Abstract
Extracts prepared from a halophilic bacterium contained a reduced nicotinamide adenine dinucleotide (NADH2) oxidase active at high solute concentrations. The cation requirement was nonspecific, since KCl, RbCl, and CsCl replaced NaCl with little or no loss of activity, and NH4Cl was only partially effective. Only LiCl failed to replace NaCl. No specific chloride requirement was observed although not all anions replaced chloride. Bromide, nitrate, and iodide were essentially ineffective, whereas acetate, formate, citrate, and sulfate proved suitable. The presence of sulfate affected the ability of a cation to satisfy the solute requirement. Sulfate enhanced the rate of NADH2 oxidation when compared with the rate observed in the presence of chloride. Cations which were inactive as chlorides (LiCl and MgCl2 at high concentrations) satisfied the cation requirement when added as sulfate salts. Although magnesium satisfied the cation requirement, a concentration effect, as well as an anion effect, was observed. In the presence of MgCl2, little NADH2 oxidation was observed at concentrations greater than 1 m. At lower concentrations, the rate of oxidation increased, reaching a maximal value at 0.1 m and remaining constant up to a concentration of 0.05 m MgCl2. Magnesium acetate and MgSO4 also replaced NaCl, and the maximal rate of oxidation occurred at 0.05 m with respect to magnesium. There was no change in the rate of oxidation at high magnesium acetate concentrations, whereas the rate of NADH2 oxidation increased at higher concentrations of MgSO4.
Full text
PDF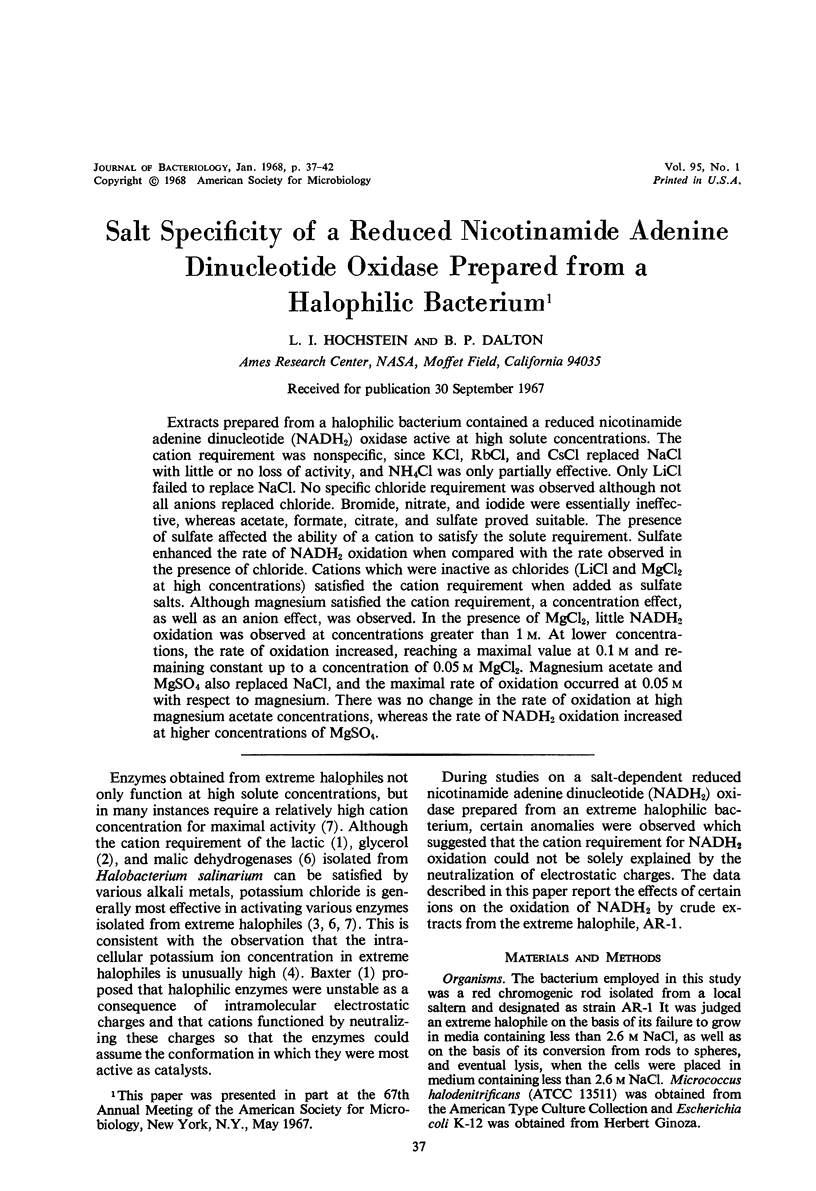
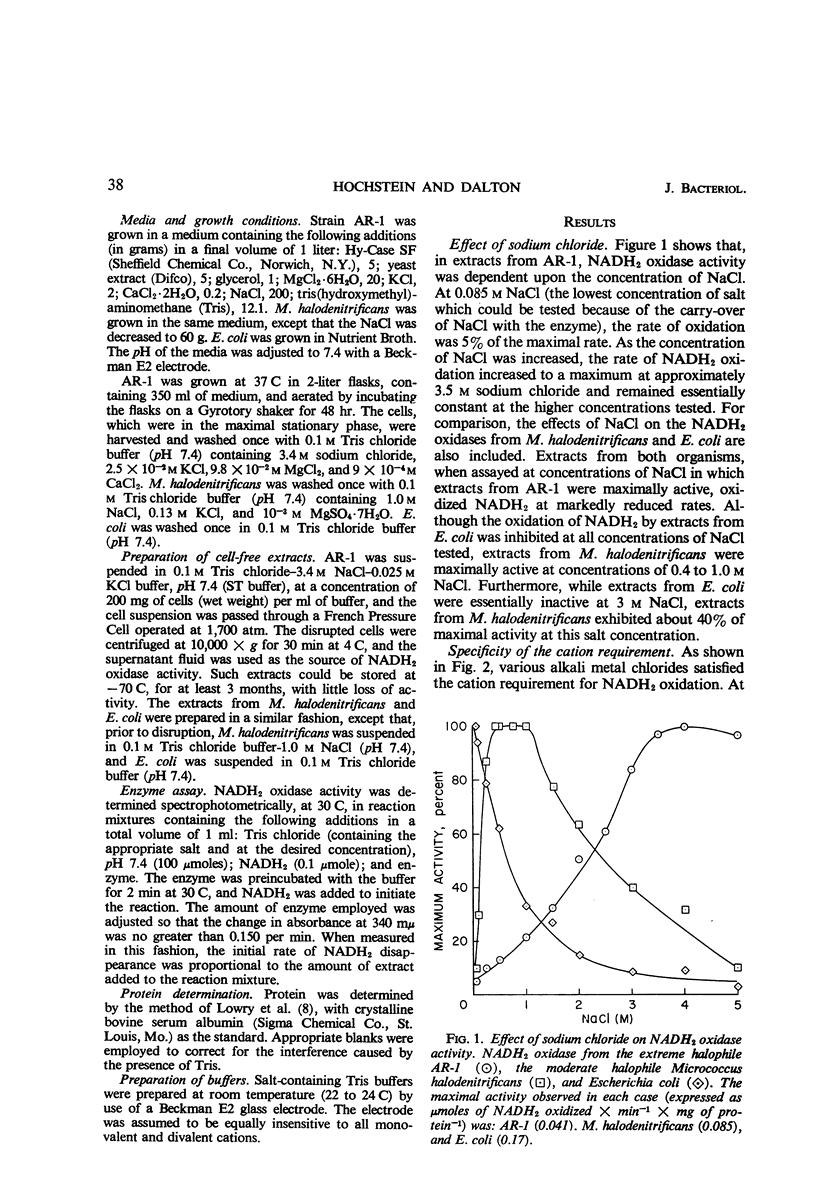
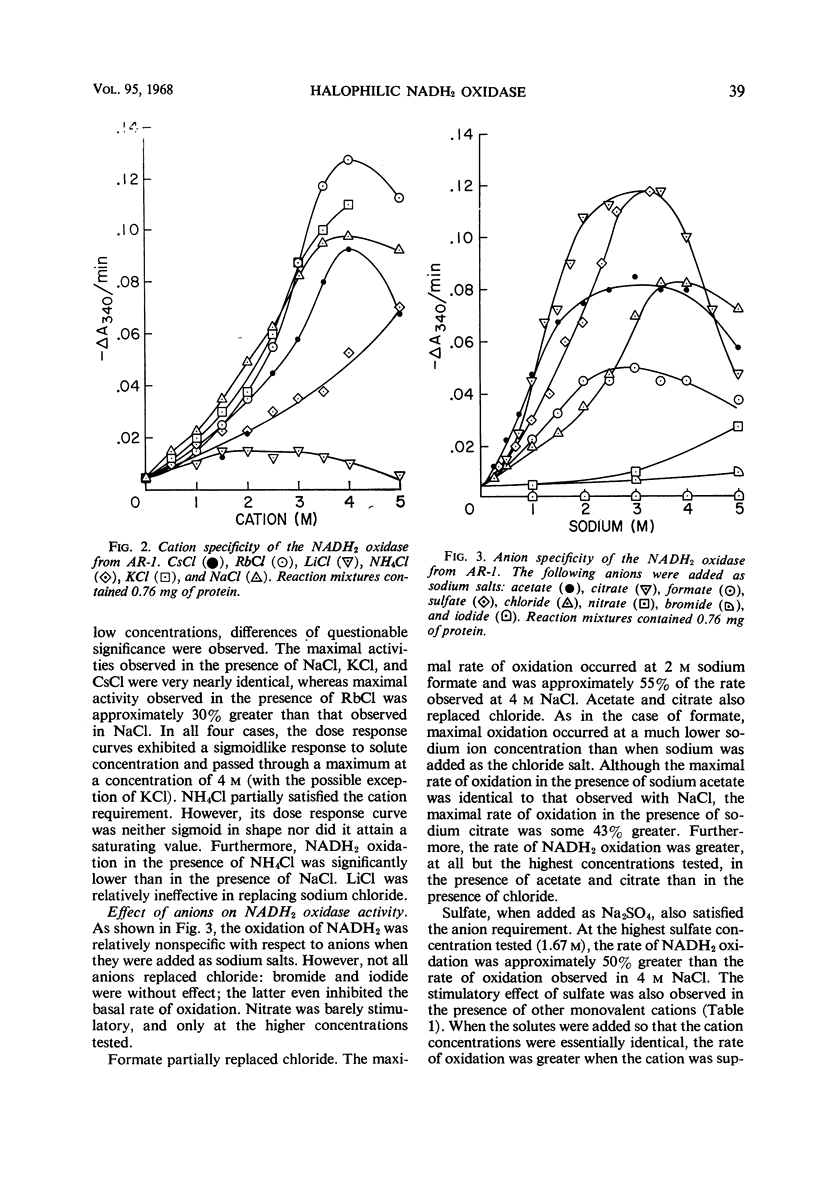
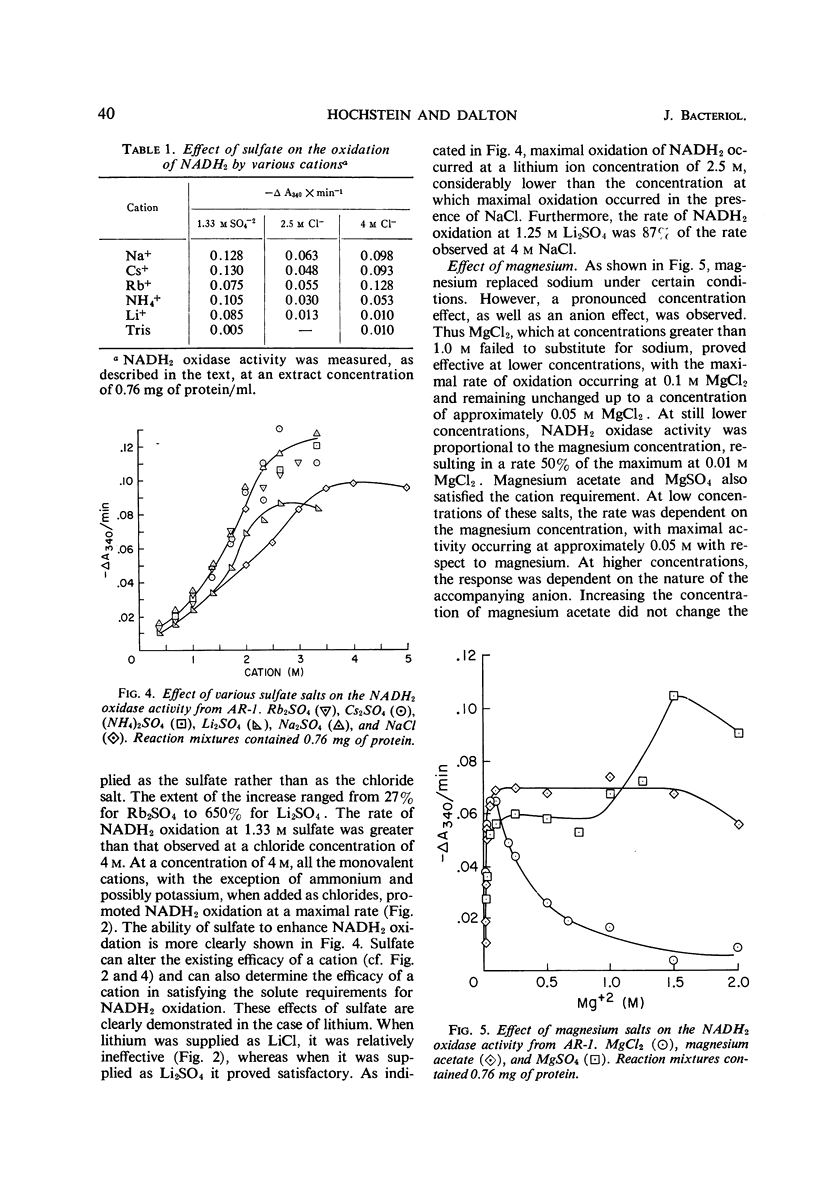
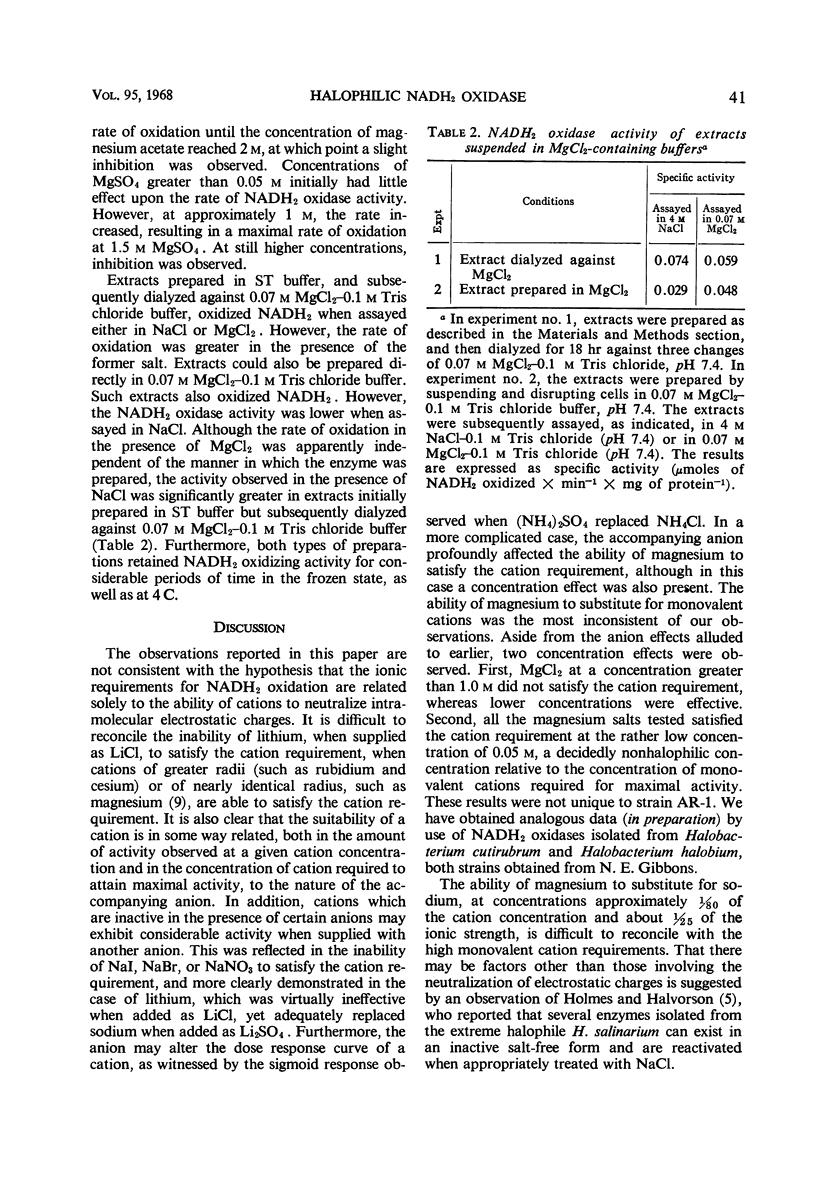
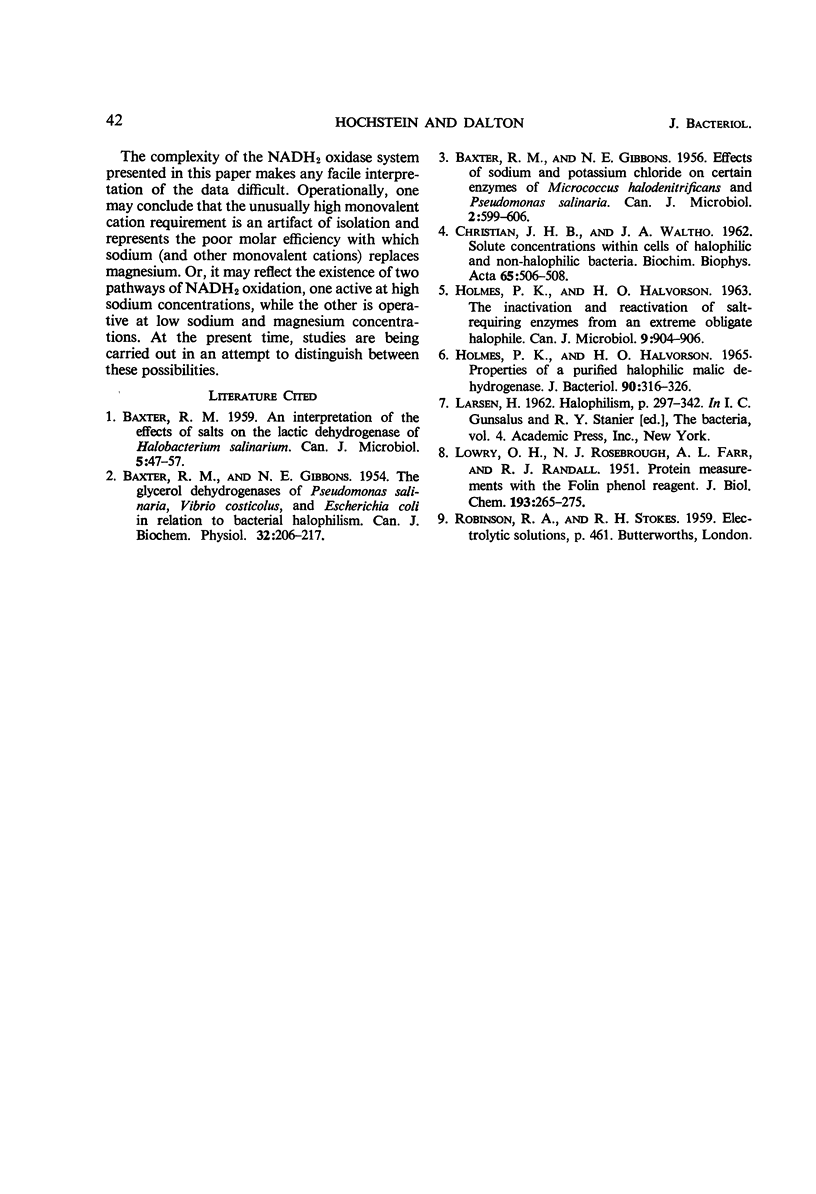
Selected References
These references are in PubMed. This may not be the complete list of references from this article.
- BAXTER R. M. An interpretation of the effects of salts on the lactic dehydrogenase of Halobacterium salinarium. Can J Microbiol. 1959 Feb;5(1):47–57. doi: 10.1139/m59-006. [DOI] [PubMed] [Google Scholar]
- BAXTER R. M., GIBBONS N. E. Effects of sodium and potassium chloride on certain enzymes of Micrococcus halodenitrificans and Pseudomonas salinaria. Can J Microbiol. 1956 Oct;2(6):599–606. doi: 10.1139/m56-072. [DOI] [PubMed] [Google Scholar]
- BAXTER R. M., GIBBONS N. E. The glycerol dehydrogenases of Pseudomonas salinaria, Vibrio costicolus, and Escherichia coli in relation to bacterial halophilism. Can J Biochem Physiol. 1954 May;32(3):206–217. [PubMed] [Google Scholar]
- CHRISTIAN J. H., WALTHO J. A. Solute concentrations within cells of halophilic and non-halophilic bacteria. Biochim Biophys Acta. 1962 Dec 17;65:506–508. doi: 10.1016/0006-3002(62)90453-5. [DOI] [PubMed] [Google Scholar]
- HOLMES P. K., HALVORSON H. O. PROPERTIES OF A PURIFIED HALOPHILIC MALIC DEHYDROGENASE. J Bacteriol. 1965 Aug;90:316–326. doi: 10.1128/jb.90.2.316-326.1965. [DOI] [PMC free article] [PubMed] [Google Scholar]
- LOWRY O. H., ROSEBROUGH N. J., FARR A. L., RANDALL R. J. Protein measurement with the Folin phenol reagent. J Biol Chem. 1951 Nov;193(1):265–275. [PubMed] [Google Scholar]