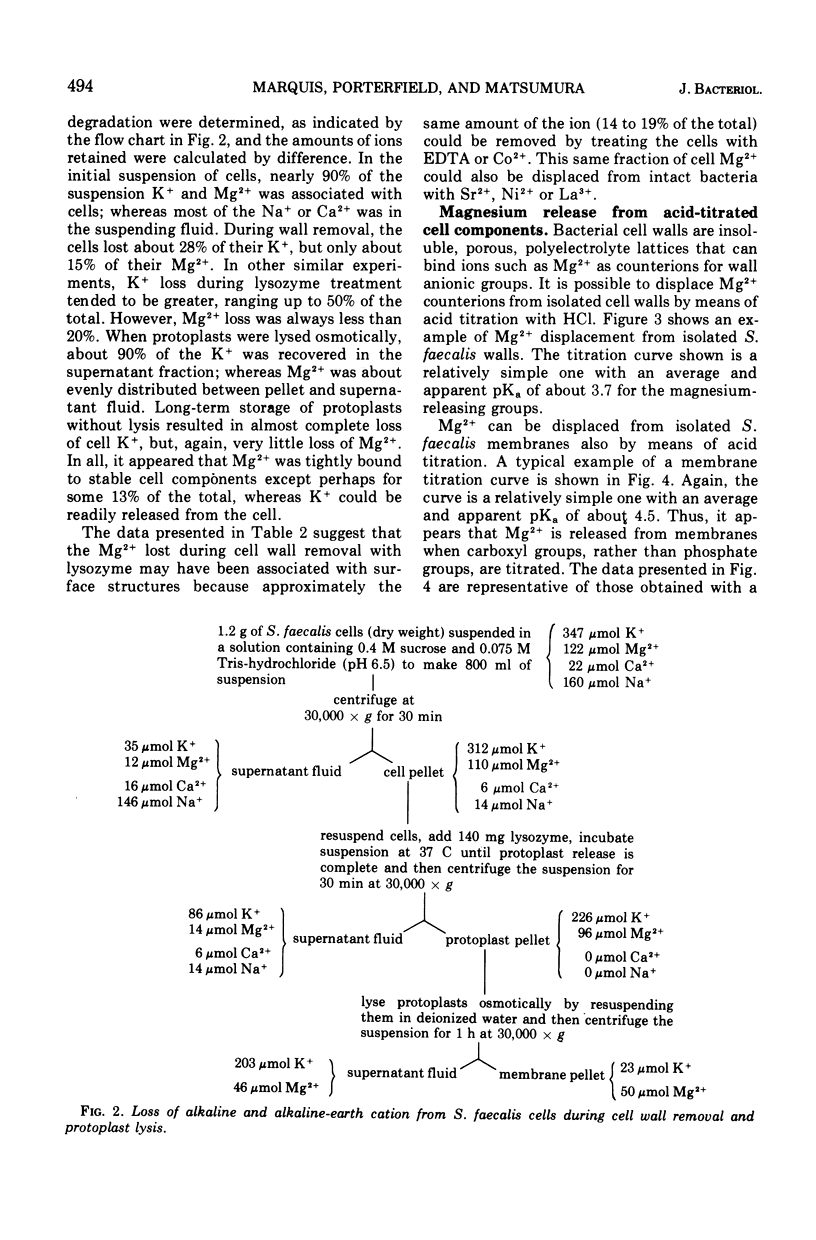
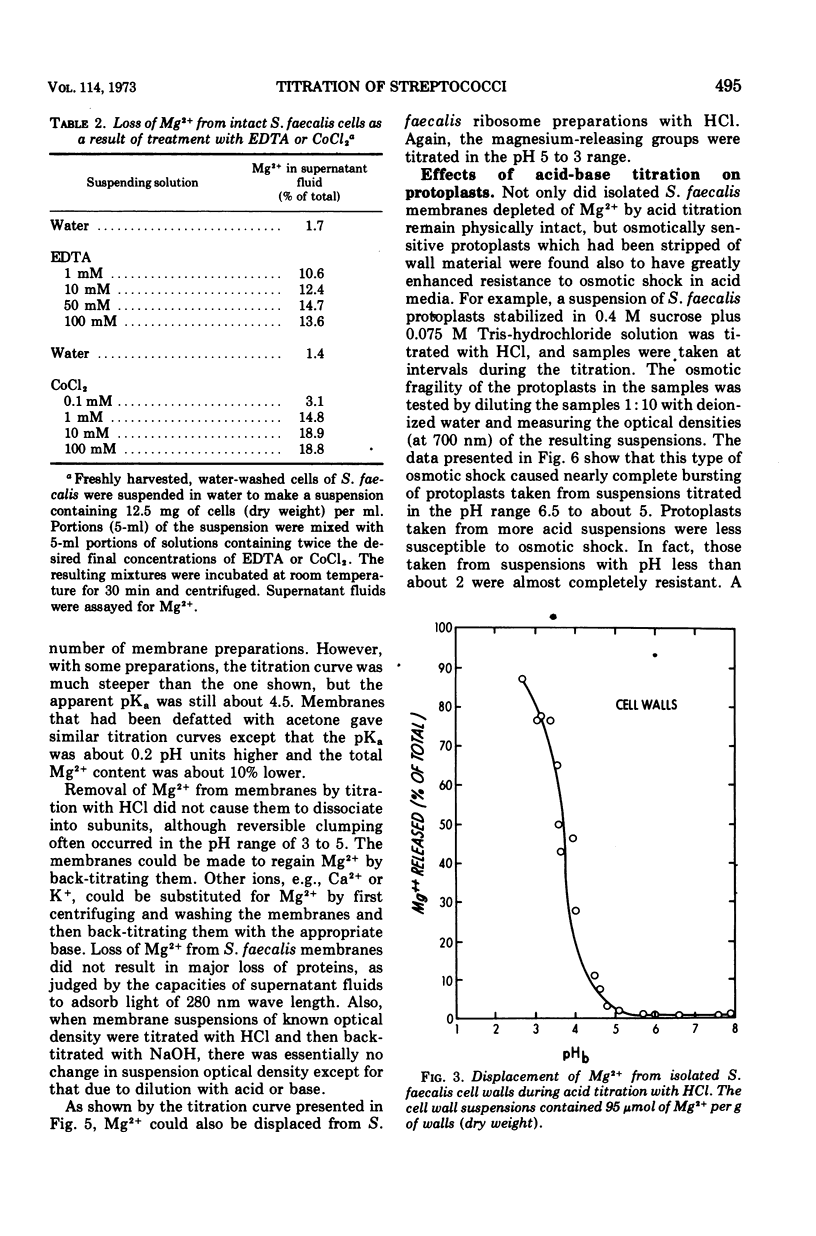
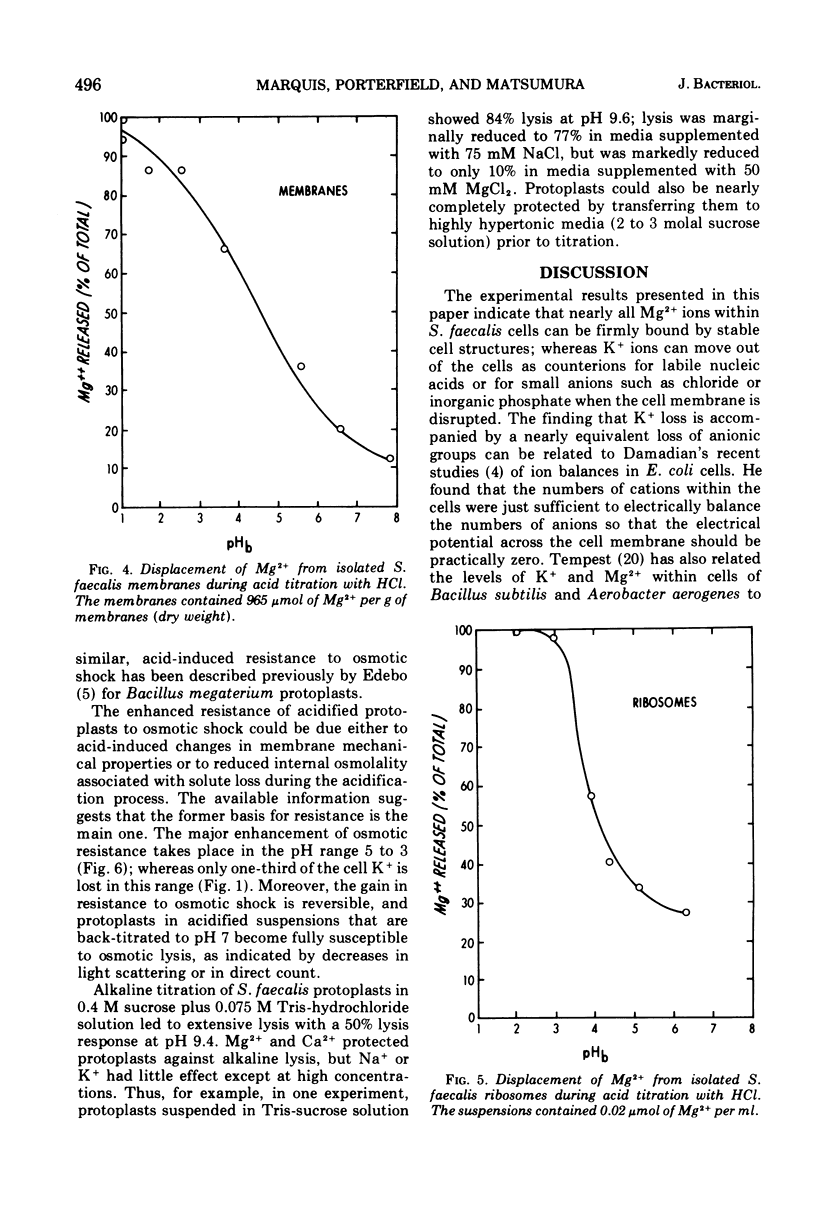
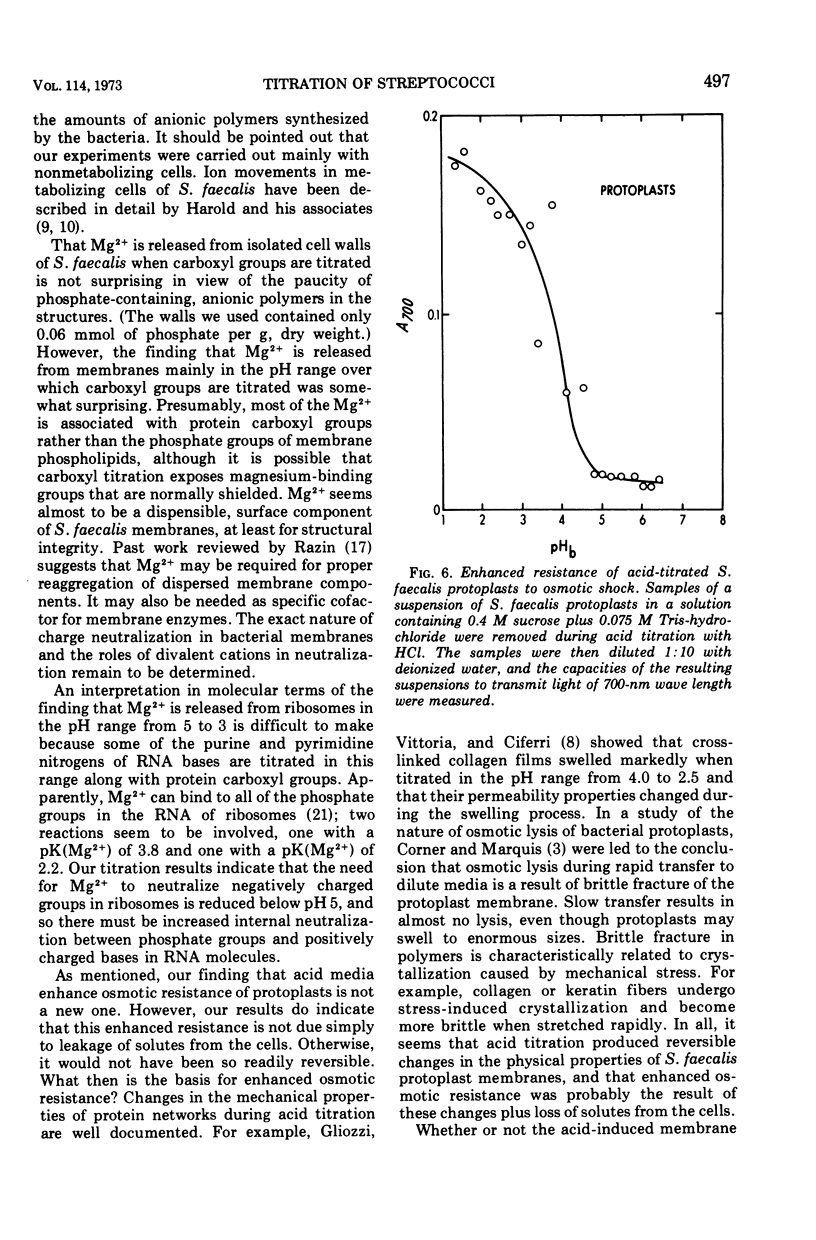
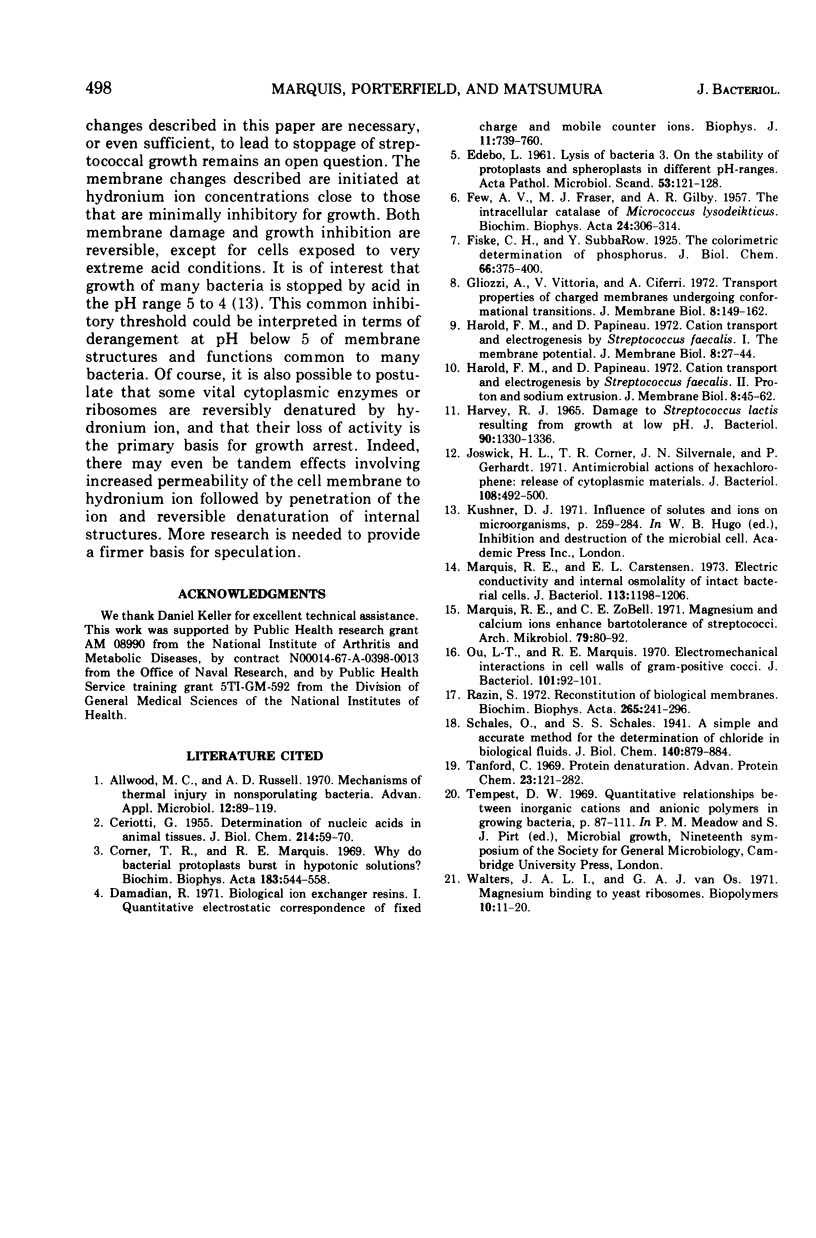
Selected References
These references are in PubMed. This may not be the complete list of references from this article.
- Allwood M. C., Russell A. D. Mechanisms of thermal injury in nonsporulating bacteria. Adv Appl Microbiol. 1970;12:89–119. doi: 10.1016/s0065-2164(08)70583-5. [DOI] [PubMed] [Google Scholar]
- CERIOTTI G. Determination of nucleic acids in animal tissues. J Biol Chem. 1955 May;214(1):59–70. [PubMed] [Google Scholar]
- Corner T. R., Marquis R. E. Why do bacterial protoplasts burst in hypotonic solutions? Biochim Biophys Acta. 1969;183(3):544–558. doi: 10.1016/0005-2736(69)90168-0. [DOI] [PubMed] [Google Scholar]
- Damadian R. Biological ion exchanger resins. I. Quantitative electrostatic correspondence of fixed charge and mobile counter ion. Biophys J. 1971 Sep;11(9):739–760. doi: 10.1016/S0006-3495(71)86251-3. [DOI] [PMC free article] [PubMed] [Google Scholar]
- EDEBO L. Lysis of bacteria. 3. On the stability of protoplasts and spheroplasts in different pH-ranges. Acta Pathol Microbiol Scand. 1961;53:121–128. [PubMed] [Google Scholar]
- FEW A. V., FRASER M. J., GILBY A. R. The intracellular catalase of Micrococcus lysodeikticus. Biochim Biophys Acta. 1957 May;24(2):306–314. doi: 10.1016/0006-3002(57)90199-3. [DOI] [PubMed] [Google Scholar]
- Gliozzi A., Vittoria V., Ciferri A. Transport properties of charged membranes undergoing conformational transitions. J Membr Biol. 1972;8(2):149–162. doi: 10.1007/BF01868099. [DOI] [PubMed] [Google Scholar]
- Harold F. M., Papineau D. Cation transport and electrogenesis by Streptococcus faecalis. I. The membrane potential. J Membr Biol. 1972;8(1):27–44. doi: 10.1007/BF01868093. [DOI] [PubMed] [Google Scholar]
- Harold F. M., Papineau D. Cation transport and electrogenesis by Streptococcus faecalis. II. Proton and sodium extrusion. J Membr Biol. 1972;8(1):45–62. doi: 10.1007/BF01868094. [DOI] [PubMed] [Google Scholar]
- Harvey R. J. Damage to Streptococcus lactis resulting from growth at low pH. J Bacteriol. 1965 Nov;90(5):1330–1336. doi: 10.1128/jb.90.5.1330-1336.1965. [DOI] [PMC free article] [PubMed] [Google Scholar]
- Joswick H. L., Corner T. R., Silvernale J. N., Gerhardt P. Antimicrobial actions of hexachlorophene: release of cytoplasmic materials. J Bacteriol. 1971 Oct;108(1):492–500. doi: 10.1128/jb.108.1.492-500.1971. [DOI] [PMC free article] [PubMed] [Google Scholar]
- Marquis R. E., Carstensen E. L. Electric conductivity and internal osmolality of intact bacterial cells. J Bacteriol. 1973 Mar;113(3):1198–1206. doi: 10.1128/jb.113.3.1198-1206.1973. [DOI] [PMC free article] [PubMed] [Google Scholar]
- Marquis R. E., ZoBell E. Magnesium and calcium ions enhance barotolerance of Streptococci. Arch Mikrobiol. 1971;79(1):80–92. doi: 10.1007/BF00412043. [DOI] [PubMed] [Google Scholar]
- Ou L. T., Marquis R. E. Electromechanical interactions in cell walls of gram-positive cocci. J Bacteriol. 1970 Jan;101(1):92–101. doi: 10.1128/jb.101.1.92-101.1970. [DOI] [PMC free article] [PubMed] [Google Scholar]
- Razin S. Reconstruction of biological membranes. Biochim Biophys Acta. 1972 Apr 18;265(2):241–296. [PubMed] [Google Scholar]
- Tanford C. Protein denaturation. Adv Protein Chem. 1968;23:121–282. doi: 10.1016/s0065-3233(08)60401-5. [DOI] [PubMed] [Google Scholar]
- Walters J. A., van Os G. A. Magnesium binding to yeast ribosomes. Biopolymers. 1971;10(1):11–20. doi: 10.1002/bip.360100103. [DOI] [PubMed] [Google Scholar]